Recent advances in the development of farnesoid X receptor agonists
Introduction
The Farnesoid X receptors (FXR) belong to a family of receptors known as the nuclear hormone receptors (1,2). These proteins bind to cis-acting elements in the promoters of their target genes and modulate gene expression in response to metabolites (3). The FXR were first described in 1995 by Forman and co-workers (3). FXRs were found to be activated by the farnesol derivative, farnesyl pyrophosphate, which is a metabolic intermediate and the last precursor common to all branches of the mevalonic pathway, which leads to the biosynthesis of cholesterol, bile acids (BAs), sterol compounds, porphyrin, dolichol, ubiquinone, carotenoids, retinoids, vitamin D, steroid hormones, and farnesylated proteins (4). Recently, BAs were identified as physiological ligands of the FXRs (5,6). BAs are liver-related metabolites that are synthesized primarily by the liver (7). They are the end products of cholesterol catabolism and are important for absorption of nutrients such as fat-soluble vitamins and lipids (7). Normally, the BAs are produced by the liver cells and are stored in the gallbladder. BAs are then secreted by the gallbladder into the small intestine, where they participate in the digestion of food, and ultimately are reabsorbed back through the portal circulation into the liver (8). The circulation of BAs from the liver to the intestines and back to the liver is called the enterohepatic circulation. Hepatic BAs comprise only 5% of the total BA pool (7).
FXRs are highly expressed in tissues that participate in BA metabolism such as the liver, intestines, and kidneys (3). Both conjugated and unconjugated bile salts activate the FXRs at physiological concentrations (9,10). FXRs regulate BA homeostasis by regulating genes involved in BA synthesis, secretion, conjugation, transportation, absorption, and detoxification (11-15). When bound to BAs, FXRs repress the expression of the gene encoding cholesterol 7 alpha-hydroxylase, which is the rate-limiting enzyme in BA synthesis (5). Moreover, the expression of an important transport protein in the intestine is increased as a result of activation of the FXRs through binding with BAs (5,16); the cytosolic intestinal bile acid-binding protein (I-BABP) (17). This protein is thought to play an important role in the BA metabolism by facilitating the transport of the BAs from the intestines across the enterocytes and the portal circulation to the liver (5,16).
BAs have been found to affect liver regeneration and growth in liver injury and resection. In a mouse model (18), mice were fed either control or a 0.2% cholic acid (CA)-containing diet for 5 days. There was an approximately 30% increase in liver weight in the CA-fed mice compared to mice fed control diets. When livers were recovered, DNA synthesis was also noted to be increased, as demonstrated by a marked increase in hepatocyte nuclei staining with BrdU (18). These results suggest that supplementation with modest amounts of BAs is sufficient to drive hepatocyte proliferation. In a partial hepatectomy mouse model (18), mice were fed either a 0.2% CA-containing diet or a cholestyramine (resin)-containing diet for 5 days. CA-fed mice showed a significantly increased rate of liver growth and an increase in the number of BrdU-positive nuclei when compared to resin-fed mice (18). The role of the BA membrane transporter Mrp3 (Abcc3), which is expressed in liver and intestine, in hepatic regeneration was investigated in a partial hepatectomy mouse model (19). In that study, the liver growth and regeneration, elicited by CA feeding, were significantly impaired in the mice lacking Mrp3 (19). These mice also showed a decrease in portal circulation levels of BAs as well as impaired DNA synthesis (19). In a study of 46 patients who underwent hemihepatectomies with or without external biliary drainage, serum BAs and liver regeneration volumes were measured at 3- and 7-postoperative days (20). The mean serum BA levels and regenerated liver volumes were significantly higher in the group of patients without external biliary drainage (20). As expected, there was a significant positive association between the serum BA levels and the liver regeneration volumes (20). These results suggest that diverting BAs from the portal circulation decreases liver growth and DNA synthesis.
One critical FXR target gene in the gut is fibroblast growth factor-19 (FGF-19), a secreted growth factor that signals through the FGFR4 cell-surface receptor tyrosine kinase (21,22). In one study, FXR was found to directly regulate the expression of FGF-19, which in turn suppresses the expression of cholesterol 7 alpha-hydroxylase in human hepatocytes through a c-Jun N-terminal kinase-dependent pathway (23). Suppression of cholesterol 7 alpha-hydroxylase and decreased BA synthesis are known to be beneficial for liver regeneration (24,25). These results highlight the effect of FXR activation on liver growth and regeneration and their potential important implication in clinical practice.
FXR has been shown to be involved in liver cirrhosis. Activation of hepatic stellate cells (HSCs) is thought to mediate the process of liver fibrosis through the secretion of extracellular matrix proteins, ultimately leading to scarring of the liver tissue (26). Recent studies have shown that FXR is expressed in rat and human stellate cells (27,28), and that activation of FXR reduces the expression of extracellular matrix proteins by these cells (29). In addition, FXR activation has been shown to prevent and resolve liver fibrosis in rodents (28-30).
The effect of FXR on atherosclerosis has been examined in several animal models of atherosclerosis. The effect of FXR activation on aortic plaque formation was assessed by feeding apolipoprotein E-deficient mice with the synthetic FXR ligand 6-ethyl chenodeoxycholic acid (CDCA) at different doses (31). Administration of 6-ethyl CDCA reduced plaque formation by 95%, reduced aortic expression of the inflammatory cytokines interleukin (IL)-1β, IL-6, and CD11b (31). FXR activation also reduced hepatic expression of sterol regulatory element binding protein 1c, resulting in reduced triglyceride and cholesterol content in the liver and amelioration of hyperlipidemia (31). These findings suggest that FXR ligands might be used in the prevention and treatment of atherosclerosis.
FXR has been shown to be important in preventing gallstone formation in susceptible mice. In a study, FXR-deficient mice were found to be more susceptible to cholesterol gallstone formation than wild-type mice when fed a lithogenic diet (32). Moreover, treatment of C57L mice, which are susceptible to cholesterol gallstone formation, with a potent FXR agonist prevents gallstone formation (32).
FXR has been demonstrated to be involved in carcinogenesis. Mice lacking FXR were found to spontaneously develop hepatocellular adenomas and carcinomas after 12 months (33). In another mouse model, selective reactivation of intestinal FXR protected mice against development of HCC (34). These findings suggest that FXRs play a key role in the pathogenesis of HCC, and that FXR activation could prevent or treat HCC.
The FXR agonists are gaining attention as potential therapeutic agents in hepatobiliary disease because of their safety profiles and proven efficacy in experimental models of several human liver diseases. In this paper, we review the advances in the FXR agonists. Table 1 summarizes the clinical trials and ongoing studies of FXR agonists in different diseases.
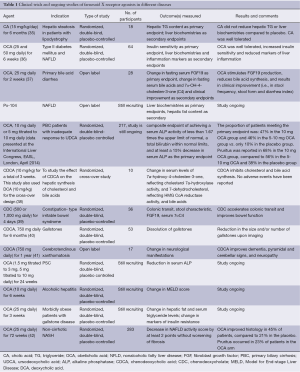
Full table
Nonsteroidal FXR agonists and their potential clinical uses
GW4064, developed by Maloney et al. (43), is a potent and selective nonsteroidal FXR agonist. It possesses an oral bioavailability of 10% with a half-life of 3.5 hours (43). The effect of GW4064 on serum triglycerides has been evaluated in a murine model in which rats were fed GW4064 by oral gavage. After 7 days, a dose-dependent lowering of serum triglycerides was observed in the rats receiving GW4064 (43). These results suggest that this FXR agonist could potentially be used in the treatment of dyslipidemia in humans.
WAY-362450 (FXR-450 or XL335), developed by Flatt et al. (44), is a potent and selective FXR agonist with an oral bioavailability of 38% and a protracted half-life of 25 hours (44). In a murine model, administration of WAY-362450 significantly lowered serum triglyceride and total cholesterol levels, and inhibited aortic atherosclerotic formation in a dose-dependent manner (44). These data suggest that WAY-362450 could be useful in treating patients with atherosclerotic vascular diseases.
PX-102 is a fully-synthetic, potent nonsteroidal FXR agonist that currently being evaluated in phase I clinical studies in healthy humans.
FXR agonists and alcoholic liver disease
Alcohol consumption is a major risk factor for chronic disease worldwide; it accounted for 3.8% of all deaths in 2004 (45). It is also a major cause of liver disease in Western countries. Alcohol-related liver deaths account for up to 48% of cirrhosis-associated deaths in the United States (46) and are also major contributors to liver disease-related mortality in other countries (45). The spectrum of alcoholic liver disease includes simple steatosis, alcoholic hepatitis, fibrosis, cirrhosis, and hepatocellular carcinoma (HCC). There are no targeted therapies for alcoholic liver disease, and treatment is mainly supportive with or without corticosteroids (46).
The role of the FXR in alcoholic liver disease has been investigated in a murine model. In this experiment, mice were fed Lieber-DeCarli ethanol diet or a control diet (47). FXR activity has been found to be impaired in this model, and treatment with the FXR agonist WAY-362450 protected the mice from development of alcoholic liver disease, possibly by suppressing the up-regulation of the microsomal cytochrome P450 2E1 enzyme, which has been shown to be the major contributor to alcohol-induced oxidative stress and liver injury (47). These data suggest that FXR plays an important role in the pathogenesis of alcoholic liver disease, and that FXR activation could be of therapeutic benefit. A phase II clinical trial of obeticholic acid (OCA), an FXR agonist, in alcoholic hepatitis is currently underway.
