Sodium Danshensu protects against oxygen glucose deprivation/reoxygenation-induced astrocytes injury through regulating NOD-like receptor pyrin domain containing 3 (NLRP3) inflammasome and tuberous sclerosis complex-2 (TSC2)/mammalian target of rapamycin (mTOR) pathways
Introduction
Salvia miltiorrhiza, called Danshen in Chinese, is a common herbal medicine used widely to enhance blood circulation, dilate coronary arteries, and improve blood flow (1,2). Danshen and its derivatives have been discovered to have pro-angiogenic, anti-inflammatory, and antioxidant properties, as well as inhibit platelet adhesion and aggregation in cardiovascular ischemia (3-5). The National Medical Products Administration of China, a government department similar to the American Food and Drug Administration (FDA), has approved about 900 Danshen-related drugs (2). Sodium Danshensu [sodium D-(+)-β-(3,4-dihydroxyphenyl) lactate, C9H9O5Na, molecular weight 220], is an important water-soluble derivative of Danshen (6). In vitro and in vivo studies have suggested that sodium Danshensu exerts various pharmacological effects, such as anti-inflammatory, coronary artery relaxation, and myocardial ischemia-reperfusion injury prevention (7-9). In clinical application, danshensu sodium can be used for the prevention of hypoxic pulmonary hypertension and the treatment of psoriasis (10,11). Besides, sodium Danshensu actively enhances neurogenesis and collagen production in post-ischemic injury, and has potential therapeutic effect on ischemic stroke (1). The existing data suggest that sodium Danshensu exerts a strong protective effect on ischemic stroke; however, the underlying molecular mechanism of this effect remains to be further investigated.
Ischemic stroke, a cerebrovascular accident, is a severe brain disorder with a high incidence of disability, morbidity, and mortality (12,13). It is characterized by abnormal blood circulation and inadequate oxygen supply to the brain (14). Several factors have been identified as involved in the development and progression of ischemic stroke, including nutrient insufficiency, ischemic stress, and dysregulation in autophagy (15). Although considerable efforts have been made to advance our knowledge on ischemic stroke, the understanding of ischemic stroke remains limited. Until recently, an intravenous thrombolytic agent with a recombinant tissue plasminogen activator was the only recommended medication for treating acute stroke, but the narrow therapeutic window and danger of intracerebral hemorrhage make this therapy unsuitable for many individuals (16). In recent years, herbal extracts and their derivatives have shown extraordinary efficacy on ischemic stroke, such as baicalin, danshensu and isoquercetin, which can improve the cell damage caused by cerebral ischemia, but the side effects are limited (1,14,17,18). Therefore, exploring new herbal therapeutic options to manage ischemic stroke is warranted.
Astrocytes, the primary glial cells of the brain, are critical for maintaining normal brain structure and function through interacting with neurons, oligodendrocytes, and endothelial cells (15,19,20). Notably, reactive astrogliosis, known as astrocyte dysfunction, is a common pathological feature in focal ischemic stroke, and dysfunction in astrocytes induces neural injury and promotes stroke-induced disability (21-23). Astrocyte homeostasis is critical to neurons, especially during cerebral ischemia, which reduces ischemia-induced disabilities (24,25). Astrocyte autophagy flux plays a crucial role in astrocyte homeostasis (24,25). Notably, the in vitro culture of astrocytes under oxygen-glucose deprivation/reoxygenation (OGD/R) insult can resemble the process of ischemia-reperfusion (25). Therefore, it is suggested that astrocytes are highly associated with ischemic stroke, which makes astrocytes an ideal therapeutic target for the management of cerebral ischemic stroke.
In this study, we aimed to investigate the role of sodium Danshensu on ischemic stroke using an in vitro model of astrocytic cerebral ischemic stroke and explored the molecular mechanism underlying this protective effect. We present the following article in accordance with the MDAR reporting checklist (available at https://atm.amegroups.com/article/view/10.21037/atm-22-2143/rc).
Methods
Primary astrocyte isolation and culture
Primary astrocytes were isolated from 24-hour postnatal male Sprague-Dawley (SD) rats, according to a previously described technique with minor modification (26). The cells were cultured in a poly-d-lysine coated 75 cm2 flask, and the seeded concentration was 1×106 cells/mL. The cells were cultured in Dulbecco’s modified Eagle’s medium (DMEM), supplemented with 10% fetal bovine serum (FBS) and 1% antibiotics at 37 ℃ with 5% CO2 supplement. The rats were obtained from Chengdu Dasuo Experimental Animal Co., LTD. Experiment was performed under a project license (No. CDYFY-IACUC-202208QR027) granted by ethics board of the First Affiliated Hospital of Nanchang University, in compliance with the First Affiliated Hospital of Nanchang University guidelines for the care and use of animals.
