Tumor heterogeneity and the dilemma of antioxidant therapies in cancer
Intra-tumor heterogeneity (ITH) consists of subpopulations of cancer cells within a tumor with different phenotypic, genetic and behavioral characteristics, whose origin is suggested by two distinct, but not exclusive, hypotheses: the cancer stem cell (CSC) and clonal evolution/selection hypotheses. Clonal evolution proposes a speciation by natural selection and a continued accumulation of genetic and epigenetic aberrations resulting in a sequential selection of diverse subpopulation with more aggressive phenotypes. On the other hand, the CSC hypothesis denotes that cells of a tumor have the same potential to promote tumor progression, and ITH arises as a consequence of the hierarchical organization of cancer cells. Therefore, CSC may be differentiated into non-CSC phenotypes, which originates several subpopulations with a new set of markers (1,2).
ITH is driven by genetic and epigenetic changes in tumor cells. Genomic instability added to selective pressures, such as transformations in the microenvironment and hormonal therapeutic interventions, can generate aberrations such as translocations, deletions and point mutations, among others, to create ITH. Regarding epigenetic regulation, ITH results of changes in genome function without a detectable modification in DNA sequence (2). It is important to emphasize that the cellular ability to react via genetic and epigenetic reprogramming requires high plasticity and may change according to their stage in metastasis and tumor progression. Also, plasticity determines how tumoral subpopulations adapt to stress conditions, which include physical, chemical and oxidative stress, to generate favorable or unfavorable outcomes to cell fate.
Breast cancer (BC) cells are overall highly plastic and react to genetic and epigenetic reprogramming, changing according to their interaction with stromal cells and constantly adapting to new environmental conditions, thus illustrating a heterogeneous type of cancer. BC can express estrogen and progesterone receptors (ER and PR), different Ki67 proliferation index and HER2 expressions. Depending on the particular combination of these components, BC are grouped in Luminal-A (ER+ and/or PR+, Ki67 <20, HER2−), Luminal B− (ER+ and/or PR+, Ki67 ≥20, HER2−), Luminal B+ (ER+ and/or PR+, HER2+), HER2+ non-Luminal (ER−, PR−, HER2+), and triple-negative (ER−, PR−, HER2−) (3). Alternative classifications based at a transcriptomic level also exist, for example for triple negative BC: basal-like 1, basal-like 2, immunomodulatory, mesenchymal, mesenchymal stem-like and luminal androgen receptor subtype (4). Besides differences among individual patients, called inter-tumor heterogeneity, BC also displays ITH, comprising different subpopulations inside primary tumors, between primary and metastatic lesions, and within metastatic lesions (3).
While it is undeniable that genetic and epigenetic factors directly affect ITH, tumors comprise not only tumor cells but a combination of other components, such as soluble factors, extracellular matrix components, and immune, vascular and stromal cells. This intricate cellular network creates a complex tumor niche or tumor microenvironment (TME), whose great adaptability to distinct environmental conditions brings multiple obstacles towards therapy efficacy during tumor progression (1,3,5,6). In fact, there is accumulating evidence that many components of the tumor niche may drive tumor heterogeneity, as well as extrinsic microenvironmental factors such as hypoxia (1,7).
Hypoxia is one of the driving factors for triggering the Warburg effect, or the favoring of aerobic glycolysis and lactate synthesis for oxygen-independent energy production (8). First described in the late 1920’s as a divergence from the Pasteur effect, in which lactate production is inhibited by oxygen exposure, the Warburg effect interferes majorly with pyruvate metabolism and disfavor its entrance into the tricarboxylic acid (TCA) cycle (9,10). This leads to a couple of consequences: (I) induction of the HIF pathway and its subsequent transcription regulation of other pathways, such as the PI3K/AKT/mTOR and JAK2/STAT3 axis, by increasing glucose transporter expression, and (II) increase in ATP consumption while blocking the feedback inhibition of phosphofructokinase (PFK), therefore inhibiting the main pathway for oxidative phosphorylation (9).
BC heterogeneity was addressed by Ren and colleagues in early 2022 from the perspective of metabolic plasticity, through investigation of the effects of the antioxidant glutathione peroxidase 2 (GPx2) loss in BC (6). By single cell RNA analysis, they demonstrated that GPx2 loss increased reactive oxygen species (ROS), with consequent activation of the ROS/HIF-1α/VEGF axis, resulting in abnormal angiogenesis and augmented hypoxia through stabilization of HIF-1α. This condition primed cells to a metabolic heterogeneity depicted by observation of the Warburg effect in most tumor cell populations, except for one cluster that maintained its oxidative phosphorylation in parallel to glycolysis (Figure 1).