FXR agonists and nonalcoholic fatty liver disease (NAFLD)
Preclinical and clinical studies demonstrating beneficial effects of FXR agonists as potential therapeutic agents for the treatment of NAFLD/NASH
NAFLD is a common chronic liver disease in Western Society (48-50). NAFLD is closely associated with features of the metabolic syndrome such as insulin resistance, obesity, type II diabetes and hypertriglyceridemia (51). Nonalcoholic steatohepatitis (NASH), characterized histologically by hepatic steatosis, lobular inflammation and hepatocellular ballooning (52), progresses to cirrhosis of the liver in up to 15% of patients (53,54). About 90% of NAFLD patients exhibit at least one of the metabolic syndrome features, and one third exhibit three or more (55). Due to the lack of accurate biomarkers and invasiveness nature of liver tissue sampling, it is difficult to examine the true incidence and prevalence if NAFLD and NASH. In a recent study using liver biopsy involving 328 patients recruited from the Brooke Army Medical Center in the United States, the reported prevalence of NAFLD and NASH was 46% and 12.2% respectively (50). In this study, the prevalence of NASH was highest among the Hispanics (19.4% vs. 9.8% among the Caucasians) and among the diabetics (22.2% vs. 10.2% among the non-diabetics) (50). These data indicate that NAFLD and NASH are far more common than appreciated.
In addition to insulin resistance, oxidative stress and activation of the fibrogenetic pathways have both been implicated as key factors contributing to the chronic liver injury in patients with NASH (56-58). Recently, high dietary cholesterol, an activator of liver X receptor, has been shown to negatively affect the balance between storage and oxidation of fatty acids, which are in turn processed to non-triglyceride metabolites, such as diacylglycerol and lysophosphatidyl choline, that drive lipotoxic injury of liver cells (59-62). The type of dietary fat contributes to the development of NASH, as shown in mice fed on a diet high in trans-saturated fats (63). In addition, fructose, which depletes intracellular adenosine triphosphate (ATP), is transformed into lipids in the absence of insulin, thus increasing the amount of intracellular fat deposits and contributing to NAFLD and NASH, as also supported by the strong association of type II diabetes and NASH in individuals consuming high-fructose drinks (64). The depletion of hepatic ATP leads to mitochondrial dysfunction, generation of reactive oxygen species, inflammation, and endoplasmic reticulum stress, with subsequent activation of stress-related Jun N-terminal kinase which promotes hepatocyte apoptosis (65). Toxic lipid metabolites lead to activation of stress kinases and activation of cell death receptor signaling pathways, and trigger organelle dysfunction, leading to progress live cell injury (66). Other factors are activation of toll-like receptor 4 (TLR4) by intestinal bacterial lipopolysaccharide (67-69) and other pro-inflammatory signals produced by dysbiosis (gut microbiota imbalance) (70-72).
NASH is one of the top leading indications for liver transplantation. A United States study examined the trends of liver transplantation for NASH between 2001 and 2009 (73). Strikingly, the percentage of patients undergoing liver transplantation for NASH significantly increased from 1.2% in 2001 to 9.7% in 2009, making it the third most common indication for liver transplantation (73). It has been proposed by the authors of the paper that if the current trends continue, NASH will become the most common indication for liver transplantation in the United States in the next decades (73).
Studies have reported that NASH is a progressive disease and that simple hepatic steatosis rarely progresses to end-stage liver disease (74). The presence of fibrosis on liver biopsy, obesity, and insulin resistance has been identified as important risk factors for the progression of NASH (53,74,75). The overall survival of patients with NAFLD and NASH is reduced when compared to a matched reference population. NAFLD patients die from cardiovascular- and liver-related causes. In one study (54), a cohort of 129 patients with biopsy-proven NAFLD (of whom 14.7% had NASH at baseline) was followed for a mean of 13.7 years. During the follow-period, 15.5% of patients died from cardiovascular disease, and 5.4% of patients developed end-stage liver disease (54). Moreover, NASH cirrhosis has been identified as an important risk factor for HCC (54,76-78). It is unknown if NASH can result in HCC in the absence of cirrhosis. Reports from case series suggest that NASH can result in HCC in the absence of live cirrhosis (79-81). In a recent study, Ertle et al. (82) examined the prevalence of the metabolic syndrome and related clinical features in a cohort of 150 patients with an established diagnosis of HCC. They found that NASH was the second most common etiology of HCC (24% of all cases), and that features of the metabolic syndrome such as overweight, diabetes mellitus, hypertension and dyslipidemia were more common in the NASH-HCC group compared to the non-NASH-HCC group (82). Interestingly, 47.2% of the NASH-HCC exhibited no evidence of cirrhosis, suggesting that NASH or the metabolic syndrome may cause HCC even in the absence of cirrhosis (82). Larger and longer-term studies are required to clarify these results.
Currently, there is no effective medical therapy for NASH. Liver transplantation is the only potentially curative treatment for patients with NASH cirrhosis. Even after liver transplantation, NASH patients are at high risk for post-operative complications, cardiovascular events and mortality (up to 42% over a 12-month period) possibly due to associated obesity and diabetes (83,84).
FXRs play a critical role in carbohydrate and lipid metabolism and regulation of insulin sensitivity (85-88). The effect of the FXR on peripheral insulin resistance was examined in a mouse model (89). The ability of FXR −/− mice to respond to a glucose challenge was investigated. After a single intraperitoneal bolus injection of glucose, FXR −/− mice displayed a more pronounced plasma glucose up rise than the FXR +/+ mice (89). The decreased plasma glucose clearance in the same mouse model was further investigated to determine whether the decreased plasma glucose clearance resulted from impaired insulin secretion or peripheral insulin resistance by intraperitoneal insulin tolerance tests. The insulin-mediated decrease of plasma glucose was less pronounced in FXR −/− than in FXR +/+ mice (89). To directly evaluate the effect of FXR deficiency on whole-body insulin sensitivity, a hyperinsulinemic-euglycemic clamp test, commonly used to measure insulin resistance, was performed on 9-hour fasted FXR +/+ and FXR −/− mice. The glucose infusion rate (GIR) required to maintain euglycemia was 22% lower in the FXR −/− mice than in the FXR +/+ mice, indicative of existence of whole-body insulin resistance (89). Endogenous blood glucose production rate, which mainly reflects hepatic glucose production, was suppressed to similar values with insulin infusion in both FXR +/+ and FXR −/− mice, indicating the absence of hepatic insulin resistance in FXR −/− mice (89). Moreover, the insulin-stimulated glucose disposal rate was reduced by 20% in FXR −/− mice (89). These results indicate that FXR deficiency results in peripheral insulin resistance, an important feature in NASH.
Preclinical studies have shown that FXR activation improves insulin sensitivity. In a mouse model of insulin resistance, genetically obese mice (ob/ob) received either the FXR agonist GW4064 or vehicle for 10 days (89). Plasma insulin concentration significantly decreased on day 10 in the treatment group, suggesting an improvement in the insulin sensitivity in the GW4064-treated mice. Furthermore, results from a glucose tolerance test (by injecting glucose intraperitoneally after a 6-hour fast) and impaired tolerance test (by injecting insulin intraperioneally after a 6-hour fast) showed improved glucose clearance and enhanced insulin sensitivity in GW4064-treated mice when compared to controls (89). These results indicate that FXR activation results in improved insulin sensitivity.
FXR activation lowers plasma glucose, free fatty acids, triglycerides, and total cholesterol. In a mouse model (88), FXR +/+ and FXR −/− mice were treated with GW4064 for 11 days. After 4 days of treatment, plasma glucose, free fatty acids, and triglycerides decreased significantly in FXR +/+ but not in FXR −/− mice (88). FXR activation has also been shown to improve plasma glucose concentrations in a diabetic mouse model (88). The db/db mice lack functional leptin receptors and have been extensively studied as a model for type II diabetes mellitus (90,91). Diabetic db/db mice and their lean littermates were treated with GW4064 or vehicle for 5 days (88). Treatment of db/db mice with GW4064 significantly decreased plasma levels of glucose, fatty acids and triglycerides (88). Together, these results indicate that FXR activation results in a reduction in plasma levels of glucose, free fatty acids, and triglycerides, underscoring the key role of FXR in regulation of carbohydrate and lipid metabolism.
In addition to their direct role, FXR indirectly regulates carbohydrate and lipid metabolism and insulin sensitivity through increasing the secretion of FGF-19 into the small intestine (92). In turn, FGF-19 has been found to improve indices of hyperlipidemia, hyperinsulinemia, hepatic steatosis, and insulin sensitivity in mice fed a high-fat diet (93). In addition, FGF-19 treatment decreases hepatic triglycerides and free fatty acids levels in FXR −/− mice (94).