Primary astrocyte OGD/R modeling and drug treatment
The primary astrocyte OGD/R model was developed based on the previously described protocol with minor changes (22). Briefly, the primary astrocytes were collected and washed with phosphate-buffered saline (PBS). For OGD insult, the cells were grown in glucose- and FBS-free DMEM for 6 hours at 37 ℃ in an anoxic incubator. For reperfusion, the culture medium was changed back to high-glucose DMEM, containing 10% fetal bovine serum (FBS), and kept in a normoxic incubator for 24 hours. The cells in the control group were always cultured with normal high-glucose and FBS-containing DMEM in a regular oxygen incubator. To understand the effect of sodium Danshensu, the cells were exposed to OGD/R insult with or without sodium Danshensu (0, 25, 50, and 100 µM), except the control group.
3-(4,5-dimethylthiazol-2-yl)-2,5-dophenyl tetrazolium bromide (MTT) assay
A MTT (Sigma-Aldrich, St. Louis, MO, USA) assay was performed to evaluate cell viability. Following specific treatment, the primary astrocytes were plated in a 96 well plate at a density of 2×104 cells/well and incubated for 24 hours. The MTT (5 mg/mL) solution was added to each well and kept at 37 ℃ for 4 hours. The culture medium was then discarded, and 150 µL of dimethyl sulfoxide (DMSO) was applied to each well. Finally, the absorbance was recorded at 490 nm by a microplate reader (Bio-Rad Instruments, Hercules, CA, USA).
Flow cytometry assay
Apoptosis was analyzed by Annexin V/propidium iodide (PI) flow cytometry kit [Becton, Dickinson, and Co. (BD), Franklin Lakes, NJ, USA], following the manufacturer’s protocol. After treatment, the cells were harvested and washed with 250 µL ice-cold PBS, followed by staining with Annexin V and PI for 30 minutes in the absence of light on ice. The cells were then washed with PBS to remove unbound antibodies and resuspended in 200 µL PBS buffer for flow cytometry. The apoptosis was detected via a flow cytometer (BD Bioscience, USA), and the data was analyzed by Flowjo software (BD, USA).
Estimation of caspase-3 activity
Caspase-3 activity was evaluated using a caspase-3 assay kit (Beyotime, Haimen, Jiangsu, China). Following treatment, the cells were collected, and a caspase-3 kit was utilized by following the manufacturer’s protocol. The absorbance was measured at 405 nm by a spectrophotometer (Bio-Rad Instruments, USA), and the results were expressed as U/mL.
Enzyme-linked immunosorbent assay
The level of cytokines [tumor necrosis factor-α (TNF-α), interleukin (IL)-1β, and IL-6] secretion were quantified using commercialized enzyme-linked immunosorbent assay (ELISA) kits (Abcam, Cambridge, UK) by following manufacturer’s instructions, and the absorbance was determined by spectrophotometer (Bio-Rad Instruments, USA).
Lactate dehydrogenase (LDH) assay
To determine the LDH release in the primary astrocytes, an LDH cytotoxicity detection kit (Beyotime, China) was used according to the manufacturer’s instructions.
Western blotting
The total proteins were extracted using radioimmunoprecipitation assay (RIPA) lysis buffer (Solarbio, Beijing, China), and the protein was quantified with a bicinchoninic acid (BCA) protein assay kit (Tanon, Shanghai, China). About 40 g of the proteins were separated according to the mass by 10% sodium dodecyl sulfate polyacrylamide gel electrophoresis (SDS-PAGE). Subsequently, the proteins were electroblotted onto polyvinylidene difluoride (PVDF) membranes. The membranes were then blocked with 5% nonfat milk, then incubated with the respective primary antibodies: NOD-like receptor pyrin domain containing 3 (NLRP3) (cat. no. Ab263899; 1:1,000; Abcam), high mobility group protein B1 (HMGB1) (cat. no. Ab18256; 1:1,000; Abcam), phosphorylated-p65 (p-p65) (cat. no. Ab76302; 1:1,000; Abcam), p65 (cat. no. 8242; 1:1,000; Cell Signaling Technology, Inc. (CST), Danvers, MA, USA), Beclin1 (cat. no. Ab207612; 1:1,000; Abcam), P62 (cat. no. 39749; CST), LC3B (cat. no. Ab192890; 1:1,000; Abcam), tuberous sclerosis complex-2 (TSC2) (cat. no. 3990; 1:1,000; CST), p-mammalian target of rapamycin (mTOR) (cat. no. Ab137133; 1:1,000; Abcam), mTOR (cat. no. Ab134903; 1:1,000; Abcam), and glyceraldehyde-3-phosphate dehydrogenase (GAPDH) (cat. no. 5174; 1:1,000; CST) for 24 hours at 4 ℃. Subsequently, the membranes were washed, and the secondary antibody (cat. no. ab96899; 1:2,000; Abcam) was applied to conjugate for 2 hours at room temperature. Finally, the western blot images were visualized using enhanced chemiluminescence (ECL) and recorded by Gel Imaging System (Bio-Rad, USA).
