Patient-specific airway stent using three-dimensional printing: a review
Introduction
Numerous diseases, malignant and benign, are known to affect the airway (1). Bronchoscopy plays an essential role in diagnosing and managing these conditions (2). Bronchoscopic treatment aims to improve a patient’s quality of life, alleviate symptoms, and deliver significant palliation after non-invasive treatments are deemed ineffective (1,3). Flexible or rigid bronchoscopy (RB) can provide several treatment modalities, such as laser therapy, cryotherapy, electrosurgery, argon plasma coagulation, photodynamic therapy, and stent placement (1,3-6).
The primary function of an airway stent is to reestablish patency, impeding restenosis, supporting the tracheobronchial wall, or occluding fistulas (7,8). There are various types of stents available on the market. They may consist of silicone, metallic wire mesh, or a combination of these materials (hybrid) (9). They also have different shapes (Straight, Y-shaped, or T-tubes), diameters, and lengths (9). Complications such as migration, granulation, infection, and mucus plugging are common (7,10-13). Therefore, stent placement should be temporary when no other techniques achieve adequate and sustained patency (5,14-16).
Benign obstructions require caution since implantation may be long-term, potentially producing a high rate of complications (14). Uncovered metallic stents are contraindicated for use in some benign diseases due to the risk of excessive granulation tissue formation that worsens airway obstruction (14). Furthermore, their adherence to the airway wall can result in significant airway damage when removed (14). As a result of complications associated with metallic stents, silicone stents are the most used for benign diseases (6,14,17). Silicone stents are made of synthetic material that elicits minimal tissue reactivity and can be easily removed (14,16).
Unfortunately, commercially available stents (CAS) only exist in limited shapes and sizes. Occasionally, it is necessary to customize them to improve fit and functionality, especially in patients with complex airway anatomy (18). This customization is usually done by cutting and sewing stents together in the procedure room and requires an experienced and highly skilled physician (18). Additionally, the procedural time needed to create the customized silicone stent may prolong a procedure, which could be detrimental to the patient (18).
The complex characteristics of tracheobronchial anatomy make the three-dimensional (3D)-printing technology ideal for developing airway prostheses to treat complex diseases. Through years of investigation and advancement, it is now feasible to manufacture a patient-specific stent using this technology (19-22). A patient-specific stent may help minimize complications, decrease procedure time, and improve patients’ quality of life while reducing symptoms and the need for repeated procedures.
3D technology
Hull patented in 1986 “an apparatus for production of three-dimensional objects by stereolithography” this method uses a computer-aided design (CAD) and produces 3D objects formed by thin layers of a ultraviolet (UV) curable material (23). Then, in 1989, Deckard patented an “apparatus and method for producing parts by selective laser sintering” that uses a computer-controlled laser to direct the energy onto a powder to produce a sintered mass (24). Crump created the “Fused deposition modeling”, which directly extrudes the supply material or filament from a heated nozzle and produces the 3D model (25). Later, Sachs and colleagues patented in 1993 “Three-dimensional printing techniques”, coining the name for 3D printing (23,26). They, among others, made significant steps in additive manufacturing using 3D printing technology, which is a process of building a physical object using modeling data that is fast, precise, and fully customizable (26,27). The process of creating 3D objects is based on the design of a virtual 3D model, the data obtained from slicing this CAD 3D model is transferred to computer-controlled equipment that deposits material layer by layer until the item is produced. Currently, several printing technologies exist, each one offers different methods and uses various printing materials like elastomers, plastic, or metals (28). After several years of development, 3D printing was adopted in various industries, and healthcare is one important using this technology.
In the beginning, 3D printing was used exclusively for prototyping, but now it offers transformative advantages and several applications such as prostheses, implants, medical models, and medical devices. Also, this technology is rapidly evolving in biomedical engineering by creating a relatively new process called bioprinting that incorporates cells directly into a printed tissue (29). Now, healthcare 3D printing is leading a revolution in personalized medicine, making customized objects or tools that fit specific patients’ needs (28).
