Cyclin-dependent kinase 5 contributes to apoptosis of vascular endothelial cells during aortic dissection
Introduction
Aortic dissection (AD) starts primarily from a tear in the intimal layer of the aorta or from a bleeding plot within the aortic wall (1). The high blood pressure inside the aortic vessels continuously damage and enlarge the tear, leading to the split of the inner and middle layers of the aorta, which becomes lethal when the outside aortic wall is broken (2). AD typically occurs in men over 70 years old and has many obvious symptoms, such as acute chest pain and back pain (3). Early diagnosis of AD in high risk population, such as patients with Marfan syndrome, enlarged aortic aneurysms, arterial hypertension and people who have family history of ADs with prompt treatment can significantly increase the chance of survival (4). A comprehensive understanding of the molecular regulation of AD can significantly improve the early diagnosis rate, but little is known about this molecular regulation.
Cyclin-dependent kinase 5 (CDK5) was initially detected in the brain as a proline-directed serine/threonine protein kinase regulating tau hyperphosphorylation (5). CDK5 is known to be activated at an equal potential by p35 and its truncated form, p25 (6). Because p25 binds to CDK5 in a more sustained manner than p35 (7), activation of CDK5 is increased by p25 binding, causing pathological processes (8). CD5 also regulates neuronal cell cycle re-entry and arrest through its downstream regulated genes, proliferating cell nuclear antigen (PCNA) (9), cyclin B1 (CCNB1) (10,11), and B-cell lymphoma 2 (Bcl-2) (12). The abnormal expression of CDK5 leads to apoptotic cell death following abortive cell cycle re-entry in some neuronal diseases (13-17). Although physiological and pathological roles of CDK5 have been widely investigated (18,19), the expression and function of CDK5 in AD have not been reported. Therefore, the aim of the present study was to address these. We present the following article in accordance with the MDAR reporting checklist (available at https://atm.amegroups.com/article/view/10.21037/atm-22-3777/rc).
Methods
Protocol and specimens
The study was conducted in accordance with the Declaration of Helsinki (as revised in 2013). The study was approved by the ethics board of Wenzhou Medical University (No. KY2022-091) and informed consent was taken from all the patients. Patient AD specimens were collected in Wenzhou Medical University. Normal aortic tissues were obtained from materials discarded from aortic bypass. None of the included human specimens were from patients with related diseases.
Fluorescence-activated cell sorting (FACS)
Human AD specimens or normal aortic tissue were digested with 0.2% trypsin and 0.1% DNase in phosphate-buffered saline solution for 30 minutes at 37 ℃. Dissociated cells were incubated with allophycocyanin (APC)-conjugated CD31 antibody (Becton-Dickinson Biosciences, San Jose, CA, USA), Cy3-conjugated α-smooth muscle actin antibody (α-SMA) after fixation of the cells (Becton-Dickinson Biosciences, USA), Cy5-conjugated CD45 antibody (Becton-Dickinson Biosciences, USA), or Cy2-conjugated N-cadherin (N-cad) antibody (Becton-Dickinson Biosciences, USA) for 15 minutes, followed by FACS. Data were analyzed and presented using Flowjo software (Flowjo LLC, Ashland, OR, USA).
Cell Counting Kit-8 (CCK-8) assay
CCK-8 assay was performed using a CCK-8 kit (Abcam, Shanghai, China) following instructions.
Plasmids
siRNA for CDK5 (si-CDK5), scrambled sequence control (scr), and CDK5 plasmids were all purchased from Clontech (Mountain View, CA, USA). Transfection was done using Lipofectamine 3000 (Invitrogen, St Louis, MO, USA).
Quantitative reverse transcription polymerase chain reaction (qRT-PCR)
The RNeasy kit (Qiagen, Beijing, China) was used for total RNA isolation to provide complementary DNA (cDNA) for qRT-PCR using a QuantiTect SYBR Green PCR kit (Qiagen, China). All primers were Qiagen-derived commercial primers. The 2-∆∆CT method was used for the analysis, and relative expression values were calculated.