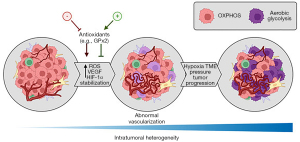
In general, the authors postulate that tumor cells may survive under aerobic or hypoxic conditions by assuming a hybrid metabolic state after the disruption of ROS levels within the cells, which may be stimulated by HIF-1α stabilization. While the reasons for this selection are not clear, the authors indicate that GPx2 loss may have a key role in generating tumor cell heterogeneity and in tumor progression. These results, however, diverge in different cancer types and stages of tumoral progression, which opens the discussion of whether a hybrid metabolic state may be advantageous for tumor cells to survive and develop resistance to treatments targeting the aerobic or glycolytic metabolism.
GPx2 is an antioxidant enzyme that catalyzes the decomposition of hydrogen peroxide (H2O2) by the oxidation of glutathione (GSH), resulting in oxidized glutathione (GSSG) and H2O. Considering the large energetic demands of highly proliferative cancer cells, mitochondrial oxidative phosphorylation generates high levels of ROS that leads to oxidative stress, which is why antioxidant enzymes are often associated with cytotoxicity prevention. Several classes of intracellular enzymatic antioxidants are available and may be altered in carcinogenesis (11). Therefore, GPx2 upregulation could be considered as a predictor of prognosis and response to cytotoxic treatments, benefiting tumor cells over healthy tissues and impairing responses to treatments that may cause oxidative damage (12).
Although logic suggests that antioxidants are a good anticancer strategy, Ren and collaborators demonstrated that GPx2 loss stimulated tumor progression, mainly due to hypoxic signaling, abnormal vascularization and shift to aerobic glycolysis/OXPHOS state. Indeed, the high complexity of GPx2 high/low expression depends on specific metabolic pathways and microenvironment conditions such as hyperproliferation-derived hypoxia (13). Additional data suggests that ROS has a dual role in cancer progression, acting both as main biochemical entity and as a secondary messenger, which shows yet another layer of complexity in antioxidant therapy efficiency (11). Table 1 summarizes the complexity and duality of GPx2 in different types of cancers.
Table 1
Tumor type | GPx2 status | Effect | Assay | Ref. |
---|---|---|---|---|
Colon carcinoma | ↓ | Increase tumor progression | Mouse model of inflammation-associated colon carcinogenesis | (14) |
Pancreas cancer | ↓ | Suppression of proliferation, invasion and metastasis | Gene expression in patient samples | (15) |
Urinary tract and bladder | ↓ | Poor prognosis | Gene expression in patient samples | (16) |
Urinary bladder | ↓ | Cancer invasion | Gene expression in patient samples | (17) |
Esophageal carcinoma | ↓ | Poor prognosis | Gene expression in patient samples | (18) |
BC | ↓ | Tumor progression and heterogeneity | Mouse model of BC with GPx2 silenced cells | (6) |
Colon carcinoma | ↓ | Increase inflammation | Stable knockdown of GPx2 in HT-29 cells | (19) |
Cutaneous squamous cell carcinomas | ↓ | Tumor initiation | Cell lines and mouse model | (20) |
Hepatocellular carcinoma | ↑ | Tumor metastasis | Cell lines and mouse model | (21) |
Lung carcinoma | ↑ | Tumor initiation and cisplatin resistance | Gene expression in lung carcinoma cell line | (22) |
Hepatocellular carcinoma | ↑ | Poor prognosis | Gene expression in patient samples | (23) |
Colorectal cancer | ↑ | Early tumor recurrence | Patient-derived colonosphere cultures | (24) |
Gastric carcinoma | ↑ | Prognostic factor in primary tumor and metastatic foci | Gene expression in patient samples | (25) |
BC, breast cancer.
While ITH is still not fully understood, it might facilitate the natural evolution of cancer and interfere with the effectiveness of anti-cancer treatment, due to either the selection or the induction of new resistant sub-clones upon treatment (7). Ren and collaborators indicate that, while tumor cells generally trigger the Warburg effect to favor glycolysis over oxidative phosphorylation, not all subpopulations follow this tendency (6). In a different study, the differential expression of 305 genes was found in invasive BC cells compared to non-invasive cells, featuring multiple biological roles from extracellular matrix (ECM) reorganization to modulation of the immune response and Rho signaling (26). However, the identification of ITH is still challenging, and while single-cell analysis may reveal how the transitioning between metabolic states occurs, this technology is far from being globally widespread (8).