3D printing technology and airway disease
One of the initial uses of rapid prototyping techniques in medicine was the anatomical modeling of different body structures that helps surgeons in preoperative planning (30,31).
3D printing technology has been used in respiratory medicine, especially in central airway diseases. In 2013 Tam et al. printed 3D models of the tracheobronchial tree of a patient with airway disease secondary to relapsing polychondritis, they used inspiratory and expiratory models and discussed the potential aid for surgical or interventional planning and education (32).
Zopf and colleagues published in 2013 a case where a bioresorbable airway splint was created using 3D printing technology and surgically tied in a malacic left main bronchus of a newborn (33).
Then, in 2015, Cheng et al. published the first reported case of a 3D modeled T-tube placed in the complex upper airway of a patient, this prosthesis was designed to obtain a 3D reconstruction of the trachea from a computed tomography (CT) scan (34).
Thomas R. Gildea, in February 2016, using CT imaging and 3D printing technology, made and implanted the first bronchial patient-specific airway stent (PSS) made out of silicone under FDA clearance for compassionate use to manage a 56-year-old male patient with airway complications of granulomatosis with polyangiitis who required multiple unsuccessful therapeutic bronchoscopies and several commercially and manually customized stents (20,22). In 2017, Gildea and colleagues reported the one-year experience of this and another patient with complex airway disease secondary to granulomatosis with polyangiitis (GPA); after insertion of PSS produced using 3D printing technology, both patients increased average time between procedures and stent life after implantation (20,22).
The same year, Guibert et al. published a case of a patient with an airway complication after lung transplantation, the right airway had dehiscence, a stenotic bronchus intermedius, and complex anatomy. A 3D airway was created from a CT scan, the complications were virtually resolved, a designed 3D mold was printed and used to make a custom stent that was placed successfully with RB (35). Similar efforts have been made in adult patients treating tracheobronchomalacia with 3D printing technology (36). Recently, Shan and colleagues have published their experiences treating aerodigestive fistula and malignant airway obstruction with hybrid stents customized with the assistance of 3D printing (37,38).
3D printing methods and materials in airway stents
Different 3D methods and materials have been used to create airway stents.
Cheng and colleagues used 3D slicer (a free, open-source, and multi-platform software package widely used for medical, biomedical, and related imaging research) to obtain a 3D reconstruction of the trachea using a CT scan. Then, this 3D model was imported to Solidworks® (CAD software), and a virtual T-tube was designed that matched his patient’s virtual complex upper airway. After the virtual design was approved, it was sent to a manufacturer, and a silicone personalized T-tube was produced and inserted through the tracheostomy stoma under bronchoscopy guidance (19,34).
Gildea and colleagues imported the CT scan digital imaging into a proprietary software developed for orthopedic surgery (COS Inc., Cleveland, OH, USA). A 3D virtual prototype of the airway is created. Based on this virtual model of each patient’s anatomy, the desired stent dimensions were defined, including the area, diameter, angulation, branching, length, and wall thickness. The physician uses the software tools to place a series of spheres in the desired area to adjust the shapes and sizes to make a virtual representation based on clinical needs. A mold of the prescribed stent is produced with 3D printing technology, and the stent is manufactured by injecting medical-grade silicone into this mold. The stent is cleaned and finished producing a smooth surface, and external studs are added. The stent is sterilized with standard steam sterilization on site. Finally, the stent is placed with standards methods and tools using RB (20,36) (Figures 1-3).
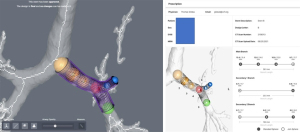
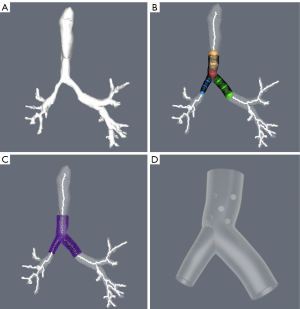
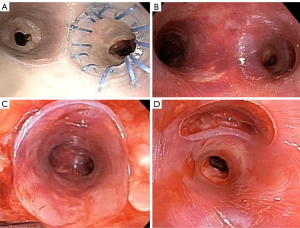
Guibert used a similar workflow, designing a virtual 3D mold (VGStudio MAX software). The 3D data was imported to the 3D printing (RolandDG MDX 40A) to produce an Ertacetal POM mold. A personalized silicone stent was made from this mold. Therapeutic RB is performed, and the stent is inserted (35,39).
