Hepatitis B: progress in understanding chronicity, the innate immune response, and cccDNA protection
Introduction
Hepatitis B virus (HBV) was initially identified as the novel “Australia antigen” in the serum of some healthy individuals by Blumberg et al. in 1965 (1). In the decades since this report, hepatitis B has been established as an infectious disease caused by HBV. HBV infection can lead to acute hepatitis, chronic hepatitis, liver cirrhosis, and hepatocellular carcinoma (HCC). Even though an effective hepatitis B vaccine is available, the number of people worldwide with a history of HBV infection is estimated to reach 2 billion, while the number of currently infected carriers may reach 240 million (2,3). Global mortality due to HBV-related liver diseases ranges from 500,000 to 1 million deaths per year. Thus, despite vaccine availability, HBV infection remains a serious public health problem worldwide.
HBV is a DNA virus belonging to the Hepadnaviridae family. The HBV genome is approximately 3,200 bp in length and is contained in circular partially double-stranded DNA. The genome contains four partially-overlapped regions: the pre-S/S domain, which encodes envelope proteins; the pre-C/C domain, which encodes core protein and hepatitis B e antigen (HBeAg); the P domain, which encodes viral polymerase; and the X domain, which encodes HBV X protein (HBx).
To date, ten different genotypes of HBV (designated A to J) have been identified, with each genotype exhibiting a variety of clinical presentations (4,5). Genotypes B and C are the dominant HBV genotypes in East Asia, where the rate of chronicity in adulthood HBV infection is estimated at less than 1%. Recently, HBV genotype Ae has been transmitted sexually across the world from its origin in western countries; this genotype has a higher chronicity rate of approximately 5–10% (6,7).
HBV establishes a chronic infection by evading the host immune system. Because HBV itself lacks an efficient level of cytotoxicity, it is the host immune response to virus-infected hepatocytes that leads to the development of hepatitis B. HBV-specific CD8+ T cells significantly contribute to both HBV elimination and hepatitis development. The innate immune response during the initial phase of HBV infection is essential for the induction of a sufficient level of acquired immunity against the virus. However, the innate immune reaction to HBV infection—including the specific roles of immunocompetent cells and associated molecules—remains to be fully elucidated.
Recently, de novo HBV reactivation during chemotherapy and immunosuppressive therapy has emerged as a major concern. Therefore, the clearance of hepatitis B surface antigen (HBsAg) has been established as the long-term goal of anti-HBV treatment. HBsAg seroclearance is commonly understood as a functional cure of HBV infection, even when covalently closed circular DNA (cccDNA) remains. Currently available treatment options like pegylated interferon (Peg-IFN) and nucleos(t)ide reverse transcriptase inhibitors (NRTIs) are insufficient to completely cure HBV and eliminate cccDNA. Thus, the complete elimination of cccDNA remains elusive, given that the mechanisms by which cccDNA is protected has not yet been completely determined.
In this review, we examine the current understanding of the mechanisms behind hepatitis development by HBV, with a particular focus on immune response. We also consider the mechanisms underlying protection of cccDNA, which is considered to be a vital strategy behind HBV persistence.
Persistent infection with HBV
There is a marked difference in clinical course depending on the age when HBV infection occurs. More than 90% of infants infected at birth by HBV-infected mothers suffer from persistent HBV infection. By contrast, more than 95% of adults infected with HBV during adulthood—primarily through sexual transmission—will eliminate HBV naturally. Among immunocompetent individuals infected with HBV in adulthood, the majority will have no apparent infection, while approximately 20–30% will initially develop acute hepatitis. In 95% of cases, detection of HBsAg will resolve within 6 months of infection, although superinfection with human immunodeficiency virus (HIV) and HBV genotype Ae infection has been associated with a higher chronicity rate.
The age-dependent disparity in clinical course can be attributed to the immature immune system in infancy. Several studies have demonstrated that the maturation of Kupffer cell plays an important role in the induction of anti-HBV immune response during the transition between the immune activation phase and the immune tolerance phase (8,9). However, the triggering mechanism behind this adolescent change in immunological response remains poorly understood. It was reported that an impaired production of IL-10, a change in Th1-dominated T cell response, and an interaction with effector T cell were observed in the transition from the immune tolerance to the immune activation phase.