Immunocytochemistry
Immunocytochemistry and terminal deoxynucleotidyl transferase-mediated digoxigenin-dUTP nick-end labeling (TUNEL) assay staining were done using an immunocytochemistry staining kit (Dako, Carpinteria, CA, USA) and a Cy5-TUNEL staining kit (R&D Systems, Beijing, China), respectively. The rabbit anti-CDK5 antibody was purchased from Abcam (Shanghai, China).
Statistical analysis
Unpaired 2-tailed Student’s t-test was used for comparison values from two groups with GraphPad Prism software (GraphPad Software, La Jolla, CA, USA). Data were represented as individual values, and significance was considered when P<0.05. No data was excluded for analysis.
Results
Analysis of GEO database that reported gene profiles from AD tissues and normal aortic specimens
To determine the genes that play important roles in AD, we first explored the published database at the Gene Expression Omnibus (GEO) website (https://www.ncbi.nlm.nih.gov/geo/) and determined that GSE52093 was a suitable resource to study. This database reported gene expression profiles from AD tissues (n=7, shown as “ad”) and normal aortic tissues (n=5, shown as “ctl”). Using bioinformatic tools, we found that the samples were good quality, as shown in Figure 1A. An expression density analysis is shown in Figure 1B, a moderated t-statistic test is shown in Figure 1C, and a mean variance trend test is shown in Figure 1D. Moreover, adjusted P values were obtained (Figure 1E). Principal components analysis plot exhibited good and separated distribution of two groups (Figure 1F), which confirmed that the data in this database were suitable for analysis. A total of 1,423 differentiated genes with an adjusted P value <0.05 were detected between the two groups (Figure 1G), shown in two types of volcano maps (Figure 1H,1I). Of note, we detected CDK5 as a downregulated gene in AD specimens (Figure 1I).
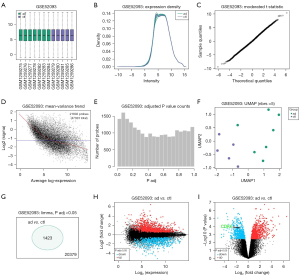
CDK5 levels increase in AD specimens compared with controls, and its regulated genes alter correspondingly
Because CDK5 is known for cell cycle control and apoptosis, which are related pathological events during the progression of AD, we further examined the genes downstream of CDK5. Interestingly, not only did we find significant increases in CDK5 in AD specimens compared with controls (Figure 2A), we also found that 3 CDK5-regulated genes significantly altered. Among them, 2 cell cycle regulators, PCNA and CCNB1, significantly increased (Figure 2B,2C), while anti-apoptotic protein, Bcl-2, significantly decreased (Figure 2D). Interestingly, when we used GSE52093 data for the correlation analysis, we detected a strong and significant correlation between CDK5 and either of its downstream factors, PCNA (positive correlation, r=0.83, P=0.0008) (Figure 2E), CCNB1 (positive correlation, r=0.81, P=0.0013) (Figure 2F), and Bcl-2 (inverse correlation, r=–0.86, P=0.0004) (Figure 2G). These data suggest that the alteration in the levels of PCNA, CCNB1, and Bcl-2 is likely due to changes in CDK5 levels.