Shan and colleagues used data from a 64-slice multidetector spiral CT scan to reconstruct 3D images of the airway using CAD software (Vitaworks, Shanghai, China). The airway and tumor were then assigned different colors, and the image was converted into a 3D stereolithographic (STL) file. The 3D reconstruction data was added into a 3D printing (RS600, Union Tech, Shanghai, China) to create an airway mold made from photosensitive resins. The dimensions of the area of interest in the 3D printed airway mold were measured in the airway. Then, using the 3D printed airway model as a template, the covered self-expandable Y-shaped metallic airway stents (Micro-Tech, Nanjing, China) were made of temperature-memory nickel-titanium alloy. The stents were inserted using flexible bronchoscopy for evaluation and guidewires placement. Posteriorly, a stent delivery system was advanced out of the endotracheal tube, and the stent was deployed under fluoroscopy guidance (37,38).
Other materials which can be directly printed to produce an airway stent have been subject of research. In 2015, Hussain at the University of South Carolina used 3D printing technology to assess possible improvements on existing stents through designing and printing a bioresorbable tracheobronchial stent. He concluded that polycaprolactone (PCL) is fused deposition modeling printing-compatible, and thermoplastic polyurethane (TPU) is potentially viable as a biologically degradable silicone alternative (40). Wood and colleagues developed a platform for designing and production of 3D printing flexible airway stents with elastomeric polyurethane (EPU), after performing comparative tests with silicone stents, they concluded that 3D printed EPU stent has similar performance (41). Paunović et al. reported using digital light 3D printing customized bioresorbable stent in an in-vivo study of healthy rabbits. The stents were made of a dual polymer which was biocompatible and stayed in place for 7 weeks (42).
These 3D printing materials are promising because of the possible rapid and direct manufacturing, customization, biocompatibility, and degradability. But further research is needed before it is recommended for use in patients.
Current evidence in 3D printing PSS
Since 2015, there has been an increased number of publications on 3D printing and airway stents. We found ten studies using 3D printing and PSS in humans (22,34-39,43-45) (Table 1). Most studies were done on benign airway diseases such as post-surgical airway complication, post-transplant airway disease, tracheobronchomalacia, GPA airway, or post-radiotherapy airway complication. The most frequently used stent material in benign airway diseases was silicone (22,35,36,39,43,46,47).
Table 1
Author | Reference no. | Year | Type of study | No. of patients | No. of stents | Type of airway disease | Airway mold | Stent material | Type stent | F/U in months | Comparation with CAS | Results | AEs | Processing time in days |
---|---|---|---|---|---|---|---|---|---|---|---|---|---|---|
Cheng et al. | 34 | 2015 | Case study | 1 | 1 | Benign | Yes (virtual) | Silicone | T-Tube | 4 | No | Improvement in phonation, no granulation tissue after 4 months | N/D | N/D |
Guibert et al. | 35 | 2017 | Case study | 1 | 1 | Benign | Yes | Silicone | Right bronchial stent branched to the RUL | 2.5 | No | Improvement of symptoms, PEF | N/D | N/D |
Gildea et al. | 22 | 2018 | Case study | 2 | 2 | Benign | Yes | Silicone | Y-stent for LMB | 12 | No | Increase time between procedures. Increase stent life. Decrease procedure time | N/D | 7 |
Schweiger et al. | 36 | 2018 | Case study | 2 | 2 | Benign | Yes | Silicone | Y tracheobronchial | 5 and 8 | No | Symptoms improvement | N/D | 7 |
Guibert et al. | 39 | 2019 | Prospective | 10 | 10 | Benign | Yes | Silicone | Y bronchial and tracheobronchial stent | 4 | No | 90% congruence, 80% improvement in dyspnea, quality of life and FEV1 or PEF | 3 months complication rate 40% | 50 |