In cases where HBV persists after initial infection in adulthood, the increased expression of inhibitory receptors like programmed cell death 1 (PD-1) has been observed on HBV-specific CD8+ T cells and regulatory T cells. Indeed, persistent infection with HBV may be maintained by the induction of hepatic immune tolerance by increased production of IL-10 from Kupffer cells, the diminished production of IFN-α from dendritic cells (DCs), the impaired production of IFN-γ from natural killer (NK) cells, and the apoptosis of HBV-specific cytotoxic T lymphocyte (CTL) by activated NK cells (10-12).
Individuals with chronic hepatitis B infection are at risk of hepatic flare; however, the mechanisms behind this abrupt rise in alanine aminotransferase (ALT) levels remain to be determined. In the acute exacerbation phase, an increase in HBV DNA and immunosuppressive components like IL-10, regulatory B cells, and regulatory T cells was observed prior to the peak in ALT levels. However, the impetus behind hepatic flare and its associated molecules are still unclear (13).
HBV infection is considered resolved when an individual is negative for HBsAg, positive for hepatitis B core antibody (HBcAb), and/or positive for hepatitis B surface antibody (HBsAb). Even in resolved cases, a low level of cccDNA persists in the liver and peripheral blood mononuclear cells (14,15). Thus, HBV can reactivate during immunosuppressive treatment or cytotoxic chemotherapy (16-20). Presumably, the reduction in immune functions which controlled HBV replication in turn triggers HBV reactivation. Identifying the variables behind HBV reactivation is an active area of research, with many groups investigating the association between reactivation and patient characteristics, genetics, virological features, underlying disease, and treatment type.
Innate immunity against HBV
Following initial infection, HBV DNA is detectable within 4–7 weeks, viral replication peaks at 8–10 weeks, and liver injury develops after 12 weeks (21,22). Individuals with an exceptionally overactive immune response against the rapid growth of HBV mutants develop fulminant hepatitis. In contrast with hepatitis C infection, studies of HBV-infected chimpanzees (23) showed that HBV does not induce interferon-stimulated genes (ISGs) during the viral incubation phase. These results indicate that HBV potentially evades the host innate immune response by reducing its visibility to immunological surveillance mechanisms. Given that HBV replication is generally not detectable until about 4 weeks after HBV infection, innate immunity is predicted to be important for controlling virus replication.
The viral life cycle of HBV offers limited opportunities for host cells to sense HBV infection. After an HBV particle enters into a host cell, it releases the HBV genome-containing nucleocapsid into the cytoplasm (Figure 1). The core particle protects the HBV genome as it moves through the cytoplasm to the nucleus. During HBV particle formation, viral pregenomic RNA (pgRNA) has a 5’ ε stem-loop element. Polymerase binding to the ε stem-loop triggers capsid formation, and the pgRNA is selectively encapsidated with the polymerase within core particles in the cytoplasm. Nonetheless, Sato et al. reported that hepatocytes recognize the 5’ ε stem-loop of HBV pgRNA via retinoic acid-inducible gene I (RIG-I), and that RIG-I exerts an anti-HBV effect by blocking the docking between HBV polymerase and pgRNA (24).
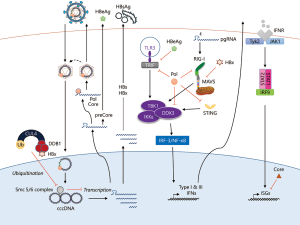
However, HBV possesses numerous additional strategies to counteract host innate immune responses. It was reported that HBV polymerase inhibits the innate immune response’s RNA- and DNA-sensing pathways by preventing the activation of interferon regulatory factor 3 (IRF-3) (25,26) and by interfering with stimulator of interferon genes (STING)-mediated IFN-β induction (27). Furthermore, HBx has been reported to negatively regulate innate immunity by targeting mitochondrial antiviral signaling protein (MAVS) (28-30) and TIR-domain-containing adapter-inducing interferon-β (TRIF) (31). Because of the lack of novel cell culture model systems, it is not able to produce infectious HBV particles of adequate quantity and quality for researching innate immunity against HBV. In the near future, robust HBV cell culture model will allow us to investigate the sensor molecules of HBV and host defense system against HBV infection in more detail.