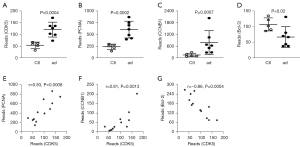
CDK5 is mainly expressed in aortic endothelial cells (AECs), and increases significantly in AD
Because data in GSE52093 was from total aortic tissue, it was unknown which cells were the major source of CDK5. It is important to develop targeted therapy; therefore, we used FACS to isolate AECs based on their unique expression of CD31, mesenchymal cells based on their expression of α-SMA, inflammatory cells based on their expression of CD45, and myocardial cells based on their expression of N-cad (Figure 3A). To confirm the quality and the proper selection of cell surface markers for FACS, purified CD31+ cells, α-SMA+ cells, CD45+ cells, and N-cad+ cells were subjected to qRT-PCR analysis for CD31, α-SMA, CD45 and N-cad. Cardiac troponin T (cTnT) was used as an additional marker for myocardial cells to increase specificity. We found that CD31 was nearly exclusively expressed by CD31+ cells, and not expressed by α-SMA+ cells, CD45+ cells, and N-cad+ cells. α-SMA was nearly exclusively expressed by α-SMA+ cells, and not expressed by CD31+ cells, CD45+ cells, and N-cad+ cells. CD45 was nearly exclusively expressed by CD45+ cells, and not expressed by α-SMA+ cells, CD31+ cells, and N-cad+ cells. N-cad was nearly exclusively expressed by N-cad+ or cTnT+ cells, and not expressed by α-SMA+ cells, CD45+ cells, and CD31+ cells (Figure 3B). These data confirm the quality and specificity of the FACS. Interestingly, CDK5 was highly expressed in CD31+ cells, but not in other cell types (Figure 3B). Next, we compared CD31+ cells in AD and normal aortic specimens. We detected significant differences to higher degrees in all 3 CDK5-regulated genes from AD than that from normal aortic specimens (Figure 3C), which suggests that CDK5 is mainly expressed in AECs, and increases significantly in AD.
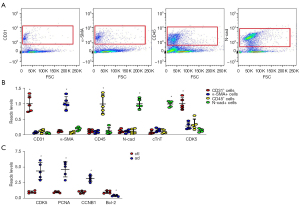
Alteration of CDK5 levels in AECs
To assess whether increases in CDK5 levels contribute to apoptotic cell death in AECs, we generated siRNA for CDK5 (si-CDK5), the CDK5-coding sequence (CDK5), and a scr to be inserted into plasmids. AECs were transfected with these plasmids, after which the CDK5 was determined by immunocytochemistry. We found that transfection with si-CDK5 significantly reduced CDK5 expression in AECs, while transfection with CDK5 significantly increased CDK5 expression in AECs compared with scr-transfected AECs, as shown by representative images (Figure 4A) and by quantification (Figure 4B).
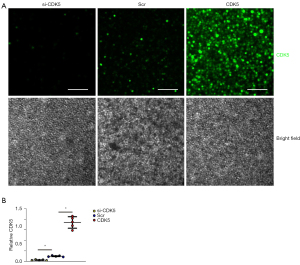
CDK5 induces apoptosis in AECs
Effects of CDK5 on cell growth and apoptosis of AECs were determined. CCK-8 assay found that alteration in CDK5 did not change the total survival AECs in 24 hours (Figure 5A). However, the total surviving cell number significantly decreased at 48 hours by overexpression of CDK5 (Figure 5A). In a TUNEL assay, transfection with si-CDK5 significantly reduced TUNEL+ apoptotic AECs, while transfection with CDK5 significantly increased TUNEL+ apoptotic AECs compared with scr-transfected AECs, as shown by quantification (Figure 5B) and by representative images (Figure 5C). Together, these data suggest that CDK5 induces apoptosis in AECs.
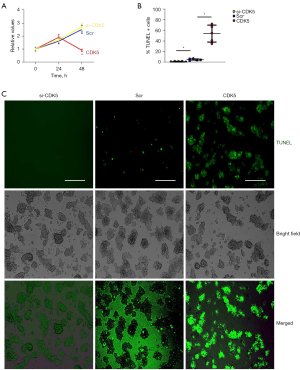
Discussion
CDK5 plays an important role in controlling the hyperphosphorylation of the tau protein, and this contributes to the development of neurodegenerative diseases (14). This role of CDK5 was further demonstrated in a diabetic status (20), likely due to the fact that CDK5 regulates neuronal cell cycle re-entry and arrest through its downstream regulated genes, PCNA (9), CCNB1 (10,11) and Bcl-2 (12). During a live cell cycle, PCNA acts as a clamp, which is necessary for DNA polymerase to work properly on the DNA. PCNA expresses throughout the active cell cycle, but reaches its peak at the S phase. CCNB1 is a key regulator in G2/M phase transition in the cell cycle. CDK5 activates both PCNA and CCNB1 to help the cell to proliferate. However, many published studies have indicated that the abnormal expression of CDK5 could lead to apoptotic cell death following cell cycle re-entry in some neuronal diseases (13-17). Therefore, the suppressive effects of CDK5 on Bcl-2 could play a more important role in the AECs during AD, which support our findings of the total number of CDK5-overexpressed AECs growing similarly to the control AECs at the first 24 hours, but decreasing significantly at 48 hours. This was likely due to the positive effects of CDK5 on cell cycle activation through PCNA and CCNB1 were ablated by apoptosis induction through Bcl-2.