Aravena Leon et al. | 43 | 2019 | Retrospective | 4 | 13 | Benign | Yes | Silicone | Y bronchial and tracheobronchial stent | 21.6 | Yes | No difference compared to commercial stents in RB loading, placement, removal. Increase time between procedures. Increase stent life | PSS experienced a lower severity of migration. All other AEs were not statistically different between the two groups | 7 |
Duong et al. | 44 | 2020 | Case study | 1 | 2 | Malignant | N/D | Polyurethane | Right bronchial stent branched to the RUL | 2 | No | Symptoms improvement | PSS replacement at 2 months and migration and removal of the 2nd PSS | N/D |
Huang et al. | 45 | 2021 | Retrospective | 6 | 7 | Benign (post esophagectomy) | Yes | Hybrid (nitinol, silicone, and PTFE) | Y tracheobronchial | 16.7 | No | All fistulas sealed. Leaking control in 6 patients. KPS improvement | 2 had mucus retention, 1 had excessive granulation tissue and stent removal | N/D |
Shan et al. | 37 | 2021 | Retrospective | 12 | 13 | Malignant | Yes | Hybrid (nitinol, silicone, and PTFE) | Y stent (1 bronchial, rest tracheobronchial) | 5.6 | No | 1 patient had 2 stents; 11 had significant symptoms improvement, KPS improvement | 4 had mucus retention, 2 excessive granulation tissue, 0 migration, 0 removal | 4 |
Shan et al. | 38 | 2021 | Retrospective | 26 | 26 | Malignant ADF and post esophagectomy | Yes | Hybrid (nitinol, silicone, and PTFE) | Y stent (1 bronchial, rest tracheobronchial) | 5 | No | Clinical success rate 80%. Resolution ADF post-esophagectomy (9/16). Resolution malignant ADF (0/10). KPS improvement | 2 (7.69%) granulation tissue treated cryotherapy and stent removal; 5 (19.23%) sputum retention, treated w/suction; 1 (3.84%) had stent migration underwent a second stent; 1 (3.84%) not tolerate the stent and was removed | N/D |
3D, three-dimensional; F/U, follow-up; CAS, commercially available stents; AEs, adverse events; N/D, not described; RUL, right upper lobe; PEF, peak expiratory flow; LMB, left main bronchus; FEV1, forced expiratory volume in the first second; RB, rigid bronchoscopy; PSS, patient-specific airway stent; PTFE, polytetrafluoroethylene; KPS, Karnofsky Performance Scale; ADF, aerodigestive fistula.
More recent research has been done on malignant central airway obstruction or malignant aerodigestive fistula. They almost exclusively, but one, used covered metal stents (37,38,44).
Nine studies described printing out a 3D airway mold to create the PSS (there is no description in one study). And mostly, the stents were Y tracheobronchial or bronchial. Interestingly, six studies used the 3D mold to make Y-bronchial stents or a bronchial branch to the right upper lobe.
All studies showed improvement in symptoms (Table 1). Guibert et al. used 3D printing silicone PSS in 10 patients (mostly post-transplant airway complications) and described a high rate of congruence between the stent and the airway, 80% of improvement in dyspnea [>1 New York Heart Association (NYHA) point gain], quality of life [>10% VQ11 chronic obstructive pulmonary disease (COPD)-specific quality of life questionnaire score increase], and pulmonary function test [>10% forced expiratory volume in the first second (FEV1) or peak expiratory flow (PEF) increase] (39). Aravena Leon et al. presented a retrospective study of patients who received 3D printing silicone PSS at the Cleveland Clinic. Interventional pulmonologists involved in the procedures completed a survey, and two physicians graded stent-related adverse events (AEs) based on the Common Terminology Criteria for Adverse Events Scoring System. A total of 13 PSSs were placed in 4 patients. Compared to the CAS, no difference was described to load, place, or remove the PSS (P>0.05). Bronchoscopists noted a significant clinical improvement after the PSS was placed (P=0.03). The average lifespan of the PSS was significantly higher than the CAS (300.2 vs. 124.0 days, P<0.001). The average duration between bronchoscopies was substantially longer with PSS than CAS (65.6 vs. 36.6 days, P=0.004) (43,47).