Studies in acute hepatitis patients showed that NK and natural killer T (NKT) cell populations increased prior to the peak of HBV DNA replication, and then decreased with the concomitant reduction in HBV DNA (32). According to a detailed case analysis of the immune reaction in patients who naturally eliminated HBV infection without ALT elevation, both activated NK cells and increased numbers of HBV-specific CD4+ and CD8+ T cells were observed subsequent to the enhancement of NK cell-derived IFN-γ secretion (33). These results indicate that the activation of innate immunity is of considerable importance to natural elimination of HBV, and that innate immune activation precedes the development of acquired immunity in HBV infection. Moreover, non-cytopathic mechanisms were observed to be effective in both a mouse model (22) and humans, with IFN-γ playing a significant role in the inhibition of HBV replication. In support of this, IFN-γ was reported to be produced by CD8+ T cells, NK cells, and NKT cells during the early phase of initial infection, and that this correlated with a decrease in intrahepatic cccDNA (33).
Acquired immunity against HBV
In acute hepatitis B, HBV-specific CD8+ T cells enter the liver and produce IFN-γ (34). Chimpanzee studies have shown that CD8+ T cells are critical to eliminating HBV; when depleted during HBV amplification, infected animals lost the capacity to eradicate HBV. In contrast, CD4+ T cell depletion in acute hepatitis B has little effect on HBV eradication. However, CD4+ T cell depletion before HBV infection impairs HBV eradication by decreasing CD8+ T cell migration into the liver (35). These results indicate that the early priming of CD4+ T cell by HBV is of considerable importance to eradicating HBV.
In addition to CD4+ and CD8+ T cells, the role of HBV-specific CTLs has been examined in studies where these cells were transferred to HBsAg transgenic mouse models. During the onset of hepatitis, hepatocyte express chemokine-like CXCL9 and CXCL10, thereby prompting HBV-specific CTLs to migrate and infiltrate into hepatocytes. These CTLs subsequently damage hepatocytes through a cytopathic mechanism (apoptosis). Neutrophils and platelets have also been reported to participate in hepatic injury (36). However, CTLs have also shown anti-HBV activity through non-cytopathic mechanisms (e.g., IFN-γ and TNF-α production). In a mouse model, DC activation was observed during the early phase of HBV infection, and these activated DCs were associated with anti-HBV activity. However, the role of DCs in human acute hepatitis B is not clear. During the recovery phase, sufficient HBsAb are produced to neutralize circulating HBsAg, thereby contributing to HBV elimination.
Protection of cccDNA by manipulation of host systems
HBV cccDNA is vital to the persistence of HBV. Unsurprisingly, many cccDNA-targeting drug candidates are undergoing evaluation in preclinical and/or clinical trials. Of these drugs, disubstituted sulfonamide compounds were identified as specific inhibitors of cccDNA formation, and were shown to interfere with the conversion of relaxed circular DNA to cccDNA (37). Indeed, activation of the lymphotoxin-β receptor was reported to alter the specific degradation of nuclear cccDNA by a cytidine-deamination mechanism via APOBEC3B upregulation (38), which resembles activation of APOBEC3A by IFN-α.
Recently, observations on HBV’s protection of cccDNA were reported by Decorsière et al. (39). HBx protein was reported to interact with damaged DNA binding protein 1 (DDB1), which is a component of the cullin 4 (CUL4)-DDB1 ubiquitin ligase complex (40,41). In addition to HBV, simian virus 5 (42), human parainfluenza virus 2 (43), mumps virus, HIV-I (44,45), HIV-II (46), murine gammaherpesvirus 68 (47), and Epstein-Barr virus (48) have all been observed to target the ubiquitin ligase activity of the CUL4-DDB1 E3 ligase, in order to counteract the host innate immune response and establish persistent infection. Decorsière et al. found that HBx causes the CUL4-DDB1 E3 ligase to target and degrade the structural maintenance of chromosomes (Smc) 5/6 complex. The Smc 5/6 complex appears to bind to only episomal HBV DNA and thereby inhibit its transcription, but does not appear to bind to chromosomally-integrated HBV DNA. Thus, this report indicated that Smc 5/6 acts as a cellular antiviral factor and that HBx manipulates the host ubiquitin-proteasome system to degrade the Smc 5/6 complex, thereby enabling HBV infection to persist.