The effect of FXR activation on the development and protection against NASH has been investigated in animal models. Feeding mice a methionine and choline-deficient (MCD) diet is a well-established nutritional model of NASH resulting in serum elevation of alanine aminotransferase (ALT) and aspartate aminotransferase (AST), and liver histological abnormalities similar to human NASH, including hepatic steatosis, lobular inflammation, and pericellular fibrosis (95,96). C57BL/6 mice were fed an MCD diet and treated with or without WAY-362450 (a synthetic FXR agonist) for 4 weeks (97). The elevations of serum ALT and AST induced by the MCD diet were markedly reduced with WAY-362450 treatment (97). In addition, treatment with WAY-362450 markedly reduced inflammatory cell infiltration and hepatic fibrosis (97). The reduction in inflammatory cell infiltration correlated with decreased serum levels of the inflammatory markers keratinocyte derived chemokine (mKC) and monocyte chemokine protein-1 (MCP) and decreased hepatic gene expression of MCP-1 and vascular cell adhesion molecule-1 (VCAM-1). The reduction of hepatic fibrosis by WAY-362450 correlated with a decrease in hepatic gene expression of fibrosis markers (97). Moreover, the hepatoprotective effects of WAY-362450 were abolished in FXR −/− mice fed an MCD diet (97). These results indicate that FXR agonists may be useful for the treatment NASH.
Preliminary data suggest that BA accumulation could override triglyceride accumulation in the liver during liver injury induced by NAFLD. In an animal model of NASH (98), wild type (WT) C57BL/6J and FXR −/− mice were fed with MCD diet for 4 weeks. FXR−/− mice developed more severe liver injury, assessed by serum ALT and AST and histology, than WT mice. Interestingly, FXR−/− MCD fed mice developed lower degrees of hepatic steatosis and higher degrees of hepatic cholestasis (98). Moreover, the super-physiological concentrations of hepatic BAs in the FXR −/− MCD fed mice inhibited the expression of genes involved in fatty acid uptake and triglyceride accumulation, which may be an explanation for the less hepatic steatosis in FXR −/− MCD fed mice in contrast to WT MCD fed mice (98). These results suggest that BA accumulation in the liver tissue is an important step in the liver injury caused by NAFLD.
Preliminary human data have shown that BAs levels are increased in patients with NAFLD/NASH, suggesting that BAs could be detrimental in patients with NAFLD/NASH. Recently, Bechmann et al. (99) examined liver biopsies and serum markers of cell death, free fatty acids (FFAs), and serum BAs from 113 obese patients receiving bariatric surgery. In this study, they found that serum FFAs and BAs were significantly higher in patients with NASH compared to controls and that serum adiponectin levels were markedly reduced in NASH patients when compared to controls (99). In addition, the BA transporter N+/taurocholate cotransporter and the BA synthesizing enzyme cholesterol 7α-hydroxylase were significantly up-regulated in obese patients and hepatoma cells upon exposure to FFA, indicating failure to activate small heterodimer partner (SHP) upon FXR stimulation by increasing BA (CDCA) concentration, even though SHP was only modestly decreased (99). An important finding is the higher serum BAs levels in patients with advanced ballooning on biopsy, an important histological feature in NASH (99). Moreover, they observed a direct inverse relationship between the adiponectin and serum BA (99). Taken all together, these data suggest that BAs are detrimental in NASH and adiponectin may have a role in BA metabolism.
OCA as a potential therapeutic agent for NAFLD/NASH- preclinical studies
OCA, also known as INT-747, a 6α-ethyl derivative of CDCA, is a first-in-class selective FXR agonist (100). Sixα-ethyl CDCA was first described in 2002 as a potent and selective steroidal FXR agonist (101). In addition to its potent role in promoting bile flow (101), 6-ECDCA protected hepatocytes against acute necrosis caused by lithocholic acid (LCA) in a male Wistar rat model of cholestasis (101). OCA has an approximately 100-fold greater FXR agonistic activity than CDCA (102).
In a rabbit model of the metabolic syndrome (103), a high-fat diet resulted in an increase in visceral fat, fasting glycemia and glucose intolerance. Treatment with OCA along with the high-fat diet normalized visceral fat fasting glucose levels, and improved glucose tolerance (103). The effect of OCA on insulin resistance and development of hepatic steatosis has been studied in Zucker fa/fa obese rats (104), a model for NAFLD with a loss-of-function mutation of the leptin receptor. These rats exhibit hyperphagia and hyperleptinemia and develop obesity, insulin resistance, diabetes, and hepatic steatosis (105-107). In this study, in comparison to lean rats, fa/fa rats on a normal diet developed insulin resistance and hepatic steatosis (104). Administration of OCA reversed insulin resistance and hepatic steatosis and protected against body weight gain and liver and muscle fat deposition (104). Moreover, FXR activation resulted in a reduction of liver expression of genes involved in fatty acid synthesis, lipogenesis and gluconeogenesis (104). In muscle, FXR activation reduced free fatty acid synthesis (104).
In addition to its role in reversing insulin resistance and hepatic steatosis, OCA displays a wide range of anti-inflammatory and anti-fibrotic activities, which have been demonstrated in several animal models. OCA treatment reduced AST and plasma levels of interferon-ɣ and tumor necrosis factor-α in a mouse model of autoimmune hepatitis (108), reduced the expression of profibrotic and fibrosis markers in a mouse model of nephropathy (109), and attenuated colon injury by increasing colon expression of FXR and SHP and reducing levels of IL-1, IL-2 and IL-6 in a mouse model of colitis (110,111).
OCA as a potential therapeutic agent for NAFLD/NASH—clinical studies
The safety and effect of OCA on insulin sensitivity has been investigated in a randomized, placebo-controlled clinical trial in patients with NAFLD and type II diabetes (36). In this study, patients were assigned to receive 25 mg OCA, 50 mg OCA, or placebo, once daily for 6 weeks. The primary endpoint was the GIR, assessed by the hyperinsulinemic-euglycemic clamp technique (112). This technique is considered gold standard for assessment of insulin sensitivity. When patients were given low-dose insulin infusion, insulin sensitivity significantly improved by 28% and 20.1% from baseline in the 25 and 50 mg OCA groups, respectively, while insulin sensitivity decreased by 5.5% in the placebo group (36). A similar pattern of change in insulin sensitivity was observed when patients were given high-dose insulin infusion (36). Moreover, a significant reduction in levels of ɣ-glutamyltransferase (GGT) and ALT and liver fibrosis markers were observed in the treatment groups (36). Adverse events were similar in all groups (36). These results are promising and larger and longer-term clinical trials are needed to assess the potential the impact of OCA and other FXR agonists on the long-term outcomes of NAFLD/NASH.
Recently, the results of the Farnesoid X nuclear receptor ligand OCA in NASH treatment (the FLINT) trial were reported (42). In this multicenter, double-blinded, placebo controlled clinical trial, a total of 283 patients with biopsy-proven NASH were randomized to receive either OCA 25 mg orally daily or placebo for 72 weeks. The primary outcome measure was improvement in NAFLD activity score by at least two points without worsening of fibrosis from baseline to the end of treatment. At the time of analysis of the primary outcome, 110 patients in the OCA arm and 109 patients in the placebo arm were included in the analysis (42). At 72 weeks of treatment, the percentage of patients who demonstrated histological improvement in the OCA and placebo arm was 45% and 21%, respectively (42). Pruritus, a known side effect of OCA, developed in 23% of the 141 patients in the OCA arm versus 6% of the 142 patients in the placebo arm (42). Of note, a decrease in the high-density lipoprotein (HDL), and an increase in the total cholesterol and low-density lipoprotein (LDL) was observed in patients in the OCA arm compared to placebo (42). These results suggest that OCA might be beneficial in preventing progression of NASH. Long-term studies are needed to explore the clinical relevance of FXR ligand treatment-related dyslipidemia.
FXR agonists and the cholestatic liver disease [primary biliary cirrhosis (PBC) and primary sclerosing cholangitis (PSC)]
OCA as a potential therapeutic agent for cholestatic liver diseases—preclinical and clinical studies
In a Wistar rat model of cholestasis, OCA promoted bile flow and protected the hepatocytes against acute necrosis caused by administration of LCA (101). In another rodent model of bile duct ligated (BDL) rats, the administration of OCA reduced liver fibrosis and α-collagen 1, transforming growth factor-β1 (TGF-β1), and tissue metalloproteinase inhibitor-1 (29). Collectively, these results indicate that FXR activation could be beneficial in patients with cholestatic liver diseases.