Shan et al. published a study of 12 patients with malignant airway obstruction secondary to lung or esophageal cancer, 13 covered metal PSS were placed. They described an improvement in the Hugh-Jones dyspnea scale (P=0.003) and Karnofsky Performance Scale (KPS) (P=0.006) (37).
Concerning to the AEs depicted in the different trials. Guibert and colleagues described a 40% complication rate at 3 months, one patient had a mucus plug, two stent migrations, and one untreatable cough. Three of them required stent removal. At four months of follow-up, an additional mucus plug event occurred, and one patient developed distal stenosis at a lobar level that required balloon dilation. Non-life-threatening complications were observed (39). Aravena Leon and colleagues demonstrated that silicone PSS experienced a lower migration severity than CAS (P=0.0225). All other AEs were not statistically different between the two groups (43,47). Shan et al. showed in covered metal PSS a complication rate of 50% (6/12) in 5.6 months of follow-up. Four patients experienced mucus plugging, two had excessive granulation tissue formation and none had migration or required stent removal (37).
Discussion
The use of any stent in complex benign or malignant airway disease is always an option of last resort. Stent-related complications are prevalent and can have catastrophic consequences. The three most common adverse events related to silicone stenting are migration, stent occlusion (by either granulation tissue or mucus), and infection. These three conditions can also be significantly intertwined, as others have shown the association between stent granulation and infection (6). The complication rate described in the literature is variable and has been communicated between 21.5% to 65% (5,6,14-18). Many patients experience long-term palliation despite these risks, even with non-malignant conditions.
The studies reported in this review showed that the 3D printing PSS is at least as safe as the CAS. The rate of complications is similar, and no threatened-life complications were described. The production of 3D printing PSS in the shape of Y-bronchial and Y-tracheobronchial stent that are congruent and fit the complex airways of those patients probably helped to decrease the migration rate compared to CAS (43,47).
The technical success of the PSS congruence to the complex airway is high in several of the studies presented here. It potentially means a decrease in AEs. Many of the complications associated with stents are directly related to fit problems. A stent that is too loose or too tight has a higher migration rate. A stent that puts too much pressure on the airway may lead to tissue necrosis and perforation. A stent that does not sit properly may incite granulation at the ends or cause impaired secretion clearance. A stent that is too long may create increased resistance and a barrier to clear secretions. Multiple methods and applications of stent shape and sizing are possible choosing size is now an interesting challenge. Further, material properties may have clinical implications. Even in silicone stents, there are stents with variable durometer and modulus that can impact entirely new considerations of wall stress.
The PSS could be loaded and deployed with existing standard techniques, similar to conventional stents (43,47). This is an understated but essential finding, as some complex stent designs, like the dynamic Y-stent, require special equipment for placement.
The benefits could be broad. There is a consistent improvement in symptoms through all the studies described. One trial showed an increase in quality of life and pulmonary function test (39). Only one study compared the PSS to CAS. This demonstrated an increase in stent life and time between procedures, it might be correlated to an improvement in the quality of life of patients with complex benign airway diseases who ordinarily require several and consecutive bronchoscopies in an attempt to achieve palliation (43,47).
Related to malignant disease, an essential additional finding is associated with the increase in the KPS (37,38,44). It could be linked with the resolution of the obstruction, the augmented congruence, and the decrease of AEs, which could improve symptoms and the ability to receive additional oncologic therapy.
The studies described in this review are not large enough to draw broad conclusions about the use of patient-specific stenting. Most of them are studies of cases or retrospective cohorts which are also subject to significant bias and should only be viewed as hypothesis-generating. They merely represent an initial, conservative user for a proof-of-concept. Despite their small sample size, the initial experience has shown that patient-specific stents are very effective and safe in the palliative treatment of extraordinary complex airway disease, beyond all the best available care to date. Designing outcomes trials with a wide variety of diseases and no standard stent design features can be quite challenging.