Conclusions
In this review, we described the current understanding of the mechanisms behind HBV-induced hepatitis development from the perspective of the host immune response. We also focused on the mechanisms by which HBV protects cccDNA. Further research into these mechanisms is essential to develop treatments that will eliminate cccDNA from HBV carriers, and to ultimately eradicate HBV worldwide.
Acknowledgements
The authors gratefully acknowledge Koji Ogawa, Goki Suda, Takuya Sho, Masato Nakai, Jyun Ito, Naoki Kawagishi, and Masatsugu Ohara of the Hepatology Department of the Hokkaido University Hospital, and Hatsumi Ueda, Terumi Hatakeyama, and Megumi Kimura for technical support; Ayumi Kachi, Maki Makino, Masumi Kodama, Kazue Yosida, and Keiko Sano for administrative support; and Masaya Sugiyama, Tomoko Date, and Takaji Wakita for helpful discussions.
Funding was provided by the Japan Agency for Medical Research and Development; the Ministry of Health, Labor and Welfare, Japan; the Ministry of Education, Culture, Sports, Science and Technology, Japan; and the Viral Hepatitis Research Foundation of Japan.
Footnote
Conflicts of Interest: The authors have no conflicts of interest to declare.
References
- Blumberg BS, Alter HJ, Visnich S. A. "new" antigen in leukemia sera. JAMA 1965;191:541-6. [Crossref] [PubMed]
- World Health Organization. Hepatits B. Media Centre, Fact sheet Updated June 2014: No. 204. Available online: http://www.who.int/mediacentre/factsheets/fs204/en/
- Ott JJ, Stevens GA, Groeger J, et al. Global epidemiology of hepatitis B virus infection: new estimates of age-specific HBsAg seroprevalence and endemicity. Vaccine 2012;30:2212-9. [Crossref] [PubMed]
- Kramvis A, Kew M, François G. Hepatitis B virus genotypes. Vaccine 2005;23:2409-23. [Crossref] [PubMed]
- Miyakawa Y, Mizokami M. Classifying hepatitis B virus genotypes. Intervirology 2003;46:329-38. [Crossref] [PubMed]
- Ito K, Yotsuyanagi H, Sugiyama M, et al. Geographic distribution and characteristics of genotype A hepatitis B virus infection in acute and chronic hepatitis B patients in Japan. J Gastroenterol Hepatol 2016;31:180-9. [Crossref] [PubMed]
- Matsuura K, Tanaka Y, Hige S, et al. Distribution of hepatitis B virus genotypes among patients with chronic infection in Japan shifting toward an increase of genotype A. J Clin Microbiol 2009;47:1476-83. [Crossref] [PubMed]
- Chou HH, Chien WH, Wu LL, et al. Age-related immune clearance of hepatitis B virus infection requires the establishment of gut microbiota. Proc Natl Acad Sci U S A 2015;112:2175-80. [Crossref] [PubMed]
- Publicover J, Gaggar A, Nishimura S, et al. Age-dependent hepatic lymphoid organization directs successful immunity to hepatitis B. J Clin Invest 2013;123:3728-39. [Crossref] [PubMed]
- Peppa D, Gill US, Reynolds G, et al. Up-regulation of a death receptor renders antiviral T cells susceptible to NK cell-mediated deletion. J Exp Med 2013;210:99-114. [Crossref] [PubMed]
- Xu L, Yin W, Sun R, et al. Kupffer cell-derived IL-10 plays a key role in maintaining humoral immune tolerance in hepatitis B virus-persistent mice. Hepatology 2014;59:443-52. [Crossref] [PubMed]
- Zhang Z, Zhang S, Zou Z, et al. Hypercytolytic activity of hepatic natural killer cells correlates with liver injury in chronic hepatitis B patients. Hepatology 2011;53:73-85. [Crossref] [PubMed]
- Chang ML, Liaw YF. Hepatitis B flares in chronic hepatitis B: pathogenesis, natural course, and management. J Hepatol 2014;61:1407-17. [Crossref] [PubMed]