The increased expression of CDK5 by AD compared with normal aortic tissue was more obvious in our study than in GSE52093. This result stemmed from the difference in the analyzed targets, as they examined total aortic tissues, but we examined purified CD31+ endothelial cells. We found that AECs are the predominant source of CDK5 in the aortic tissue; therefore, it is not surprising that the degree of the augmentation of CDK5 in our study was much higher than in GSE52093. Changes in AEC-derived CDK5 in their study were diluted in the mixed tissue.
To the best of our knowledge, the present study is the first to demonstrate the role of CDK5 in AD; however, it has some limitations. First, we used human specimens from a public database and from our own resources for the analysis, and performed in vitro studies using primary AECs. Future studies should include in vivo animal models. Second, the analyzed database was relatively small and recruited samples from only one region. More samples from different areas will be needed to confirm findings in this study.
Conclusions
CDK5 induces apoptosis of AECs to promote AD. CDK5 appears to be a promising novel target for preventing AD.
Acknowledgments
The authors appreciate the academic support from the AME Vascular Surgery Collaborative Group.
Funding: This work was supported by a grant from Scientific Research Incubator Project of the First Affiliated Hospital of Wenzhou Medical University (No. FHY2019030).
Footnote
Reporting Checklist: The authors have completed the MDAR reporting checklist. Available at https://atm.amegroups.com/article/view/10.21037/atm-22-3777/rc
Data Sharing Statement: Available at https://atm.amegroups.com/article/view/10.21037/atm-22-3777/dss
Conflicts of Interest: All authors have completed the ICMJE uniform disclosure form (available at https://atm.amegroups.com/article/view/10.21037/atm-22-3777/coif). The authors have no conflicts of interest to declare.
Ethical Statement: The authors are accountable for all aspects of the work in ensuring that questions related to the accuracy or integrity of any part of the work are appropriately investigated and resolved. The study was conducted in accordance with the Declaration of Helsinki (as revised in 2013). The study was approved by the ethics board of Wenzhou Medical University (No. KY2022-091) and informed consent was taken from all the patients.
Open Access Statement: This is an Open Access article distributed in accordance with the Creative Commons Attribution-NonCommercial-NoDerivs 4.0 International License (CC BY-NC-ND 4.0), which permits the non-commercial replication and distribution of the article with the strict proviso that no changes or edits are made and the original work is properly cited (including links to both the formal publication through the relevant DOI and the license). See: https://creativecommons.org/licenses/by-nc-nd/4.0/.