Additionally, ITH may also affect both hormone-based and antibody-drug conjugate therapies, having been described to interfere with Fulvestran or Tamoxifen effectiveness (4). Immunohistochemistry (IHC) analysis of BC samples from clinical trials revealed that patients with heterogeneous tumors had different recurrence-free survival (DRFS) than those with homogeneous diseases. Differences in ER and PR percentage within tumor biopsy via staining were suggested as prognostic of DRFS (4,27).
In a distinct study, the differential expression of PDL-1 in >1% of tumor area is sufficient to increase patient survival upon Atezolizumab and Nab-Paclitaxel therapy (4). Interestingly, another study identified a better prognosis for HER-2 positive invasive ductal BC patients with a higher degree of heterogeneity when compared to patients with progressive disease. Authors attributed this peculiar behavior to proteins involved in immune processes and hemostasis, suggesting the high ITH would be associated with increased lymphocyte infiltration, facilitating the response to treatment (7).
Considering the variety of responses to ROS-mediated processes due to ITH establishment and the generation of subpopulations with distinct adaptations, are antioxidant-based treatments a double-edged sword for cancer therapy? Is there a specific condition in tumor tissues responsible for the different clinical outcomes regarding antioxidant therapies? The answers to these questions are still under debate, considering the paradoxical relationship between cancer development and increased ROS levels, as well as the consequences observed in cell metabolism and heterogeneity stimulation.
Acknowledgments
Funding: This work was supported by the São Paulo Research Foundation (FAPESP) under grants (Nos. 2021/01983-4, 2019/05149-9, and 2019/11437-7); Coordenação de Aperfeiçoamento de Pessoal de Nível Superior (CAPES) and Conselho Nacional de Desenvolvimento Científico e Tecnológico (CNPq) under grant (No. 429235/2018-6).
Footnote
Provenance and Peer Review: This article was commissioned by the editorial office, Annals of Translational Medicine. The article did not undergo external peer review.
Conflicts of Interest: All authors have completed the ICMJE uniform disclosure form (available at https://atm.amegroups.com/article/view/10.21037/atm-22-4219/coif). The authors have no conflicts of interest to declare.
Ethical Statement: The authors are accountable for all aspects of the work in ensuring that questions related to the accuracy or integrity of any part of the work are appropriately investigated and resolved.
Open Access Statement: This is an Open Access article distributed in accordance with the Creative Commons Attribution-NonCommercial-NoDerivs 4.0 International License (CC BY-NC-ND 4.0), which permits the non-commercial replication and distribution of the article with the strict proviso that no changes or edits are made and the original work is properly cited (including links to both the formal publication through the relevant DOI and the license). See: https://creativecommons.org/licenses/by-nc-nd/4.0/.
References
- Esparza-López J, Escobar-Arriaga E, Soto-Germes S, et al. Breast Cancer Intra-Tumor Heterogeneity: One Tumor, Different Entities. Rev Invest Clin 2017;69:66-76. [Crossref] [PubMed]
- Martelotto LG, Ng CK, Piscuoglio S, et al. Breast cancer intra-tumor heterogeneity. Breast Cancer Res 2014;16:210. [Crossref] [PubMed]
- Fumagalli C, Barberis M. Breast Cancer Heterogeneity. Diagnostics (Basel) 2021;11:1555. [Crossref] [PubMed]
- Baliu-Piqué M, Pandiella A, Ocana A. Breast Cancer Heterogeneity and Response to Novel Therapeutics. Cancers (Basel) 2020;12:3271. [Crossref] [PubMed]
- Lüönd F, Tiede S, Christofori G. Breast cancer as an example of tumour heterogeneity and tumour cell plasticity during malignant progression. Br J Cancer 2021;125:164-75. [Crossref] [PubMed]
- Ren Z, Liang H, Galbo PM Jr, et al. Redox signaling by glutathione peroxidase 2 links vascular modulation to metabolic plasticity of breast cancer. Proc Natl Acad Sci U S A 2022;119:e2107266119. [Crossref] [PubMed]