Other advances are a matter of ongoing research. New materials that might be directly printed and could be biodegradable when temporal stents are desirable (42). 3D printing drug-eluting stents that could be a potential treatment strategy to avoid excessive granulation tissue, prevent infections, and control airway-related malignancy (48).
Conclusions
3D printing technology has advanced important steps in the last decades. Healthcare has used this technology for education, preoperative planning, medical devices, prostheses, implants, and medical models. Respiratory medicine is leading a revolution in personalized medicine, making customized airway stents that fit the specific needs of patients with complex malignant or benign airway disease. These PSSs created using 3D printing technology have shown the potential to improve symptoms, quality of life, performance status, stent life and decrease the time between procedures and AEs. Randomized control trials with a large number of patients comparing PSS against CAS will be needed but challenging to determine the real impact that this technology will have on this group of patients. The ability to design many more variables into the process adds further complexity.
Acknowledgments
The authors of this review wish to thank to Adrianna Baiera and Keith Grafmeyer for assisting in previous research on which the work was based.
Funding: None.
Footnote
Provenance and Peer Review: This article was commissioned by the Guest Editor (Calvin S. H. Ng) for the series “Lung Cancer Management—The Next Decade” published in Annals of Translational Medicine. The article has undergone external peer review.
Conflicts of Interest: Both authors have completed the ICMJE uniform disclosure form (available at https://atm.amegroups.com/article/view/10.21037/atm-22-2878/coif). The series “Lung Cancer Management—The Next Decade” was commissioned by the editorial office without any funding or sponsorship. TRG reports that Cleveland Clinic and Cleveland Clinic Institutional Officials/Leaders have an equity interest in Visionair and are entitled to royalty payments from the company for technology developed at Cleveland Clinic. Visionair is the manufacturer of the stents. TRG is the inventor and may be entitled to royalty payments from the company in accordance with Cleveland Clinic policy. The authors have no other conflicts of interest to declare.
Ethical Statement: The authors are accountable for all aspects of the work in ensuring that questions related to the accuracy or integrity of any part of the work are appropriately investigated and resolved.
Open Access Statement: This is an Open Access article distributed in accordance with the Creative Commons Attribution-NonCommercial-NoDerivs 4.0 International License (CC BY-NC-ND 4.0), which permits the non-commercial replication and distribution of the article with the strict proviso that no changes or edits are made and the original work is properly cited (including links to both the formal publication through the relevant DOI and the license). See: https://creativecommons.org/licenses/by-nc-nd/4.0/.
References
- Mehta AC, Jain P, Gildea TR. Diseases of the Central Airways A Clinical Guide. Totowa, NJ, USA: Humana Cham, 2016:385.
- Ernst A, Herth FJF. Principles and practice of interventional pulmonology. Principles and Practice of Interventional Pulmonology. New York, NY, USA: Springer, 2013:1-757.
- Ost DE, Ernst A, Grosu HB, et al. Therapeutic bronchoscopy for malignant central airway obstruction: Success rates and impact on dyspnea and quality of life. Chest 2015;147:1282-98. [Crossref] [PubMed]
- Chua AP, Santacruz JF, Gildea TR. Pulmonary complications of cancer therapy and central airway obstruction. In: Supportive Oncology. Elsevier, 2011.