PBC is a chronic, progressive, cholestatic liver disease characterized histologically by destruction of intrahepatic bile ducts and serologically by the presence of the antimitochondrial antibodies (AMAs) (113). AMA is a highly disease-specific autoantibody (114), rarely found in individuals without PBC (115). The diagnosis of PBC is suspected in the setting of cholestasis and presence of AMA (116). Liver biopsy, once an important diagnostic step to establish or rule out PBC, is now rarely performed to confirm a diagnosis of PBC, as ~95% of PBC patients test positive for AMA (114). The targets of the AMAs are members of a family of enzymes, the 2-oxo-acid dehydrogenase complexes (117-120). The etiology of PBC is poorly understood. In addition to female sex, several other clinical variables such as infections (particularly urinary tract infections), use of chemicals and xenobiotics (such as 2-Octynoic acid), smoking and having a first-degree family member with PBC have been implicated as risk factors for PBC (121-123). Recent genome-wide association studies (GWAS) have identified loci strongly associated with PBC, such as HLA, IL12A, IL12RB2, SPIB, IRF5-TNPO3, 17q12-21, STAT4, DENND1B, CD80, IL7R, CXCR5, TNFRSF1A, CLEC16A, and NFKB1 (124-129). Epidemiological studies have reported a prevalence of PBC ranging from 19 to 365 cases per million, and an incidence of 4 to 58 cases per million persons-years (130-135). The incidence and prevalence of PBC may be increasing, according to a recent Dutch population-based study (135). PBC affects mainly middle-aged women, with a female: male ratio of 10:1 (136). The majority of PBC patients are asymptomatic (113). Fatigue and pruritus are the most common symptoms in PBC (113). The etiology of fatigue is poorly understood; however, degenerative brain changes in the areas regulating autonomic functions as a result of chronic cholestasis have been proposed as a cause of fatigue in PBC (137). Fatigue can be very disabling in PBC, often affects patients’ quality of life, frequently associated with depression, is associated with an overall reduced survival, and in some extreme cases is an indication for liver transplantation (138-145). A significant proportion of PBC patients continue to suffer from severe fatigue even after liver transplantation (146). Pruritus is another manifestation of PBC (147). The cause of pruritus in PBC is unknown. Recently, lysophosphatidic acid (LPA) and autotaxin, the serum enzyme converting lysophosphatidylcholine into LPA, have been found in higher concentrations in the sera of patients with cholestatic disorders compared to control, suggesting that LPA and autotaxin play a critical role in cholestatic pruritus (148). PBC may lead to hepatic fibrosis, cirrhosis, and eventually liver failure (136). In the pre-UDCA era, the 10-year survival of PBC patients was 50-70%, even worse in symptomatic PBC patients, with a reported median survival of 5 to 8 years (149). PBC is an important indication for liver transplantation in the United States and Europe (150). Currently, the only therapy approved by the United States Food and Drug Administration (US FDA) is ursodeoxycholic acid (UDCA). Several randomized controlled clinical trials have shown that long-term administration of UDCA in PBC patients delays histological progression to cirrhosis and prolongs survival without liver transplantation (151-157). However, up to 40% of PBC patients have incomplete response to UDCA (158). Therefore, there is a critical need for other effective therapies for PBC patients who are at high risk for progressive disease. Recently, a meta-analysis international follow up study of 4,845 patients with PBC has shown that levels of serum ALP and serum bilirubin correlate well with long-term outcomes of patients with PBC (159). Thus, serum ALP and bilirubin could be used as surrogate markers of clinical endpoints when designing therapeutic trials in PBC.
PSC is a progressive disease of the liver characterized by cholestasis and ongoing destruction of intra- and extra-hepatic bile ducts, leading ultimately to fibrosis, cirrhosis, and liver failure (160). The diagnosis of PSC is made in the setting of cholestasis and cholangiographic evidence of intra- and/or extra-hepatic biliary ductal structuring (161). Small-duct PSC is a variant of PSC which is characterized by cholestatic and histological evidence of PSC but normal cholangiography (162-164). PSC occurs more often in men than in women, and frequently occurs in association with the inflammatory bowel disease ulcerative colitis (UC) (165), suggesting an immunological mechanism for the disease. The etiology of PSC is poorly understood. Data from large randomized controlled clinical trials suggest that the accumulation of toxic BAs could be detrimental to the hepatic tissue in PSC patients (166,167). Patients with PSC are frequently asymptomatic, but patients may also present with abdominal pain, pruritus, and bacterial cholangitis (161).
PSC can progress to liver fibrosis, cirrhosis, and ultimately liver failure (168-171). PSC is an important risk factor for cholangiocarcinoma (CCA), which is the most common primary biliary malignancy, and the second most common primary liver cancer after HCC (172-174). CCA is a very aggressive disease, often diagnosed in late stages. The percentage of CCA patients who survive 5 years after diagnosis is only 10% (174).
Several agents including immunosuppressants and immunomodulators have been studied in PSC patients but failed to show positive results (175). UDCA has been extensively studied in large and long-term clinical trials in PSC but failed to demonstrate a positive impact on the long-term clinical outcomes in PSC (166,176). High-dose UDCA has been associated with adverse events in PSC patients (166,177). At this time, there is no medical therapy proven to be beneficial for patients with PSC, and liver transplantation is the only potentially curative option for patients with PSC. It is the fifth leading indication for liver transplantation in the United States (178), and in some Scandinavian countries where the prevalence of viral hepatitis B and C are low, is the leading indication for liver transplantation (178). PSC recurs in the transplanted liver in up to 40% (179). Clinical trials evaluating potentially effective therapies for PSC are urgently needed.
The safety and efficacy of OCA has been evaluated in an international, double-blind, placebo controlled, dose-response study in 165 patients with PBC with persistently elevated serum ALP levels while on stable doses of UDCA (180). The primary endpoint was a change in serum ALP. Patients were randomly assigned to the following groups: placebo, 10, 25, and 50 mg OCA. Patients received treatment once daily for 12 weeks, while remaining on UDCA. A significant reduction in serum ALP levels from baseline was observed in the treatment groups (23.7% in the 10 mg group, 24.7% in the 25 mg group, and 21% in the 50 mg group) compared to placebo (2.6%) (180). Moreover, patients in the treatment groups had significant reduction in serum GGT (48% in the 10 mg group, 63% in the 25 mg group, 57% in the 50 mg group) and ALT(28% in the 10 mg group, 35% in the 25 mg group, 21% in the 50 mg group) when compared to placebo (GGT increased by 7%; ALT, no change) (180). Pruritus was the most common adverse experience in this study (47% in the 10 mg group, 85% in the 25 mg group, 80% in the 50 mg group, and 50% in the placebo group), leading to discontinuation of the drug in 8% in the 10 and 25 mg groups, and in 24% of the 50 mg group (180). Serious adverse events (GI bleeding and jaundice) were reported in 7 patients, 75% in the 50 mg group (180).
In another study, 59 PBC patients off UDCA for 6 months were randomly assigned to receive placebo, 10 or 50 mg OCA once daily for 12 weeks (181). In this study, OCA was administered as monotherapy. The primary endpoint was a change in serum ALP levels. Patients in the treatment groups achieved a significant reduction in serum ALP (44% in the 10 mg group, 37% in the 50 mg group), serum GGT (73% in the 10 mg group, 65% in the 50 mg group), and serum ALT (37% in the 10 mg group, 35% in the 50 mg group) when compared to placebo (ALP increased by 0.4%, GGT reduced by 3%, and ALT reduced by 4%) (181). Pruritus was the most common adverse event (70% in the 10 mg group and 94% in the 50 mg group, compared to 30% in the placebo group), leading to drug discontinuation in 15% of the 10 mg group and in 38% of the 50 mg group (181).
Initial results from an international 1-year phase III clinical trial of OCA in PBC patients were reported in the International Liver Congress in April 2014. A total of 217 patients with PBC whom previously had an inadequate response to UDCA were randomly assigned to receive placebo, OCA 10 mg daily, or OCA 5 mg daily titrated to 10 mg daily. The primary endpoint was a composite endpoint of achieving a serum ALP activity of less than 1.67 times the upper limit of normal, a total bilirubin within normal limits, and at least a 15% decrease in serum ALP. The proportion of patients meeting the primary endpoint was: 47% in the 10 mg OCA group and 46% in the 5-10 mg OCA group vs. only 10% in the placebo group. In addition, both OCA dose groups met secondary endpoints of improvements in other liver function parameters, GG, ALT and total bilirubin. Pruritus, generally mild to moderate, was the most frequently reported adverse event associated with OCA treatment (placebo: 38%, OCA 10 mg: 68%, OCA 5-10 mg titration: 56%). However, only a few patients withdrew due to pruritus: none in the placebo group, seven (10%) of the patients in the 10 mg OCA group, and only one (1%) of the patients in the OCA 5-10 mg titration group. Together, these results indicate that OCA could be an effective therapy for patients with PBC. Currently, a phase II clinical trial of OCA in PSC is ongoing.
Conclusions
FXR agonists are an attractive class of drugs for patients with chronic liver diseases. In addition to their critical role in regulating BA, carbohydrate, and lipid metabolism, they also possess potent anti-inflammatory and anti-fibrotic properties. Clinical experience with OCA in patients with chronic liver disease indicates that FXR agonists are safe and effective. Larger and longer term clinical trials are warranted to explore the beneficial effects of the FXR agonists in patients suffering from chronic liver diseases.
Acknowledgements
Disclosure: Dr. Keith Lindor is an unpaid advisor for Intercept and Lumena. The authors declare no conflict of interest.
References
- Evans RM. The steroid and thyroid hormone receptor superfamily. Science 1988;240:889-95. [PubMed]
- Giguère V, Yang N, Segui P, et al. Identification of a new class of steroid hormone receptors. Nature 1988;331:91-4. [PubMed]
- Forman BM, Goode E, Chen J, et al. Identification of a nuclear receptor that is activated by farnesol metabolites. Cell 1995;81:687-93. [PubMed]
- Goldstein JL, Brown MS. Regulation of the mevalonate pathway. Nature 1990;343:425-30. [PubMed]
- Makishima M, Okamoto AY, Repa JJ, et al. Identification of a nuclear receptor for bile acids. Science 1999;284:1362-5. [PubMed]
- Wang H, Chen J, Hollister K, et al. Endogenous bile acids are ligands for the nuclear receptor FXR/BAR. Mol Cell 1999;3:543-53. [PubMed]
- Fan M, Wang X, Xu G, et al. Bile acid signaling and liver regeneration. Biochim Biophys Acta 2014. [Epub ahead of print]. [PubMed]
- Arias I, Boyer JL, Boyer J, et al. eds. The liver: biology and pathobiology. Philadelphia: Lippincott Williams & Wilkins, 2001.