Quantitative real-time polymerase chain reaction (qRT-PCR)
qRT-PCR was used to determine the NLRP3, high-mobility group box 1 (HMGB1), p-p65, p65, TSC2, and mTOR expression levels after sodium Danshensu treatment. The cells were collected, and the total RNA was extracted by using TRIzol (Invitrogen, Carlsbad, CA, USA) RNA extraction kit from astrocytes, and the RNA concentration was determined by a NanoDrop spectrophotometer (Thermo Fisher Scientific, Waltham, MA, USA). About 2 µg total RNA was used to synthesize complementary DNA using PrimeScript RT Reagent Kit (Takara Bio., Beijing, China). The qPCR reaction was conducted using SYBR Green PCR Master mix (Takara, Otsu, Japan) in a thermocycler (Thermo Fisher Scientific, USA). The relative messenger RNA (mRNA) levels of target genes were analyzed by 2−∆∆Ct formula.
Statistical analysis
All experiments were repeated at least 3 times. The data analysis was conducted by using SPSS 20.0 software (IBM Corp., Armonk, NY, USA). The data were presented as mean ± SD. One-way analysis of variance (ANOVA) followed by Tukey’s test was used to compare the difference among 3 or more groups.
Results
The effects of sodium Danshensu on cell cytotoxicity
The chemical molecular structure formula of sodium Danshensu is displayed in Figure 1A. To understand the toxic effect of sodium Danshensu, we treated primary cultured astrocytes with different amounts (0, 25, 50, and 100 µM) of sodium Danshensu for 24 hours. We found that sodium Danshensu had no considerable effect on cell viability and LDH production from primary astrocytes (Figure 1B,1C). It was implied that sodium Danshensu is not toxic to primary cultured astrocytes.
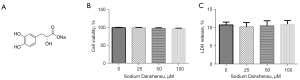
Sodium Danshensu protects OGD/R-induced primary astrocyte injury
Whether sodium Danshensu can prevent astrocytes injury, we established the OGD/R model according to previously reported protocols with minor modifications (22). The OGD/R group showed a substantial decrease in cell viability (Figure 2A), a significant increase in LDH release (Figure 2B), a significant increase in apoptosis (Figure 2C,2D), and a considerable increase in caspase-3 activity (Figure 2E) compared to the control group. We treated OGD/R exposed astrocytes with various doses of sodium Danshensu and found that sodium Danshensu dose-dependently decreased LDH release, increased cell viability, decreased apoptosis, and decreased caspase-3 activity in comparison to the OGD/R group. Besides, IL-6, IL-1β, and TNF-α secretion were markedly increased in the supernatant of the OGD/R group. While sodium Danshensu showed a dose-dependent decrease in IL-6, IL-1β, and TNF-α secretion compared with the OGD/R group (Figure 3A-3C). The mRNA expression level of TNF-α, IL-6, and IL-1β was the same pattern (Figure 3D-3F).
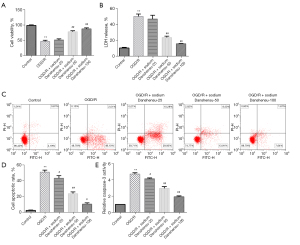
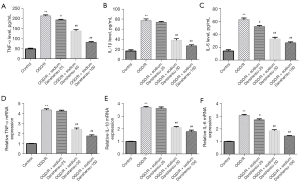
Besides, the protein and mRNA expression of NLRP3 and HMGB1 were considerably upregulated in primary astrocytes of OGD/R group (Figure 4A-4C). The p-p65 protein and p-p65/p65 ratio were also markedly enhanced (Figure 4D,4E), while p65 protein and mRNA expression were similar between the groups (Figure 4D,4F) in OGD/R group. On the other hand, sodium Danshensu showed a dose-dependent decreased in protein and mRNA levels of NLRP3 and HMGB1 (Figure 4A-4C). The p-p65 protein and p-p65/p65 ratio were considerably reduced (Figure 4D,4E). Besides, the p65 protein and mRNA expression were incomparable after sodium Danshensu treatment (Figure 4D,4F). These results suggested that sodium Danshensu could protect astrocytes from ischemia/reperfusion insult.