- Aravena C, Almeida FA, Mukhopadhyay S, et al. Idiopathic subglottic stenosis: a review. J Thorac Dis 2020;12:1100-11. [Crossref] [PubMed]
- Dutau H, Dumon JF. Airway Stenting Revisited: 30 Years, the Age of Reason? J Bronchology Interv Pulmonol 2017;24:257-9. [Crossref] [PubMed]
- Martinez-Ballarin JI, Diaz-Jimenez JP, Castro MJ, et al. Silicone stents in the management of benign tracheobronchial stenoses. Tolerance and early results in 63 patients. Chest 1996;109:626-9. [Crossref] [PubMed]
- Dumon JF. A dedicated tracheobronchial stent. Chest 1990;97:328-32. [Crossref] [PubMed]
- Folch E, Keyes C. Airway stents. Ann Cardiothorac Surg 2018;7:273-83. [Crossref] [PubMed]
- Ost DE, Ernst A, Grosu HB, et al. Complications Following Therapeutic Bronchoscopy for Malignant Central Airway Obstruction: Results of the AQuIRE Registry. Chest 2015;148:450-71. [Crossref] [PubMed]
- Dumon JF, Cavaliere S, Diaz-Jimenez JP, et al. Seven-year experience with the dumon prosthesis. Journal of Bronchology 1996;3:6-10. [Crossref]
- Noppen M, Stratakos G, D'Haese J, et al. Removal of covered self-expandable metallic airway stents in benign disorders: indications, technique, and outcomes. Chest 2005;127:482-7. [Crossref] [PubMed]
- Ernst A, Silvestri GA, Johnstone D, et al. Interventional pulmonary procedures: Guidelines from the American College of Chest Physicians. Chest 2003;123:1693-717. [Crossref] [PubMed]
- Flannery A, Daneshvar C, Dutau H, et al. The Art of Rigid Bronchoscopy and Airway Stenting. Clin Chest Med 2018;39:149-67. [Crossref] [PubMed]
- Shapshay SM, Valdez TA. Bronchoscopic management of benign stenosis. Chest Surg Clin N Am 2001;11:749-68. [PubMed]
- Mudambi L, Miller R, Eapen GA. Malignant central airway obstruction. J Thorac Dis 2017;9:S1087-110. [Crossref] [PubMed]
- Wood DE, Liu YH, Vallières E, et al. Airway stenting for malignant and benign tracheobronchial stenosis. Ann Thorac Surg 2003;76:167-74. [Crossref] [PubMed]
- Breen DP, Dutau H. On-site customization of silicone stents: towards optimal palliation of complex airway conditions. Respiration 2009;77:447-53. [Crossref] [PubMed]
- Cheng GZ, San Jose Estepar R, Folch E, et al. Three-dimensional Printing and 3D Slicer: Powerful Tools in Understanding and Treating Structural Lung Disease. Chest 2016;149:1136-42. [Crossref] [PubMed]
- Young BP, Machuzak MS, Gildea TR. Initial Clinical Experience Using 3d Printing And Patient-Specific Airway Stents: Compassionate Use Of 3d Printed Patient-Specific Airway Stents. Am J Respir Crit Care Med 2017;195:A1711.
- Alraiyes AH, Avasarala SK, Machuzak MS, et al. 3D printing for airway disease. AME Med J 2019;4:14. [Crossref] [PubMed]
- Gildea TR, Young BP, Machuzak MS. Application of 3D printing for patient-specific silicone stents: 1-year follow-up on 2 patients. Respiration 2018;96:488-94. [Crossref] [PubMed]
- Hull CW. Apparatus for Production of Three-Dimensional Objects By Stereo Thography. Patent 1986. Available online: https://patents.google.com/patent/US4575330
- Deckard C. Method and apparatus for producing parts by selective sintering. Patent 1989. Available online: https://patents.google.com/patent/US4863538
- Crump S. Apparatus and method for creating three-dimensional objects. Patent 1992.