- Claudel T, Staels B, Kuipers F. The Farnesoid X receptor: a molecular link between bile acid and lipid and glucose metabolism. Arterioscler Thromb Vasc Biol 2005;25:2020-30. [PubMed]
- Wang YD, Chen WD, Moore DD, et al. FXR: a metabolic regulator and cell protector. Cell Res 2008;18:1087-95. [PubMed]
- Cariou B, Staels B. FXR: a promising target for the metabolic syndrome? Trends Pharmacol Sci 2007;28:236-43. [PubMed]
- Edwards PA, Kast HR, Anisfeld AM. BAREing it all: the adoption of LXR and FXR and their roles in lipid homeostasis. J Lipid Res 2002;43:2-12. [PubMed]
- Li J, Pircher PC, Schulman IG, et al. Regulation of complement C3 expression by the bile acid receptor FXR. J Biol Chem 2005;280:7427-34. [PubMed]
- Sinal CJ, Tohkin M, Miyata M, et al. Targeted disruption of the nuclear receptor FXR/BAR impairs bile acid and lipid homeostasis. Cell 2000;102:731-44. [PubMed]
- Zhang Y, Castellani LW, Sinal CJ, et al. Peroxisome proliferator-activated receptor-gamma coactivator 1alpha (PGC-1alpha) regulates triglyceride metabolism by activation of the nuclear receptor FXR. Genes Dev 2004;18:157-69. [PubMed]
- Gong YZ, Everett ET, Schwartz DA, et al. Molecular cloning, tissue distribution, and expression of a 14-kDa bile acid-binding protein from rat ileal cytosol. Proc Natl Acad Sci U S A 1994;91:4741-5. [PubMed]
- Kanda T, Foucand L, Nakamura Y, et al. Regulation of expression of human intestinal bile acid-binding protein in Caco-2 cells. Biochem J 1998;330:261-5. [PubMed]
- Huang W, Ma K, Zhang J, et al. Nuclear receptor-dependent bile acid signaling is required for normal liver regeneration. Science 2006;312:233-6. [PubMed]
- Fernández-Barrena MG, Monte MJ, Latasa MU, et al. Lack of Abcc3 expression impairs bile-acid induced liver growth and delays hepatic regeneration after partial hepatectomy in mice. J Hepatol 2012;56:367-73. [PubMed]
- Otao R, Beppu T, Isiko T, et al. External biliary drainage and liver regeneration after major hepatectomy. Br J Surg 2012;99:1569-74. [PubMed]
- Goldfarb M. Signaling by fibroblast growth factors: the inside story. Sci STKE 2001;2001:pe37.
- Ornitz DM, Itoh N. Fibroblast growth factors. Genome Biol 2001;2:REVIEWS3005.
- Holt JA, Luo G, Billin AN, et al. Definition of a novel growth factor-dependent signal cascade for the suppression of bile acid biosynthesis. Genes Dev 2003;17:1581-91. [PubMed]
- Song KH, Ellis E, Strom S, et al. Hepatocyte growth factor signaling pathway inhibits cholesterol 7alpha-hydroxylase and bile acid synthesis in human hepatocytes. Hepatology 2007;46:1993-2002. [PubMed]
- Zhang L, Huang X, Meng Z, et al. Significance and mechanism of CYP7a1 gene regulation during the acute phase of liver regeneration. Mol Endocrinol 2009;23:137-45. [PubMed]
- Friedman SL. Liver fibrosis -- from bench to bedside. J Hepatol 2003;38 Suppl 1:S38-53. [PubMed]
- Lee FY, Kast-Woelbern HR, Chang J, et al. Alpha-crystallin is a target gene of the farnesoid X-activated receptor in human livers. J Biol Chem 2005;280:31792-800. [PubMed]
- Fiorucci S, Rizzo G, Antonelli E, et al. A farnesoid x receptor-small heterodimer partner regulatory cascade modulates tissue metalloproteinase inhibitor-1 and matrix metalloprotease expression in hepatic stellate cells and promotes resolution of liver fibrosis. J Pharmacol Exp Ther 2005;314:584-95. [PubMed]
- Fiorucci S, Antonelli E, Rizzo G, et al. The nuclear receptor SHP mediates inhibition of hepatic stellate cells by FXR and protects against liver fibrosis. Gastroenterology 2004;127:1497-512. [PubMed]
- Fiorucci S, Rizzo G, Antonelli E, et al. Cross-talk between farnesoid-X-receptor (FXR) and peroxisome proliferator-activated receptor gamma contributes to the antifibrotic activity of FXR ligands in rodent models of liver cirrhosis. J Pharmacol Exp Ther 2005;315:58-68. [PubMed]
- Mencarelli A, Renga B, Distrutti E, et al. Antiatherosclerotic effect of farnesoid X receptor. Am J Physiol Heart Circ Physiol 2009;296:H272-81. [PubMed]
- Moschetta A, Bookout AL, Mangelsdorf DJ. Prevention of cholesterol gallstone disease by FXR agonists in a mouse model. Nat Med 2004;10:1352-8. [PubMed]
- Kim I, Morimura K, Shah Y, et al. Spontaneous hepatocarcinogenesis in farnesoid X receptor-null mice. Carcinogenesis 2007;28:940-6. [PubMed]
- Degirolamo C, Modica S, Vacca M, et al. Prevention of spontaneous hepatocarcinogenesis in farnesoid X receptor-null mice by intestinal-specific farnesoid X receptor reactivation. Hepatology 2015;61:161-70. [PubMed]
- Ahmad Z, Subramanyam L, Szczepaniak L, et al. Cholic acid for hepatic steatosis in patients with lipodystrophy: a randomized, controlled trial. Eur J Endocrinol 2013;168:771-8. [PubMed]
- Mudaliar S, Henry RR, Sanyal AJ, et al. Efficacy and safety of the farnesoid X receptor agonist obeticholic acid in patients with type 2 diabetes and nonalcoholic fatty liver disease. Gastroenterology 2013;145:574-82. [PubMed]
- Walters JR, Johnston IM, Nolan JD, et al. The response of patients with bile acid diarrhoea to the farnesoid X receptor agonist obeticholic acid. Aliment Pharmacol Ther 2015;41:54-64. [PubMed]
- Einarsson C, Hillebrant CG, Axelson M. Effects of treatment with deoxycholic acid and chenodeoxycholic acid on the hepatic synthesis of cholesterol and bile acids in healthy subjects. Hepatology 2001;33:1189-93. [PubMed]
- Rao AS, Wong BS, Camilleri M, et al. Chenodeoxycholate in females with irritable bowel syndrome-constipation: a pharmacodynamic and pharmacogenetic analysis. Gastroenterology 2010;139:1549-58. [PubMed]
- Thistle JL, Hofmann AF. Efficacy and specificity of chenodeoxycholic acid therapy for dissolving gallstones. N Engl J Med 1973;289:655-9. [PubMed]
- Berginer VM, Salen G, Shefer S. Long-term treatment of cerebrotendinous xanthomatosis with chenodeoxycholic acid. N Engl J Med 1984;311:1649-52. [PubMed]
- Neuschwander-Tetri BA, Loomba R, Sanyal AJ, et al. Farnesoid X nuclear receptor ligand obeticholic acid for non-cirrhotic, non-alcoholic steatohepatitis (FLINT): a multicentre, randomised, placebo-controlled trial. Lancet 2014. [Epub ahead of print]. [PubMed]
- Maloney PR, Parks DJ, Haffner CD, et al. Identification of a chemical tool for the orphan nuclear receptor FXR. J Med Chem 2000;43:2971-4. [PubMed]
- Flatt B, Martin R, Wang TL, et al. Discovery of XL335 (WAY-362450), a highly potent, selective, and orally active agonist of the farnesoid X receptor (FXR). J Med Chem 2009;52:904-7. [PubMed]
- Rehm J, Mathers C, Popova S, et al. Global burden of disease and injury and economic cost attributable to alcohol use and alcohol-use disorders. Lancet 2009;373:2223-33. [PubMed]
- Gao B, Bataller R. Alcoholic liver disease: pathogenesis and new therapeutic targets. Gastroenterology 2011;141:1572-85. [PubMed]
- Wu W, Zhu B, Peng X, et al. Activation of farnesoid X receptor attenuates hepatic injury in a murine model of alcoholic liver disease. Biochem Biophys Res Commun 2014;443:68-73. [PubMed]
- Browning JD, Szczepaniak LS, Dobbins R, et al. Prevalence of hepatic steatosis in an urban population in the United States: impact of ethnicity. Hepatology 2004;40:1387-95. [PubMed]
- Schwimmer JB, Deutsch R, Kahen T, et al. Prevalence of fatty liver in children and adolescents. Pediatrics 2006;118:1388-93. [PubMed]
- Williams CD, Stengel J, Asike MI, et al. Prevalence of nonalcoholic fatty liver disease and nonalcoholic steatohepatitis among a largely middle-aged population utilizing ultrasound and liver biopsy: a prospective study. Gastroenterology 2011;140:124-31. [PubMed]
- Marchesini G, Brizi M, Bianchi G, et al. Nonalcoholic fatty liver disease: a feature of the metabolic syndrome. Diabetes 2001;50:1844-50. [PubMed]