- Rehermann B, Ferrari C, Pasquinelli C, et al. The hepatitis B virus persists for decades after patients' recovery from acute viral hepatitis despite active maintenance of a cytotoxic T-lymphocyte response. Nat Med 1996;2:1104-8. [Crossref] [PubMed]
- Yotsuyanagi H, Yasuda K, Iino S, et al. Persistent viremia after recovery from self-limited acute hepatitis B. Hepatology 1998;27:1377-82. [Crossref] [PubMed]
- Kusumoto S, Tanaka Y, Suzuki R, et al. Monitoring of Hepatitis B Virus (HBV) DNA and Risk of HBV Reactivation in B-Cell Lymphoma: A Prospective Observational Study. Clin Infect Dis 2015;61:719-29. [Crossref] [PubMed]
- Liu CJ, Chen PJ, Chen DS, et al. Hepatitis B virus reactivation in patients receiving cancer chemotherapy: natural history, pathogenesis, and management. Hepatol Int 2013;7:316-26. [Crossref] [PubMed]
- Takahashi H, Ikeda M, Kumada T, et al. Multicenter cooperative case survey of hepatitis B virus reactivation by chemotherapeutic agents. Hepatol Res 2015;45:1220-7. [Crossref] [PubMed]
- Tanaka E, Umemura T. History and prevention of de novo hepatitis B virus-related hepatitis in Japan and the world. Clin J Gastroenterol 2008;1:83-6. [Crossref] [PubMed]
- Umemura T, Tanaka E, Kiyosawa K, et al. Mortality secondary to fulminant hepatic failure in patients with prior resolution of hepatitis B virus infection in Japan. Clin Infect Dis 2008;47:e52-6. [Crossref] [PubMed]
- Fong TL, Di Bisceglie AM, Biswas R, et al. High levels of viral replication during acute hepatitis B infection predict progression to chronicity. J Med Virol 1994;43:155-8. [Crossref] [PubMed]
- Guidotti LG, Rochford R, Chung J, et al. Viral clearance without destruction of infected cells during acute HBV infection. Science 1999;284:825-9. [Crossref] [PubMed]
- Wieland SF, Spangenberg HC, Thimme R, et al. Expansion and contraction of the hepatitis B virus transcriptional template in infected chimpanzees. Proc Natl Acad Sci U S A 2004;101:2129-34. [Crossref] [PubMed]
- Sato S, Li K, Kameyama T, et al. The RNA sensor RIG-I dually functions as an innate sensor and direct antiviral factor for hepatitis B virus. Immunity 2015;42:123-32. [Crossref] [PubMed]
- Wang H, Ryu WS. Hepatitis B virus polymerase blocks pattern recognition receptor signaling via interaction with DDX3: implications for immune evasion. PLoS Pathog 2010;6:e1000986. [Crossref] [PubMed]
- Yu S, Chen J, Wu M, et al. Hepatitis B virus polymerase inhibits RIG-I- and Toll-like receptor 3-mediated beta interferon induction in human hepatocytes through interference with interferon regulatory factor 3 activation and dampening of the interaction between TBK1/IKKepsilon and DDX3. J Gen Virol 2010;91:2080-90. [Crossref] [PubMed]
- Liu Y, Li J, Chen J, et al. Hepatitis B virus polymerase disrupts K63-linked ubiquitination of STING to block innate cytosolic DNA-sensing pathways. J Virol 2015;89:2287-300. [Crossref] [PubMed]
- Kumar M, Jung SY, Hodgson AJ, et al. Hepatitis B virus regulatory HBx protein binds to adaptor protein IPS-1 and inhibits the activation of beta interferon. J Virol 2011;85:987-95. [Crossref] [PubMed]
- Wang X, Li Y, Mao A, et al. Hepatitis B virus X protein suppresses virus-triggered IRF3 activation and IFN-beta induction by disrupting the VISA-associated complex. Cell Mol Immunol 2010;7:341-8. [Crossref] [PubMed]