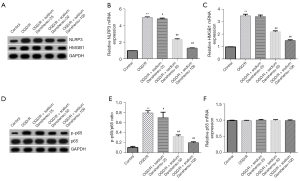
Sodium Danshensu promotes OGD/R-induced autophagy in primary astrocytes
Autophagy flux has an inevitable function in astrocytes injury and highly associated with ischemic stroke. To understand the role of autophagy and sodium Danshensu in our in vitro OGD/R model, we studied the role of sodium Danshensu on astrocyte autophagy. After OGD/R insult, there was no considerable change in autophagy-associated protein Beclin 1 expression in primary astrocytes (Figure 5A,5B). While we noticed an increase in P62 protein expression (Figure 5A,5C) and LC3B-II/LC3B-I ratio (Figure 5A,5D) after OGD/R insult. Interestingly, sodium Danshensu dose-dependently upregulated Beclin 1 expression in primary astrocytes, decreased P62 protein level, and further increased LC3B-II/LC3B-I ratio compared to the OGD/R group, suggesting that autophagy was promoted after sodium Danshensu treatment.
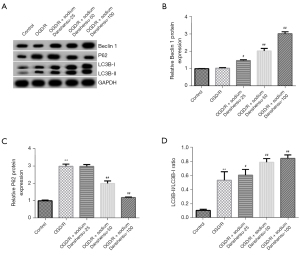
To further understand the molecular mechanism, we studied the role of sodium Danshensu on different autophagy-related pathways and found TSC2/mTOR was the most obvious one. The TSC2/mTOR pathway is an important pathway in autophagy regulation. After OGD/R insult, we found there was no significant change in TSC2 protein in primary astrocytes (Figure 6A,6B), while the p-mTOR protein (Figure 6A) and p-mTOR/mTOR (Figure 6C) ratio were slightly reduced. Notably, sodium Danshensu showed a dose-dependently increased TSC2 protein and mRNA expression and further decreased p-mTOR protein levels and p-mTOR/mTOR ratio in astrocytes compared to the OGD/R group. These findings demonstrate that sodium Danshensu may promote autophagy in OGD/R exposed astrocytes by modulating the TSC2/mTOR pathway.
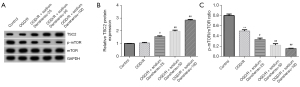
Discussion
Ischemic stroke is a leading cause of neurological morbidity and mortality globally, and the rate of death and disability due to stroke are gradually increasing (27,28). Hypoxia and ischemia in brain tissue after cerebral ischemia led to inadequate energy supply, which in turn promotes neuronal damage. In the absence of oxygen, mitochondrial function is dysregulated, leading to cellular damage, such as astrocyte injury, which is critical for neurons in ischemic stroke (29). Therefore, restoring cellular energy balance and astrocyte homeostasis are the key to the management of stroke. In this study, we investigated the role of sodium Danshensu in OGD/R-induced astrocyte injury. Our results shed light on the molecular mechanisms behind sodium Danshensu-mediated astrocyte protection during ischemic stroke.
Previous studies have demonstrated that sodium Danshensu has neuroprotective effects, and most importantly, can cross the blood brain barrier (18,30). It also has a strong capacity to increase superoxide dismutase (SOD) and glutathione peroxidase (GPx) activity and decrease the level of malondialdehyde (MDA) and the reactive oxygen species (ROS), which make sodium Danshensu a potent antioxidant agent (30,31). Moreover, Danshensu and Danshen derivatives decrease inflammation, and they have protective roles in myocardial ischemia-reperfusion injury (7,32,33). These results suggested that sodium Danshensu could help to prevent subsequent damage responses after ischemia. However, the molecular mechanism was not elucidated, and further investigation is required.