- Ludwig J, Viggiano TR, McGill DB, et al. Nonalcoholic steatohepatitis: Mayo Clinic experiences with a hitherto unnamed disease. Mayo Clin Proc 1980;55:434-8. [PubMed]
- Adams LA, Sanderson S, Lindor KD, et al. The histological course of nonalcoholic fatty liver disease: a longitudinal study of 103 patients with sequential liver biopsies. J Hepatol 2005;42:132-8. [PubMed]
- Ekstedt M, Franzen LE, Mathiesen UL, et al. Long-term follow-up of patients with NAFLD and elevated liver enzymes. Hepatology 2006;44:865-73. [PubMed]
- Almeda-Valdés P, Cuevas-Ramos D, Aguilar-Salinas CA. Metabolic syndrome and non-alcoholic fatty liver disease. Ann Hepatol 2009;8 Suppl 1:S18-24. [PubMed]
- McClain CJ, Mokshagundam SP, Barve SS, et al. Mechanisms of non-alcoholic steatohepatitis. Alcohol 2004;34:67-79. [PubMed]
- Sanyal AJ. Insulin resistance and nonalcoholic steatohepatitis: fat or fiction? Am J Gastroenterol 2001;96:274-6. [PubMed]
- Sanyal AJ, Campbell-Sargent C, Mirshahi F, et al. Nonalcoholic steatohepatitis: association of insulin resistance and mitochondrial abnormalities. Gastroenterology 2001;120:1183-92. [PubMed]
- Savard C, Tartaglione EV, Kuver R, et al. Synergistic interaction of dietary cholesterol and dietary fat in inducing experimental steatohepatitis. Hepatology 2013;57:81-92. [PubMed]
- Neuschwander-Tetri BA, Wang DQ. Excess cholesterol and fat in the diet: a dangerous liaison for energy expenditure and the liver. Hepatology 2013;57:7-9. [PubMed]
- Kalaany NY, Gauthier KC, Zavacki AM, et al. LXRs regulate the balance between fat storage and oxidation. Cell Metab 2005;1:231-44. [PubMed]
- Neuschwander-Tetri BA. Hepatic lipotoxicity and the pathogenesis of nonalcoholic steatohepatitis: the central role of nontriglyceride fatty acid metabolites. Hepatology 2010;52:774-88. [PubMed]
- Neuschwander-Tetri BA, Ford DA, Acharya S, et al. Dietary trans-fatty acid induced NASH is normalized following loss of trans-fatty acids from hepatic lipid pools. Lipids 2012;47:941-50. [PubMed]
- Abdelmalek MF, Suzuki A, Guy C, et al. Increased fructose consumption is associated with fibrosis severity in patients with nonalcoholic fatty liver disease. Hepatology 2010;51:1961-71. [PubMed]
- Abdelmalek MF, Lazo M, Horska A, et al. Higher dietary fructose is associated with impaired hepatic adenosine triphosphate homeostasis in obese individuals with type 2 diabetes. Hepatology 2012;56:952-60. [PubMed]
- Schattenberg JM, Schuchmann M. Diabetes and apoptosis: liver. Apoptosis 2009;14:1459-71. [PubMed]
- Spruss A, Kanuri G, Wagnerberger S, et al. Toll-like receptor 4 is involved in the development of fructose-induced hepatic steatosis in mice. Hepatology 2009;50:1094-104. [PubMed]
- Iacono A, Raso GM, Canani RB, et al. Probiotics as an emerging therapeutic strategy to treat NAFLD: focus on molecular and biochemical mechanisms. J Nutr Biochem 2011;22:699-711. [PubMed]
- Ye D, Li FY, Lam KS, et al. Toll-like receptor-4 mediates obesity-induced non-alcoholic steatohepatitis through activation of X-box binding protein-1 in mice. Gut 2012;61:1058-67. [PubMed]
- Machado MV, Cortez-Pinto H. No need for a large belly to have NASH. J Hepatol 2011;54:1090-3. [PubMed]
- Rabot S, Membrez M, Bruneau A, et al. Germ-free C57BL/6J mice are resistant to high-fat-diet-induced insulin resistance and have altered cholesterol metabolism. FASEB J 2010;24:4948-59. [PubMed]
- Zhu L, Baker SS, Gill C, et al. Characterization of gut microbiomes in nonalcoholic steatohepatitis (NASH) patients: a connection between endogenous alcohol and NASH. Hepatology 2013;57:601-9. [PubMed]
- Charlton MR, Burns JM, Pedersen RA, et al. Frequency and outcomes of liver transplantation for nonalcoholic steatohepatitis in the United States. Gastroenterology 2011;141:1249-53. [PubMed]
- Vernon G, Baranova A, Younossi ZM. Systematic review: the epidemiology and natural history of non-alcoholic fatty liver disease and non-alcoholic steatohepatitis in adults. Aliment Pharmacol Ther 2011;34:274-85. [PubMed]
- Fassio E, Alvarez E, Dominguez N, et al. Natural history of nonalcoholic steatohepatitis: a longitudinal study of repeat liver biopsies. Hepatology 2004;40:820-6. [PubMed]
- Bugianesi E, Leone N, Vanni E, et al. Expanding the natural history of nonalcoholic steatohepatitis: from cryptogenic cirrhosis to hepatocellular carcinoma. Gastroenterology 2002;123:134-40. [PubMed]
- Shimada M, Hashimoto E, Taniai M, et al. Hepatocellular carcinoma in patients with non-alcoholic steatohepatitis. J Hepatol 2002;37:154-60. [PubMed]
- Baffy G, Brunt EM, Caldwell SH. Hepatocellular carcinoma in non-alcoholic fatty liver disease: an emerging menace. J Hepatol 2012;56:1384-91. [PubMed]
- Bullock RE, Zaitoun AM, Aithal GP, et al. Association of non-alcoholic steatohepatitis without significant fibrosis with hepatocellular carcinoma. J Hepatol 2004;41:685-6. [PubMed]
- Orikasa H, Ohyama R, Tsuka N, et al. Lipid-rich clear-cell hepatocellular carcinoma arising in non-alcoholic steatohepatitis in a patient with diabetes mellitus. J Submicrosc Cytol Pathol 2001;33:195-200. [PubMed]
- Zen Y, Katayanagi K, Tsuneyama K, et al. Hepatocellular carcinoma arising in non-alcoholic steatohepatitis. Pathol Int 2001;51:127-31. [PubMed]
- Ertle J, Dechene A, Sowa JP, et al. Non-alcoholic fatty liver disease progresses to hepatocellular carcinoma in the absence of apparent cirrhosis. Int J Cancer 2011;128:2436-43. [PubMed]
- Heuer M, Kaiser GM, Kahraman A, et al. Liver transplantation in nonalcoholic steatohepatitis is associated with high mortality and post-transplant complications: a single-center experience. Digestion 2012;86:107-13. [PubMed]
- Vanwagner LB, Bhave M, Te HS, et al. Patients transplanted for nonalcoholic steatohepatitis are at increased risk for postoperative cardiovascular events. Hepatology 2012;56:1741-50. [PubMed]
- Lefebvre P, Cariou B, Lien F, et al. Role of bile acids and bile acid receptors in metabolic regulation. Physiol Rev 2009;89:147-91. [PubMed]
- Ma K, Saha PK, Chan L, et al. Farnesoid X receptor is essential for normal glucose homeostasis. J Clin Invest 2006;116:1102-9. [PubMed]
- Rizzo G, Disante M, Mencarelli A, et al. The farnesoid X receptor promotes adipocyte differentiation and regulates adipose cell function in vivo. Mol Pharmacol 2006;70:1164-73. [PubMed]
- Zhang Y, Lee FY, Barrera G, et al. Activation of the nuclear receptor FXR improves hyperglycemia and hyperlipidemia in diabetic mice. Proc Natl Acad Sci U S A 2006;103:1006-11. [PubMed]
- Cariou B, van Harmelen K, Duran-Sandoval D, et al. The farnesoid X receptor modulates adiposity and peripheral insulin sensitivity in mice. J Biol Chem 2006;281:11039-49. [PubMed]
- Coleman DL. Obese and diabetes: two mutant genes causing diabetes-obesity syndromes in mice. Diabetologia 1978;14:141-8. [PubMed]
- Leibel RL, Chung WK, Chua SC Jr. The molecular genetics of rodent single gene obesities. J Biol Chem 1997;272:31937-40. [PubMed]
- Inagaki T, Choi M, Moschetta A, et al. Fibroblast growth factor 15 functions as an enterohepatic signal to regulate bile acid homeostasis. Cell Metab 2005;2:217-25. [PubMed]
- Fu L, John LM, Adams SH, et al. Fibroblast growth factor 19 increases metabolic rate and reverses dietary and leptin-deficient diabetes. Endocrinology 2004;145:2594-603. [PubMed]
- Miyata M, Sakaida Y, Matsuzawa H, et al. Fibroblast growth factor 19 treatment ameliorates disruption of hepatic lipid metabolism in farnesoid X receptor (Fxr)-null mice. Biol Pharm Bull 2011;34:1885-9. [PubMed]
- Ip E, Farrell G, Hall P, et al. Administration of the potent PPARalpha agonist, Wy-14,643, reverses nutritional fibrosis and steatohepatitis in mice. Hepatology 2004;39:1286-96. [PubMed]