References
- Chakraborty A, Li Y, Zhang C, et al. Programmed cell death in aortic aneurysm and dissection: A potential therapeutic target. J Mol Cell Cardiol 2022;163:67-80. [Crossref] [PubMed]
- Juraszek A, Czerny M, Rylski B. Update in aortic dissection. Trends Cardiovasc Med 2021; Epub ahead of print. [Crossref] [PubMed]
- Sen I, Erben YM, Franco-Mesa C, et al. Epidemiology of aortic dissection. Semin Vasc Surg 2021;34:10-7. [Crossref] [PubMed]
- Sherk WM, Khaja MS, Williams DM. Anatomy, Pathology, and Classification of Aortic Dissection. Tech Vasc Interv Radiol 2021;24:100746. [Crossref] [PubMed]
- Dhavan R, Tsai LH. A decade of CDK5. Nat Rev Mol Cell Biol 2001;2:749-59. [Crossref] [PubMed]
- Pao PC, Tsai LH. Three decades of Cdk5. J Biomed Sci 2021;28:79. [Crossref] [PubMed]
- Toro-Fernández LF, Zuluaga-Monares JC, Saldarriaga-Cartagena AM, et al. Targeting CDK5 in Astrocytes Promotes Calcium Homeostasis Under Excitotoxic Conditions. Front Cell Neurosci 2021;15:643717. [Crossref] [PubMed]
- Posada-Duque RA, Cardona-Gómez GP. CDK5 Targeting as a Therapy for Recovering Neurovascular Unit Integrity in Alzheimer's Disease. J Alzheimers Dis 2021;82:S141-61. [Crossref] [PubMed]
- Chao AC, Chen CH, Wu MH, et al. Roles of Id1/HIF-1 and CDK5/HIF-1 in cell cycle reentry induced by amyloid-beta peptide in post-mitotic cortical neuron. Biochim Biophys Acta Mol Cell Res 2020;1867:118628. [Crossref] [PubMed]
- Yan H, Xu JJ, Ali I, et al. CDK5RAP3, an essential regulator of checkpoint, interacts with RPL26 and maintains the stability of cell growth. Cell Prolif 2022;55:e13240. [Crossref] [PubMed]
- Maestre C, Delgado-Esteban M, Gomez-Sanchez JC, et al. Cdk5 phosphorylates Cdh1 and modulates cyclin B1 stability in excitotoxicity. EMBO J 2008;27:2736-45. [Crossref] [PubMed]
- Liu H, Ho PW, Leung CT, et al. Aberrant mitochondrial morphology and function associated with impaired mitophagy and DNM1L-MAPK/ERK signaling are found in aged mutant Parkinsonian LRRK2R1441G mice. Autophagy 2021;17:3196-220. [Crossref] [PubMed]
- Chen C, Peng X, Tang J, et al. CDK5 inhibition protects against OGDR induced mitochondrial fragmentation and apoptosis through regulation of Drp1S616 phosphorylation. Life Sci 2021;269:119062. [Crossref] [PubMed]
- Zhuang J, Chen Z, Cai P, et al. Targeting MicroRNA-125b Promotes Neurite Outgrowth but Represses Cell Apoptosis and Inflammation via Blocking PTGS2 and CDK5 in a FOXQ1-Dependent Way in Alzheimer Disease. Front Cell Neurosci 2020;14:587747. [Crossref] [PubMed]
- Rong R, Xia X, Peng H, et al. Cdk5-mediated Drp1 phosphorylation drives mitochondrial defects and neuronal apoptosis in radiation-induced optic neuropathy. Cell Death Dis 2020;11:720. [Crossref] [PubMed]
- Roufayel R, Murshid N. CDK5: Key Regulator of Apoptosis and Cell Survival. Biomedicines 2019;7:88. [Crossref] [PubMed]
- Zhou T, Wang H, Shen J, et al. The p35/CDK5 signaling is regulated by p75NTR in neuronal apoptosis after intracerebral hemorrhage. J Cell Physiol 2019; Epub ahead of print. [Crossref] [PubMed]
- Do PA, Lee CH. The Role of CDK5 in Tumours and Tumour Microenvironments. Cancers (Basel) 2020;13:101. [Crossref] [PubMed]
- Sharma S, Sicinski P. A kinase of many talents: non-neuronal functions of CDK5 in development and disease. Open Biol 2020;10:190287. [Crossref] [PubMed]
- Cai HB, Fan ZZ, Tian T, et al. Epigenetic Control of CDK5 Promoter Regulates Diabetes-Associated Development of Alzheimer's Disease. J Alzheimers Dis 2019;69:743-50. [Crossref] [PubMed]
(English Language Editor: R. Scott)