- Sachs E, Cima M, Cornie J, et al. Three-Dimensional Printing: The Physics and Implications of Additive Manufacturing. CIRP Annals 1993;42:257-60. [Crossref]
- Whitaker M. The history of 3D printing in healthcare. Bulletin of The Royal College of Surgeons of England 2014;96:228-9. (Royal College of Surgeons). [Crossref]
- Dodziuk H. Applications of 3D printing in healthcare. Kardiochir Torakochirurgia Pol 2016;13:283-93. [Crossref] [PubMed]
- Willson K, Atala A. Medical 3D Printing: Tools and Techniques, Today and Tomorrow. Annu Rev Chem Biomol Eng 2022;13:481-99. [Crossref] [PubMed]
- McGurk M, Amis AA, Potamianos P, et al. Rapid prototyping techniques for anatomical modelling in medicine. Ann R Coll Surg Engl 1997;79:169-74. [PubMed]
- Matsumoto JS, Morris JM, Foley TA, et al. Three-dimensional Physical Modeling: Applications and Experience at Mayo Clinic. Radiographics 2015;35:1989-2006. [Crossref] [PubMed]
- Tam MD, Laycock SD, Jayne D, et al. 3-D printouts of the tracheobronchial tree generated from CT images as an aid to management in a case of tracheobronchial chondromalacia caused by relapsing polychondritis. J Radiol Case Rep 2013;7:34-43. [Crossref] [PubMed]
- Zopf DA, Hollister SJ, Nelson ME, et al. Bioresorbable airway splint created with a three-dimensional printer. N Engl J Med 2013;368:2043-5. [Crossref] [PubMed]
- Cheng GZ, Folch E, Brik R, et al. Three-dimensional modeled T-tube design and insertion in a patient with tracheal dehiscence. Chest 2015;148:e106-8. [Crossref] [PubMed]
- Guibert N, Didier A, Moreno B, et al. Treatment of Post-transplant Complex Airway Stenosis with a Three-Dimensional, Computer-assisted Customized Airway Stent. Am J Respir Crit Care Med 2017;195:e31-3. [Crossref] [PubMed]
- Schweiger T, Gildea TR, Prosch H, et al. Patient-specific, 3-dimensionally engineered silicone Y-stents in tracheobronchomalacia: Clinical experience with a novel type of airway stent. J Thorac Cardiovasc Surg 2018;156:2019-21. [Crossref] [PubMed]
- Shan Q, Huang W, Shang M, et al. Customization of stent design for treating malignant airway stenosis with the aid of three-dimensional printing. Quant Imaging Med Surg 2021;11:1437-46. [Crossref] [PubMed]
- Shan Q, Huang W, Shang M, et al. Treatment of aerodigestive fistulas with a novel covered metallic Y-shaped segmented airway stent customized with the assistance of 3D printing. Ann Transl Med 2021;9:1051. [Crossref] [PubMed]
- Guibert N, Didier A, Moreno B, et al. Treatment of complex airway stenoses using patient-specific 3D-engineered stents: a proof-of-concept study. Thorax 2019;74:810-3. [Crossref] [PubMed]
- Hussain NM. Considerations for Development of 3D Printed Bronchial Material Considerations for Development of 3D Printed Bronchial and Tracheal Stents and Tracheal Stents. University of South Carolina, 2015. Available online: https://scholarcommons.sc.edu/etd
- Wood C, Cheng G, Miller A, et al. Mechanical Characteristics of 3D Printed Airway Stent. American Journal of Respiratory and Critical Care Medicine 2018;197:A1739.
- Paunović N, Bao Y, Coulter FB, et al. Digital light 3D printing of customized bioresorbable airway stents with elastomeric properties. Sci Adv 2021;7:eabe9499. [Crossref] [PubMed]
- Aravena Leon C, Inaty H, Urbas A, et al. Early outcomes with 3D printing and airway stents. Chest 2019;156:A199-200. [Crossref]
- Duong DK, Bedi HS, Guo HH, et al. Custom 3D-Printed Airway Stent for the Management of Complex Malignant Airway Obstruction. American Journal of Respiratory and Critical Care Medicine 2020;201:A4883.
- Huang W, Shan Q, Wu Z, et al. Retrievable covered metallic segmented Y airway stent for gastrorespiratory fistula of carina or main bronchi. J Thorac Cardiovasc Surg 2021;161:1664-71.e2. [Crossref] [PubMed]
- Cheng GZ, Folch E, Wilson A, et al. 3D Printing and Personalized Airway Stents. Pulm Ther 2017;3:59-66. [Crossref]
- Aravena Leon C, Inaty H, Urbas A, et al. Clinical outcomes with 3D patient specific airway stents compared to commercially available airway stents. 21st World Congr Bronchol Interv Pulmonol 2020:397.
- Xu J. Development of 3D-Printed, Drug-Eluting Airway Stents for the Personalised and Local Treatment of Central Airway Pathologies Statement of Originality. The University of Sydney, 2021. Available online: https://hdl.handle.net/2123/27402