- Yamaguchi K, Yang L, McCall S, et al. Inhibiting triglyceride synthesis improves hepatic steatosis but exacerbates liver damage and fibrosis in obese mice with nonalcoholic steatohepatitis. Hepatology 2007;45:1366-74. [PubMed]
- Zhang S, Wang J, Liu Q, et al. Farnesoid X receptor agonist WAY-362450 attenuates liver inflammation and fibrosis in murine model of non-alcoholic steatohepatitis. J Hepatol 2009;51:380-8. [PubMed]
- Wu W, Liu X, Peng X, et al. Bile acids override steatosis in farnesoid X receptor deficient mice in a model of non-alcoholic steatohepatitis. Biochem Biophys Res Commun 2014;448:50-5. [PubMed]
- Bechmann LP, Kocabayoglu P, Sowa JP, et al. Free fatty acids repress small heterodimer partner (SHP) activation and adiponectin counteracts bile acid-induced liver injury in superobese patients with nonalcoholic steatohepatitis. Hepatology 2013;57:1394-406. [PubMed]
- Adorini L, Pruzanski M, Shapiro D. Farnesoid X receptor targeting to treat nonalcoholic steatohepatitis. Drug Discov Today 2012;17:988-97. [PubMed]
- Pellicciari R, Fiorucci S, Camaioni E, et al. 6alpha-ethyl-chenodeoxycholic acid (6-ECDCA), a potent and selective FXR agonist endowed with anticholestatic activity. J Med Chem 2002;45:3569-72. [PubMed]
- Pellicciari R, Costantino G, Camaioni E, et al. Bile acid derivatives as ligands of the farnesoid X receptor. Synthesis, evaluation, and structure-activity relationship of a series of body and side chain modified analogues of chenodeoxycholic acid. J Med Chem 2004;47:4559-69. [PubMed]
- Vignozzi L, Morelli A, Filippi S, et al. Farnesoid X receptor activation improves erectile function in animal models of metabolic syndrome and diabetes. J Sex Med 2011;8:57-77. [PubMed]
- Cipriani S, Mencarelli A, Palladino G, et al. FXR activation reverses insulin resistance and lipid abnormalities and protects against liver steatosis in Zucker (fa/fa) obese rats. J Lipid Res 2010;51:771-84. [PubMed]
- Durham HA, Truett GE. Development of insulin resistance and hyperphagia in Zucker fatty rats. Am J Physiol Regul Integr Comp Physiol 2006;290:R652-8. [PubMed]
- Larter CZ, Yeh MM. Animal models of NASH: getting both pathology and metabolic context right. J Gastroenterol Hepatol 2008;23:1635-48. [PubMed]
- Varela-Rey M, Embade N, Ariz U, et al. Non-alcoholic steatohepatitis and animal models: understanding the human disease. Int J Biochem Cell Biol 2009;41:969-76. [PubMed]
- Mencarelli A, Renga B, Migliorati M, et al. The bile acid sensor farnesoid X receptor is a modulator of liver immunity in a rodent model of acute hepatitis. J Immunol 2009;183:6657-66. [PubMed]
- Wang XX, Jiang T, Shen Y, et al. The farnesoid X receptor modulates renal lipid metabolism and diet-induced renal inflammation, fibrosis, and proteinuria. Am J Physiol Renal Physiol 2009;297:F1587-96. [PubMed]
- Gadaleta RM, van Erpecum KJ, Oldenburg B, et al. Farnesoid X receptor activation inhibits inflammation and preserves the intestinal barrier in inflammatory bowel disease. Gut 2011;60:463-72. [PubMed]
- Vavassori P, Mencarelli A, Renga B, et al. The bile acid receptor FXR is a modulator of intestinal innate immunity. J Immunol 2009;183:6251-61. [PubMed]
- DeFronzo RA, Tobin JD, Andres R. Glucose clamp technique: a method for quantifying insulin secretion and resistance. Am J Physiol 1979;237:E214-23. [PubMed]
- Carey EJ, Lindor KD. eds. Cholestatic liver disease. New York: Springer, 2014.
- Gershwin ME, Mackay IR, Sturgess A, et al. Identification and specificity of a cDNA encoding the 70 kd mitochondrial antigen recognized in primary biliary cirrhosis. J Immunol 1987;138:3525-31. [PubMed]
- Mattalia A, Quaranta S, Leung PS, et al. Characterization of antimitochondrial antibodies in health adults. Hepatology 1998;27:656-61. [PubMed]
- Lindor KD, Gershwin ME, Poupon R, et al. Primary biliary cirrhosis. Hepatology 2009;50:291-308. [PubMed]
- Fregeau DR, Davis PA, Danner DJ, et al. Antimitochondrial antibodies of primary biliary cirrhosis recognize dihydrolipoamide acyltransferase and inhibit enzyme function of the branched chain alpha-ketoacid dehydrogenase complex. J Immunol 1989;142:3815-20. [PubMed]
- Surh CD, Danner DJ, Ahmed A, et al. Reactivity of primary biliary cirrhosis sera with a human fetal liver cDNA clone of branched-chain alpha-keto acid dehydrogenase dihydrolipoamide acyltransferase, the 52 kD mitochondrial autoantigen. Hepatology 1989;9:63-8. [PubMed]
- Surh CD, Roche TE, Danner DJ, et al. Antimitochondrial autoantibodies in primary biliary cirrhosis recognize cross-reactive epitope(s) on protein X and dihydrolipoamide acetyltransferase of pyruvate dehydrogenase complex. Hepatology 1989;10:127-33. [PubMed]
- Fussey SP, Ali ST, Guest JR, et al. Reactivity of primary biliary cirrhosis sera with Escherichia coli dihydrolipoamide acetyltransferase (E2p): characterization of the main immunogenic region. Proc Natl Acad Sci U S A 1990;87:3987-91. [PubMed]
- Parikh-Patel A, Gold EB, Worman H, et al. Risk factors for primary biliary cirrhosis in a cohort of patients from the united states. Hepatology 2001;33:16-21. [PubMed]
- Gershwin ME, Selmi C, Worman HJ, et al. Risk factors and comorbidities in primary biliary cirrhosis: a controlled interview-based study of 1032 patients. Hepatology 2005;42:1194-202. [PubMed]
- Prince MI, Ducker SJ, James OF. Case-control studies of risk factors for primary biliary cirrhosis in two United Kingdom populations. Gut 2010;59:508-12. [PubMed]
- Hirschfield GM, Liu X, Xu C, et al. Primary biliary cirrhosis associated with HLA, IL12A, and IL12RB2 variants. N Engl J Med 2009;360:2544-55. [PubMed]
- Hirschfield GM, Liu X, Han Y, et al. Variants at IRF5-TNPO3, 17q12-21 and MMEL1 are associated with primary biliary cirrhosis. Nat Genet 2010;42:655-7. [PubMed]
- Liu X, Invernizzi P, Lu Y, et al. Genome-wide meta-analyses identify three loci associated with primary biliary cirrhosis. Nat Genet 2010;42:658-60. [PubMed]
- Hirschfield GM, Invernizzi P. Progress in the genetics of primary biliary cirrhosis. Semin Liver Dis 2011;31:147-56. [PubMed]
- Mells GF, Floyd JA, Morley KI, et al. Genome-wide association study identifies 12 new susceptibility loci for primary biliary cirrhosis. Nat Genet 2011;43:329-32. [PubMed]
- Hirschfield GM, Gershwin ME. The immunobiology and pathophysiology of primary biliary cirrhosis. Annu Rev Pathol 2013;8:303-30. [PubMed]
- Eriksson S, Lindgren S. The prevalence and clinical spectrum of primary biliary cirrhosis in a defined population. Scand J Gastroenterol 1984;19:971-6. [PubMed]
- Löfgren J, Järnerot G, Danielsson D, et al. Incidence and prevalence of primary biliary cirrhosis in a defined population in Sweden. Scand J Gastroenterol 1985;20:647-50. [PubMed]
- Remmel T, Remmel H, Uibo R, et al. Primary biliary cirrhosis in Estonia. With special reference to incidence, prevalence, clinical features, and outcome. Scand J Gastroenterol 1995;30:367-71. [PubMed]
- Sood S, Gow PJ, Christie JM, et al. Epidemiology of primary biliary cirrhosis in Victoria, Australia: high prevalence in migrant populations. Gastroenterology 2004;127:470-5. [PubMed]
- Rautiainen H, Salomaa V, Niemela S, et al. Prevalence and incidence of primary biliary cirrhosis are increasing in Finland. Scand J Gastroenterol 2007;42:1347-53. [PubMed]
- Boonstra K, Kunst AE, Stadhouders PH, et al. Rising incidence and prevalence of primary biliary cirrhosis: a large population-based study. Liver Int 2014;34:e31-8. [PubMed]
- Talwalkar JA, Lindor KD. Primary biliary cirrhosis. Lancet 2003;362:53-61. [PubMed]