In this study, we used primary astrocytes to understand the effect of sodium Danshensu in astrocyte injury caused by the OGD/R insult. Primary astrocytes exposed to OGD/R insult are widely used as an in vitro cell model of ischemic stroke (22,34,35). Here, we adopted the OGD/R model of primary astrocytes to mimic cerebral ischemia-reperfusion in vitro. According to the literature, the concentration range of danshansu sodium is 0.5–80 mg /L (360 µM), and in this concentration range, danshansu sodium can play the best therapeutic effect, with higher safety (35,36). To understand the toxic effect of sodium Danshensu, we cultured primary astrocytes with different doses of sodium Danshensu (0, 25, 50, and 100 µM) for 24 hours, and assessed the cell viability and LDH production, which revealed that sodium Danshensu did not affect the cell cytotoxicity.
A Previous study has demonstrated that ischemic stroke induces neural cell injury and promotes apoptosis (29). To understand whether sodium Danshensu protect astrocytes injury in OGD/R-induced ischemic model, we studied the effect of sodium Danshensu on an OGD/R model. The cell viability was markedly decreased, while LDH release, caspase-3 activity, and apoptosis were considerably enhanced in the model group. Importantly, sodium Danshensu dose-dependently increased cell viability, reduced apoptosis, caspase-3 activity, and LDH production. Besides, sodium Danshensu lowered the secretion of ischemia-associated cytokines, such as TNF-α, IL-1β, and IL-6, which are usually upregulated in stroke and promote tissue injury (37). Moreover, sodium Danshensu showed a dose-dependent decrease in protein and mRNA expression of NLRP3 and HMGB1. NLRP3 inflammasome can be stimulated by a variety of molecules and events, such as reactive oxygen species, mitochondrial dysfunction, etc. (38). NLRP3 inflammasome can be induced and activated in cerebral arterial thrombosis, promoting brain cell death and playing an important role in brain cell injury. Inhibition of NLRP3 inflammasome activation improves cerebral ischemia outcome (39,40). Many traditional Chinese medicine components also regulate the activation of NLRP3 inflammasome. Currently, more than 20 traditional Chinese medicine monomers such as resveratrol, colchicine and chrysophanol are known to inhibit the formation of NLRP3 inflammasome and play a therapeutic role in ischemic stroke (41). Also, HMGB1 has an important function in ischemic stroke in that it activates innate immunity and promotes proinflammatory cytokine release. Besides, HMGB1 can activate the NLRP3 inflammasome and further promote neural injury (39,42). Additionally, the p-p65 protein and p-p65/p65 ratio were considerably reduced, which are usually upregulated after ischemia and tend to cooperate with cellular injury (43). These results suggest that sodium Danshensu can protect OGD/R-induced primary astrocyte injury via modulating the NLRP3 inflammasome.
Autophagy is a catabolic process in which cells eliminate destroyed impaired proteins and organelles via lysosome-dependent pathway to preserve the homeostatic balance (44). Autophagy plays controversial roles in ischemic brain injury (15). According to increasing evidence, autophagy has a protective effect in ischemic stroke (25). Importantly, activation of autophagy reduces inflammation and improves overall survival in rat models of cerebral ischemia (45,46). Therefore, modulating autophagy may be beneficial in preventing or treating ischemic stroke.
In recent years, many techniques and markers have been established for determining autophagy in cells and animals (37). Notably, Beclin 1 and LC3 are essential autophagy-related factors. In vitro astrocyte autophagy is indicated by the levels of autophagic substrate p62, and autophagy-associated protein of LC3-II, Beclin-1 expression (14,47). Our study found that sodium Danshensu dose-dependently upregulated Beclin 1 expression in primary astrocytes, reduced P62 protein expression, and further increased LC3B-II/LC3B-I ratio compared with the OGD/R group. To further elucidate the role of sodium Danshensu on astrocytes autophagy, we studied its effect on TSC2 and the mTOR signaling pathway, which is highly linked to autophagy. While TSC2 regulates the phosphorylation of the mTOR, which controls autophagy, TSC2 is now widely acknowledged to have a function in brain ischemia-reperfusion injury (34,48).
Interestingly, we noticed sodium Danshensu dose-dependently increased TSC2 protein expression and significantly reduced p-mTOR protein expression and p-mTOR/mTOR ratio in astrocytes compared with the OGD/R group. However, the TSC2 protein in the control and OGD/R groups was similar. Altogether, our results suggested that sodium Danshensu mediated neuroprotection, which may be due to sodium Danshensu-induced astrocyte autophagy via modulating the TSC2/mTOR pathway. Therefore, in the following experiments, we can conduct in vivo experiments and use danshensu sodium to treat cerebral ischemia-reperfusion mice, so as to further explore the therapeutic effect of danshensu sodium on ischemic stroke.