- Wei C, Ni C, Song T, et al. The hepatitis B virus X protein disrupts innate immunity by downregulating mitochondrial antiviral signaling protein. J Immunol 2010;185:1158-68. [Crossref] [PubMed]
- Hong Y, Zhou L, Xie H, et al. Innate immune evasion by hepatitis B virus-mediated downregulation of TRIF. Biochem Biophys Res Commun 2015;463:719-25. [Crossref] [PubMed]
- Dunn C, Peppa D, Khanna P, et al. Temporal analysis of early immune responses in patients with acute hepatitis B virus infection. Gastroenterology 2009;137:1289-300. [Crossref] [PubMed]
- Fisicaro P, Valdatta C, Boni C, et al. Early kinetics of innate and adaptive immune responses during hepatitis B virus infection. Gut 2009;58:974-82. [Crossref] [PubMed]
- Thimme R, Wieland S, Steiger C, et al. CD8(+) T cells mediate viral clearance and disease pathogenesis during acute hepatitis B virus infection. J Virol 2003;77:68-76. [Crossref] [PubMed]
- Asabe S, Wieland SF, Chattopadhyay PK, et al. The size of the viral inoculum contributes to the outcome of hepatitis B virus infection. J Virol 2009;83:9652-62. [Crossref] [PubMed]
- Iannacone M, Sitia G, Ruggeri ZM, et al. HBV pathogenesis in animal models: recent advances on the role of platelets. J Hepatol 2007;46:719-26. [Crossref] [PubMed]
- Cai D, Mills C, Yu W, et al. Identification of disubstituted sulfonamide compounds as specific inhibitors of hepatitis B virus covalently closed circular DNA formation. Antimicrob Agents Chemother 2012;56:4277-88. [Crossref] [PubMed]
- Lucifora J, Xia Y, Reisinger F, et al. Specific and nonhepatotoxic degradation of nuclear hepatitis B virus cccDNA. Science 2014;343:1221-8. [Crossref] [PubMed]
- Decorsière A, Mueller H, van Breugel PC, et al. Hepatitis B virus X protein identifies the Smc5/6 complex as a host restriction factor. Nature 2016;531:386-9. [Crossref] [PubMed]
- Becker SA, Lee TH, Butel JS, et al. Hepatitis B virus X protein interferes with cellular DNA repair. J Virol 1998;72:266-72. [PubMed]
- Wentz MJ, Becker SA, Slagle BL. Dissociation of DDB1-binding and transactivation properties of the hepatitis B virus X protein. Virus Res 2000;68:87-92. [Crossref] [PubMed]
- Precious B, Childs K, Fitzpatrick-Swallow V, et al. Simian virus 5 V protein acts as an adaptor, linking DDB1 to STAT2, to facilitate the ubiquitination of STAT1. J Virol 2005;79:13434-41. [Crossref] [PubMed]
- Ulane CM, Horvath CM. Paramyxoviruses SV5 and HPIV2 assemble STAT protein ubiquitin ligase complexes from cellular components. Virology 2002;304:160-6. [Crossref] [PubMed]
- Le Rouzic E, Belaïdouni N, Estrabaud E, et al. HIV1 Vpr arrests the cell cycle by recruiting DCAF1/VprBP, a receptor of the Cul4-DDB1 ubiquitin ligase. Cell Cycle 2007;6:182-8. [Crossref] [PubMed]
- Schröfelbauer B, Hakata Y, Landau NR. HIV-1 Vpr function is mediated by interaction with the damage-specific DNA-binding protein DDB1. Proc Natl Acad Sci U S A 2007;104:4130-5. [Crossref] [PubMed]
- Srivastava S, Swanson SK, Manel N, et al. Lentiviral Vpx accessory factor targets VprBP/DCAF1 substrate adaptor for cullin 4 E3 ubiquitin ligase to enable macrophage infection. PLoS Pathog 2008;4:e1000059. [Crossref] [PubMed]
- Liang X, Pickering MT, Cho NH, et al. Deregulation of DNA damage signal transduction by herpesvirus latency-associated M2. J Virol 2006;80:5862-74. [Crossref] [PubMed]
- Gastaldello S, Hildebrand S, Faridani O, et al. A deneddylase encoded by Epstein-Barr virus promotes viral DNA replication by regulating the activity of cullin-RING ligases. Nat Cell Biol 2010;12:351-61. [Crossref] [PubMed]