- Newton JL. Fatigue in primary biliary cirrhosis. Clin Liver Dis 2008;12:367-83. [PubMed]
- Jones DE, Al-Rifai A, Frith J, et al. The independent effects of fatigue and UDCA therapy on mortality in primary biliary cirrhosis: results of a 9 year follow-up. J Hepatol 2010;53:911-7. [PubMed]
- Jones DE, Bhala N, Burt J, et al. Four year follow up of fatigue in a geographically defined primary biliary cirrhosis patient cohort. Gut 2006;55:536-41. [PubMed]
- Jones EA, Bergasa NV. The pathogenesis and treatment of pruritus and fatigue in patients with PBC. Eur J Gastroenterol Hepatol 1999;11:623-31. [PubMed]
- Newton JL, Gibson GJ, Tomlinson M, et al. Fatigue in primary biliary cirrhosis is associated with excessive daytime somnolence. Hepatology 2006;44:91-8. [PubMed]
- Quarneti C, Muratori P, Lalanne C, et al. Fatigue and pruritus at onset identify a more aggressive subset of primary biliary cirrhosis. Liver Int 2014. [Epub ahead of print]. [PubMed]
- Stanca CM, Bach N, Krause C, et al. Evaluation of fatigue in U.S. patients with primary biliary cirrhosis. Am J Gastroenterol 2005;100:1104-9. [PubMed]
- Theal JJ, Toosi MN, Girlan L, et al. A randomized, controlled crossover trial of ondansetron in patients with primary biliary cirrhosis and fatigue. Hepatology 2005;41:1305-12. [PubMed]
- Zein CO, McCullough AJ. Association between fatigue and decreased survival in primary biliary cirrhosis. Gut 2007;56:1165-6; author reply 1166. [PubMed]
- Carbone M, Bufton S, Monaco A, et al. The effect of liver transplantation on fatigue in patients with primary biliary cirrhosis: a prospective study. J Hepatol 2013;59:490-4. [PubMed]
- Beuers U, Kremer AE, Bolier R, et al. Pruritus in cholestasis: facts and fiction. Hepatology 2014;60:399-407. [PubMed]
- Kremer AE, Martens JJ, Kulik W, et al. Lysophosphatidic acid is a potential mediator of cholestatic pruritus. Gastroenterology 2010;139:1008-18. [PubMed]
- Imam MH, Lindor KD. The natural history of primary biliary cirrhosis. Semin Liver Dis 2014;34:329-33. [PubMed]
- Neuberger J. Liver transplantation for primary biliary cirrhosis: indications and risk of recurrence. J Hepatol 2003;39:142-8. [PubMed]
- Angulo P, Batts KP, Therneau TM, et al. Long-term ursodeoxycholic acid delays histological progression in primary biliary cirrhosis. Hepatology 1999;29:644-7. [PubMed]
- Corpechot C, Carrat F, Bonnand AM, et al. The effect of ursodeoxycholic acid therapy on liver fibrosis progression in primary biliary cirrhosis. Hepatology 2000;32:1196-9. [PubMed]
- Lindor KD, Jorgensen RA, Therneau TM, et al. Ursodeoxycholic acid delays the onset of esophageal varices in primary biliary cirrhosis. Mayo Clin Proc 1997;72:1137-40. [PubMed]
- Lindor KD, Therneau TM, Jorgensen RA, et al. Effects of ursodeoxycholic acid on survival in patients with primary biliary cirrhosis. Gastroenterology 1996;110:1515-8. [PubMed]
- Poupon RE, Balkau B, Eschwege E, et al. A multicenter, controlled trial of ursodiol for the treatment of primary biliary cirrhosis. UDCA-PBC Study Group. N Engl J Med 1991;324:1548-54. [PubMed]
- Poupon RE, Lindor KD, Cauch-Dudek K, et al. Combined analysis of randomized controlled trials of ursodeoxycholic acid in primary biliary cirrhosis. Gastroenterology 1997;113:884-90. [PubMed]
- Poupon RE, Lindor KD, Pares A, et al. Combined analysis of the effect of treatment with ursodeoxycholic acid on histologic progression in primary biliary cirrhosis. J Hepatol 2003;39:12-6. [PubMed]
- Bowlus CL, Gershwin ME. The diagnosis of primary biliary cirrhosis. Autoimmun Rev 2014;13:441-4. [PubMed]
- Lammers WJ, van Buuren HR, Hirschfield GM, et al. Levels of Alkaline Phosphatase and Bilirubin Are Surrogate End Points of Outcomes of Patients With Primary Biliary Cirrhosis: An International Follow-up Study. Gastroenterology 2014;147:1338-49. [PubMed]
- Eaton JE, Talwalkar JA, Lazaridis KN, et al. Pathogenesis of primary sclerosing cholangitis and advances in diagnosis and management. Gastroenterology 2013;145:521-36. [PubMed]
- Chapman R, Fevery J, Kalloo A, et al. Diagnosis and management of primary sclerosing cholangitis. Hepatology 2010;51:660-78. [PubMed]
- Björnsson E, Olsson R, Bergquist A, et al. The natural history of small-duct primary sclerosing cholangitis. Gastroenterology 2008;134:975-80. [PubMed]
- Miloh T, Arnon R, Shneider B, et al. A retrospective single-center review of primary sclerosing cholangitis in children. Clin Gastroenterol Hepatol 2009;7:239-45. [PubMed]
- Olsson R, Glaumann H, Almer S, et al. High prevalence of small duct primary sclerosing cholangitis among patients with overlapping autoimmune hepatitis and primary sclerosing cholangitis. Eur J Intern Med 2009;20:190-6. [PubMed]
- Bambha K, Kim WR, Talwalkar J, et al. Incidence, clinical spectrum, and outcomes of primary sclerosing cholangitis in a United States community. Gastroenterology 2003;125:1364-9. [PubMed]
- Lindor KD, Kowdley KV, Luketic VA, et al. High-dose ursodeoxycholic acid for the treatment of primary sclerosing cholangitis. Hepatology 2009;50:808-14. [PubMed]
- Sinakos E, Marschall HU, Kowdley KV, et al. Bile acid changes after high-dose ursodeoxycholic acid treatment in primary sclerosing cholangitis: Relation to disease progression. Hepatology 2010;52:197-203. [PubMed]
- Broomé U, Olsson R, Lööf L, et al. Natural history and prognostic factors in 305 Swedish patients with primary sclerosing cholangitis. Gut 1996;38:610-5. [PubMed]
- Farrant JM, Hayllar KM, Wilkinson ML, et al. Natural history and prognostic variables in primary sclerosing cholangitis. Gastroenterology 1991;100:1710-7. [PubMed]
- Ponsioen CY, Vrouenraets SM, Prawirodirdjo W, et al. Natural history of primary sclerosing cholangitis and prognostic value of cholangiography in a Dutch population. Gut 2002;51:562-6. [PubMed]
- Wiesner RH, Grambsch PM, Dickson ER, et al. Primary sclerosing cholangitis: natural history, prognostic factors and survival analysis. Hepatology 1989;10:430-6. [PubMed]
- Razumilava N, Gores GJ. Classification, diagnosis, and management of cholangiocarcinoma. Clin Gastroenterol Hepatol 2013;11:13-21. [PubMed]
- Razumilava N, Gores GJ, Lindor KD. Cancer surveillance in patients with primary sclerosing cholangitis. Hepatology 2011;54:1842-52. [PubMed]
- Rizvi S, Gores GJ. Pathogenesis, diagnosis, and management of cholangiocarcinoma. Gastroenterology 2013;145:1215-29. [PubMed]
- Ali A, Carey EJ, Lindor KD. An Overview of Current and Future Strategies for the Treatment of Primary Sclerosing Cholangitis. Expert Opinion on Orphan Drugs 2014;2:545-56.
- Olsson R, Boberg KM, de Muckadell OS, et al. High-dose ursodeoxycholic acid in primary sclerosing cholangitis: a 5-year multicenter, randomized, controlled study. Gastroenterology 2005;129:1464-72. [PubMed]
- Imam MH, Sinakos E, Gossard AA, et al. High-dose ursodeoxycholic acid increases risk of adverse outcomes in patients with early stage primary sclerosing cholangitis. Aliment Pharmacol Ther 2011;34:1185-92. [PubMed]
- Bjøro K, Brandsaeter B, Foss A, et al. Liver transplantation in primary sclerosing cholangitis. Semin Liver Dis 2006;26:69-79. [PubMed]
- Graziadei IW. Live donor liver transplantation for primary sclerosing cholangitis: is disease recurrence increased? Curr Opin Gastroenterol 2011;27:301-5. [PubMed]
- Mason A, Luketic V, Lindor K, et al. Farnesoid-x receptor agonists: a new class of drugs for the treatment of PBC? An international study evaluating the addition of INT-747 to ursodeoxycholic acid. J Hepatol 2010;52:S1-2.
- Kowdley KV, Jones D, Luketic V, et al. An international study evaluating the farnesoid X receptor agonist obeticholic acid as monotherapy in PBC. J Hepatol 2011;54:S13.