Conclusions
In conclusion, our study provides experimental evidence for the protective effect of sodium Danshensu on cerebral OGD/R injury. It inhibits OGD/R-mediated astrocyte injury through modulating the NLRP3 inflammasome and TSC2/mTOR pathways. The present findings further our understanding of sodium Danshensu’s neuroprotective effect and its underlying mechanism in ischemic stroke.
Acknowledgments
Funding: This work was supported by Science and Technology Research Project of Education Department of Jiangxi Province (No. GJJ210143); Science and Technology Research Project of Education Department of Jiangxi Province (No. GJJ210141); and Science and Technology Project of Jiangxi Provincial Health Commission (No. 20185077).
Footnote
Reporting Checklist: The authors have completed the MDAR reporting checklist. Available at https://atm.amegroups.com/article/view/10.21037/atm-22-2143/rc
Data Sharing Statement: Available at https://atm.amegroups.com/article/view/10.21037/atm-22-2143/dss
Conflicts of Interest: All authors have completed the ICMJE uniform disclosure form (available at https://atm.amegroups.com/article/view/10.21037/atm-22-2143/coif). The authors have no conflicts of interest to declare.
Ethical Statement: The authors are accountable for all aspects of the work in ensuring that questions related to the accuracy or integrity of any part of the work are appropriately investigated and resolved. Experiment was performed under a project license (No. CDYFY-IACUC-202208QR027) granted by ethics board of the First Affiliated Hospital of Nanchang University, in compliance with the First Affiliated Hospital of Nanchang University’s guidelines for the care and use of animals.
Open Access Statement: This is an Open Access article distributed in accordance with the Creative Commons Attribution-NonCommercial-NoDerivs 4.0 International License (CC BY-NC-ND 4.0), which permits the non-commercial replication and distribution of the article with the strict proviso that no changes or edits are made and the original work is properly cited (including links to both the formal publication through the relevant DOI and the license). See: https://creativecommons.org/licenses/by-nc-nd/4.0/.
References
- Wei ZZ, Chen D, Liu LP, et al. Enhanced Neurogenesis and Collaterogenesis by Sodium Danshensu Treatment After Focal Cerebral Ischemia in Mice. Cell Transplant 2018;27:622-36. [Crossref] [PubMed]
- Zhang J, Zhang Q, Liu G, et al. Therapeutic potentials and mechanisms of the Chinese traditional medicine Danshensu. Eur J Pharmacol 2019;864:172710. [Crossref] [PubMed]
- Wu B, Liu M, Zhang S. Dan Shen agents for acute ischaemic stroke. Cochrane Database Syst Rev 2007;CD004295. [Crossref] [PubMed]
- Ai F, Chen M, Li W, et al. Danshen improves damaged cardiac angiogenesis and cardiac function induced by myocardial infarction by modulating HIF1α/VEGFA signaling pathway. Int J Clin Exp Med 2015;8:18311-8. [PubMed]
- Yin Q, Lu H, Bai Y, et al. A metabolite of Danshen formulae attenuates cardiac fibrosis induced by isoprenaline, via a NOX2/ROS/p38 pathway. Br J Pharmacol 2015;172:5573-85. [Crossref] [PubMed]
- Bao XY, Zheng Q, Tong Q, et al. Danshensu for Myocardial Ischemic Injury: Preclinical Evidence and Novel Methodology of Quality Assessment Tool. Front Pharmacol 2018;9:1445. [Crossref] [PubMed]
- Lam FF, Yeung JH, Chan KM, et al. Relaxant effects of danshen aqueous extract and its constituent danshensu on rat coronary artery are mediated by inhibition of calcium channels. Vascul Pharmacol 2007;46:271-7. [Crossref] [PubMed]
- Wang W, Li SS, Xu XF, et al. Danshensu alleviates pseudo-typed SARS-CoV-2 induced mouse acute lung inflammation. Acta Pharmacol Sin 2022;43:771-80. [Crossref] [PubMed]
- MEIm XD. Danshen: a phytochemical and pharmacological overview. Chin J Nat Med 2019;17:59-80. [PubMed]