- Gawin M, Kurczyk A, Niemiec J, et al. Intra-Tumor Heterogeneity Revealed by Mass Spectrometry Imaging Is Associated with the Prognosis of Breast Cancer. Cancers (Basel) 2021;13:4349. [Crossref] [PubMed]
- Kondo H, Ratcliffe CDH, Hooper S, et al. Single-cell resolved imaging reveals intra-tumor heterogeneity in glycolysis, transitions between metabolic states, and their regulatory mechanisms. Cell Rep 2021;34:108750. [Crossref] [PubMed]
- Bensinger SJ, Christofk HR. New aspects of the Warburg effect in cancer cell biology. Semin Cell Dev Biol 2012;23:352-61. [Crossref] [PubMed]
- Warburg O, Wind F, Negelein E. The metabolism of tumors in the body. J Gen Physiol 1927;8:519-30. [Crossref] [PubMed]
- Luo X, Cheng C, Tan Z, et al. Emerging roles of lipid metabolism in cancer metastasis. Mol Cancer 2017;16:76. [Crossref] [PubMed]
- Jardim BV, Moschetta MG, Leonel C, et al. Glutathione and glutathione peroxidase expression in breast cancer: an immunohistochemical and molecular study. Oncol Rep 2013;30:1119-28. [Crossref] [PubMed]
- Ray PD, Huang BW, Tsuji Y. Reactive oxygen species (ROS) homeostasis and redox regulation in cellular signaling. Cell Signal 2012;24:981-90. [Crossref] [PubMed]
- Brigelius-Flohé R, Kipp AP. Physiological functions of GPx2 and its role in inflammation-triggered carcinogenesis. Ann N Y Acad Sci 2012;1259:19-25. [Crossref] [PubMed]
- Li F, Dai L, Niu J. GPX2 silencing relieves epithelial-mesenchymal transition, invasion, and metastasis in pancreatic cancer by downregulating Wnt pathway. J Cell Physiol 2020;235:7780-90. [Crossref] [PubMed]
- Chang IW, Lin VC, Hung CH, et al. GPX2 underexpression indicates poor prognosis in patients with urothelial carcinomas of the upper urinary tract and urinary bladder. World J Urol 2015;33:1777-89. [Crossref] [PubMed]
- Minato A, Noguchi H, Ohnishi R, et al. Reduced Expression Level of GPX2 in T1 Bladder Cancer and its Role in Early-phase Invasion of Bladder Cancer. In Vivo 2021;35:753-9. [Crossref] [PubMed]
- Lei Z, Tian D, Zhang C, et al. Clinicopathological and prognostic significance of GPX2 protein expression in esophageal squamous cell carcinoma. BMC Cancer 2016;16:410. [Crossref] [PubMed]
- Banning A, Florian S, Deubel S, et al. GPx2 counteracts PGE2 production by dampening COX-2 and mPGES-1 expression in human colon cancer cells. Antioxid Redox Signal 2008;10:1491-500. [Crossref] [PubMed]
- Walshe J, Serewko-Auret MM, Teakle N, et al. Inactivation of glutathione peroxidase activity contributes to UV-induced squamous cell carcinoma formation. Cancer Res 2007;67:4751-8. [Crossref] [PubMed]
- Suzuki S, Pitchakarn P, Ogawa K, et al. Expression of glutathione peroxidase 2 is associated with not only early hepatocarcinogenesis but also late stage metastasis. Toxicology 2013;311:115-23. [Crossref] [PubMed]
- Du H, Chen B, Jiao NL, et al. Elevated Glutathione Peroxidase 2 Expression Promotes Cisplatin Resistance in Lung Adenocarcinoma. Oxid Med Cell Longev 2020;2020:7370157. [Crossref] [PubMed]
- Liu T, Kan XF, Ma C, et al. GPX2 overexpression indicates poor prognosis in patients with hepatocellular carcinoma. Tumour Biol 2017;39:1010428317700410. [Crossref] [PubMed]
- Emmink BL, Laoukili J, Kipp AP, et al. GPx2 suppression of H2O2 stress links the formation of differentiated tumor mass to metastatic capacity in colorectal cancer. Cancer Res 2014;74:6717-30. [Crossref] [PubMed]
- Liu D, Sun L, Tong J, et al. Prognostic significance of glutathione peroxidase 2 in gastric carcinoma. Tumour Biol 2017;39:1010428317701443. [Crossref] [PubMed]
- Aw Yong KM, Ulintz PJ, Caceres S, et al. Heterogeneity at the invasion front of triple negative breast cancer cells. Sci Rep 2020;10:5781. [Crossref] [PubMed]
- Saha A, Harowicz MR, Cain EH, et al. Intra-tumor molecular heterogeneity in breast cancer: definitions of measures and association with distant recurrence-free survival. Breast Cancer Res Treat 2018;172:123-32. [Crossref] [PubMed]