- Liu G, Zhang Q, Zhang J, et al. Preventive but nontherapeutic effect of danshensu on hypoxic pulmonary hypertension. J Int Med Res 2020;48:300060520914218. [Crossref] [PubMed]
- Jia J, Mo X, Liu J, et al. Mechanism of danshensu-induced inhibition of abnormal epidermal proliferation in psoriasis. Eur J Pharmacol 2020;868:172881. [Crossref] [PubMed]
- Antman EM, Braunwald E. Managing Stable Ischemic Heart Disease. N Engl J Med 2020;382:1468-70. [Crossref] [PubMed]
- Piepoli MF, Hoes AW, Agewall S, et al. 2016 European Guidelines on cardiovascular disease prevention in clinical practice: The Sixth Joint Task Force of the European Society of Cardiology and Other Societies on Cardiovascular Disease Prevention in Clinical Practice (constituted by representatives of 10 societies and by invited experts)Developed with the special contribution of the European Association for Cardiovascular Prevention & Rehabilitation (EACPR). Eur Heart J 2016;37:2315-81. [Crossref] [PubMed]
- Wang G, Wang T, Zhang Y, et al. Schizandrin Protects against OGD/R-Induced Neuronal Injury by Suppressing Autophagy: Involvement of the AMPK/mTOR Pathway. Molecules 2019;24:3624. [Crossref] [PubMed]
- Wang P, Shao BZ, Deng Z, et al. Autophagy in ischemic stroke. Prog Neurobiol 2018;163-164:98-117. [Crossref] [PubMed]
- Henderson SJ, Weitz JI, Kim PY. Fibrinolysis: strategies to enhance the treatment of acute ischemic stroke. J Thromb Haemost 2018;16:1932-40. [Crossref] [PubMed]
- Xiong X, Borrelli F, de Sá Ferreira A, et al. Herbal medicines for cardiovascular diseases. Evid Based Complement Alternat Med 2014;2014:809741. [Crossref] [PubMed]
- Zhang QZ, Fu TT, Dai JN, et al. Sodium Danshensu promotes the healing of stage 2 pressure injury wounds in ischemia/reperfusion injury rat models: possible regulation of apoptosis and inflammatory response. J Tradit Chin Med 2021;41:571-80. [PubMed]
- Freeman MR, Rowitch DH. Evolving concepts of gliogenesis: a look way back and ahead to the next 25 years. Neuron 2013;80:613-23. [Crossref] [PubMed]
- Hanani M, Verkhratsky A. Satellite Glial Cells and Astrocytes, a Comparative Review. Neurochem Res 2021;46:2525-37. [Crossref] [PubMed]
- Alluri H, Anasooya Shaji C, Davis ML, et al. Oxygen-glucose deprivation and reoxygenation as an in vitro ischemia-reperfusion injury model for studying blood-brain barrier dysfunction. J Vis Exp 2015;e52699. [Crossref] [PubMed]
- Zheng L, Cheng W, Wang X, et al. Overexpression of MicroRNA-145 Ameliorates Astrocyte Injury by Targeting Aquaporin 4 in Cerebral Ischemic Stroke. Biomed Res Int 2017;2017:9530951. [Crossref] [PubMed]
- Choudhury GR, Ding S. Reactive astrocytes and therapeutic potential in focal ischemic stroke. Neurobiol Dis 2016;85:234-44. [Crossref] [PubMed]
- Pekny M, Wilhelmsson U, Tatlisumak T, et al. Astrocyte activation and reactive gliosis-A new target in stroke? Neurosci Lett 2019;689:45-55. [Crossref] [PubMed]
- Liu X, Tian F, Wang S, et al. Astrocyte Autophagy Flux Protects Neurons Against Oxygen-Glucose Deprivation and Ischemic/Reperfusion Injury. Rejuvenation Res 2018;21:405-15. [Crossref] [PubMed]
- Iwata-Ichikawa E, Kondo Y, Miyazaki I, et al. Glial cells protect neurons against oxidative stress via transcriptional up-regulation of the glutathione synthesis. J Neurochem 1999;72:2334-44. [Crossref] [PubMed]
- Yang G, Wang Y, Zeng Y, et al. Rapid health transition in China, 1990-2010: findings from the Global Burden of Disease Study 2010. Lancet 2013;381:1987-2015. [Crossref] [PubMed]
- Kim J, Thayabaranathan T, Donnan GA, et al. Global Stroke Statistics 2019. Int J Stroke 2020;15:819-38. [Crossref] [PubMed]
- Stary CM, Giffard RG. Advances in astrocyte-targeted approaches for stroke therapy: an emerging role for mitochondria and microRNAS. Neurochem Res 2015;40:301-7. [Crossref] [PubMed]
- Wang T, Li C, Han B, et al. Neuroprotective effects of Danshensu on rotenone-induced Parkinson's disease models in vitro and in vivo. BMC Complement Med Ther 2020;20:20. [Crossref] [PubMed]
- Wang J, Fan K, He C, et al. A novel Danshensu/tetramethylpyrazine protects against Myocardial Ischemia Reperfusion Injury in rats. Int J Med Sci 2021;18:2716-24. [Crossref] [PubMed]
- Wang T, Fu F, Han B, et al. Danshensu ameliorates the cognitive decline in streptozotocin-induced diabetic mice by attenuating advanced glycation end product-mediated neuroinflammation. J Neuroimmunol 2012;245:79-86. [Crossref] [PubMed]
- Teng MC, Wu PC, Lin SP, et al. Danshensu Decreases UVB-Induced Corneal Inflammation in an Experimental Mouse Model via Oral Administration. Curr Eye Res 2018;43:27-34. [Crossref] [PubMed]
- Zhu C, Zhou Q, Luo C, et al. Dexmedetomidine Protects Against Oxygen-Glucose Deprivation-Induced Injury Through Inducing Astrocytes Autophagy via TSC2/mTOR Pathway. Neuromolecular Med 2020;22:210-7. [Crossref] [PubMed]
- Zheng Y, Pan C, Chen M, et al. miR-29a ameliorates ischemic injury of astrocytes in vitro by targeting the water channel protein aquaporin 4. Oncol Rep 2019;41:1707-17. [Crossref] [PubMed]
- Wang J, Ma Z, Wang W, et al. Comparative pharmacokinetic study of sodium Danshensu and Salvia miltiorrhiza injection in rat. Zhongguo Zhong Yao Za Zhi 2009;34:2943-5. [PubMed]
- Lambertsen KL, Biber K, Finsen B. Inflammatory cytokines in experimental and human stroke. J Cereb Blood Flow Metab 2012;32:1677-98. [Crossref] [PubMed]
- Kelley N, Jeltema D, Duan Y, et al. The NLRP3 Inflammasome: An Overview of Mechanisms of Activation and Regulation. Int J Mol Sci 2019;20:3328. [Crossref] [PubMed]
- Espinosa-Garcia C, Atif F, Yousuf S, et al. Progesterone Attenuates Stress-Induced NLRP3 Inflammasome Activation and Enhances Autophagy following Ischemic Brain Injury. Int J Mol Sci 2020;21:3740. [Crossref] [PubMed]
- Ye Y, Jin T, Zhang X, et al. Meisoindigo Protects Against Focal Cerebral Ischemia-Reperfusion Injury by Inhibiting NLRP3 Inflammasome Activation and Regulating Microglia/Macrophage Polarization via TLR4/NF-κB Signaling Pathway. Front Cell Neurosci 2019;13:553. [Crossref] [PubMed]
- Tao YW, Yang L, Chen SY, et al. Pivotal regulatory roles of traditional Chinese medicine in ischemic stroke via inhibition of NLRP3 inflammasome. J Ethnopharmacol 2022;294:115316. [Crossref] [PubMed]
- Frank MG, Weber MD, Watkins LR, et al. Stress sounds the alarmin: The role of the danger-associated molecular pattern HMGB1 in stress-induced neuroinflammatory priming. Brain Behav Immun 2015;48:1-7. [Crossref] [PubMed]
- Li SJ, Cui KF, Fu JJ, et al. EPO promotes axonal sprouting via upregulating GDF10. Neurosci Lett 2019;711:134412. [Crossref] [PubMed]
- Mo Y, Sun YY, Liu KY. Autophagy and inflammation in ischemic stroke. Neural Regen Res 2020;15:1388-96. [Crossref] [PubMed]
- Zhu J, Liu K, Huang K, et al. Metformin Improves Neurologic Outcome Via AMP-Activated Protein Kinase-Mediated Autophagy Activation in a Rat Model of Cardiac Arrest and Resuscitation. J Am Heart Assoc 2018;7:008389. [Crossref] [PubMed]
- Wei H, Yin M, Lu Y, et al. Mild hypothermia improves neurological outcome in mice after cardiopulmonary resuscitation through Silent Information Regulator 1-actviated autophagy. Cell Death Discov 2019;5:129. [Crossref] [PubMed]
- Frugier T, Taylor JM, McLean C, et al. Evidence for the recruitment of autophagic vesicles in human brain after stroke. Neurochem Int 2016;96:62-8. [Crossref] [PubMed]
- Hayakawa K, Esposito E, Wang X, et al. Transfer of mitochondria from astrocytes to neurons after stroke. Nature 2016;535:551-5. [Crossref] [PubMed]
(English Language Editor: J. Jones)