A narrative review on the association of high intraocular pressure and glaucoma in patients with retinal vein occlusion
Introduction
Retinal vein occlusion (RVO) is the second most common retinal vascular disorder. It is classified into branch retinal vein occlusion (BRVO), central retinal vein occlusion (CRVO) (Figure 1), and hemi-retinal vein occlusion (HRVO) based on the zone of retinal vein drainage (1,2). CRVO and HRVO can be ischemic or non-ischemic with distinctive clinical manifestations and prognoses, and BRVO can be further divided into major and macular BRVO (3). Degree of vision loss, presence or absence of relative afferent pupillary defect (APD) and retinal capillary non-perfusion on fluorescein angiography (FA) indicates the level of ischemia (2).
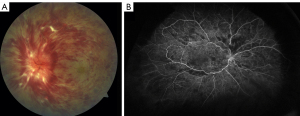
The association between RVO and high intraocular pressure (IOP)/glaucoma has been broadly described (3-6). Glaucoma is a leading cause of irreversible blindness worldwide and number of individuals with glaucoma is expected to increase from 76 million in 2020 to 111.8 million in 2040 (7-9). Elevated IOP is one of the most well-known and treatable targets in the management of glaucoma, a leading cause of retinal ganglion cell loss due to retinal ischemia (10). Identifying the role of glaucoma as a causative factor in the pathophysiology of different forms of ischemic and non-ischemic RVO in patients with pre-existing glaucoma and clarifying the pathophysiology of this association may facilitate the development of novel approaches for the management and modification of RVO patient outcomes.
In this review, we discuss the impact of high IOP and glaucoma on RVO and the importance of considering high IOP or pre-existing glaucoma during the management of RVO. We present the following article in accordance with the Narrative Review reporting checklist (available at https://atm.amegroups.com/article/view/10.21037/atm-22-2730/rc).
Methods
As summarized in Table 1, relevant manuscripts were searched and collected in the PubMed and Medline databases until May 2022 using the following keywords: “retinal vein occlusion”, “branch retinal vein occlusion”, “central retinal vein occlusion”, “hemi-retinal vein occlusion”, “hemispheric retinal vein occlusion”, “ischemic retinal vein occlusion”, “non-ischemic retinal vein occlusion”, “high intraocular pressure”, “glaucoma”, and their combinations. The English abstract of non-English papers was used if available. No limitation on publication date was applied.
Table 1
Items | Specification |
---|---|
Date of search | May 25, 2022 |
Databases and other sources searched | PubMed and Medline databases |
Search terms used | “Retinal vein occlusion”, “branch retinal vein occlusion”, “central retinal vein occlusion”, “hemi-retinal vein occlusion”, “hemispheric retinal vein occlusion”, “ischemic retinal vein occlusion”, “non-ischemic retinal vein occlusion”, “high intraocular pressure”, “glaucoma”, and their combinations |
Timeframe | All the published papers until date (May 2022) of performing the search |
Inclusion and exclusion criteria | All papers were included. The English abstract of non-English papers was used. Non-English papers with no English abstract were excluded |
Selection process | The selection was performed by SJ and ARH |
Discussion
Pathophysiology of retinal vein occlusion
While the definitive pathobiology of RVO is still unclear, it is thought to be a consequence of a thrombotic event. In CRVO, the occlusion may occur at the lamina cribrosa or within the optic nerve posterior to the lamina cribrosa (11). In BRVO, it is thought that the arterial compression of the vein at the junction of arteriovenous crossings triggers thrombus formation (12). Following the occlusive event, the inflammation plays an important role in the pathophysiology, progression, and prognosis of RVO. Elevated level of cytokines, such as monocyte chemoattractant protein-1, interleukin (IL)-6 and 8, and vascular endothelial growth factor (VEGF), in the vitreous and aqueous of patients with RVO, especially those with CRVO and hemicentral RVO, indicate the relevant role of inflammation in RVO (13,14).
Association between retinal vein occlusion and glaucoma
The presence of large cup-to-disk ratio in RVO patients had raised the possibility of common vascular occlusive pathogenesis between RVO and glaucoma (15,16). In addition, there are reports with higher prevalence of glaucoma in patients with RVO compared to controls supporting the hypothesis of shared common vascular pathogenesis between RVO and glaucoma. However, this association is still controversial (17,18). One of the dilemmas in diagnosing glaucoma in patients with RVO is abnormalities in the retinal nerve fiber layer (RNFL) thicknesses secondary to the RVO and not glaucomatous damage. The disruption of inner retina circulation in RVO leads to RNFL thinning, which interfere with optic nerve head analysis of glaucoma. Instead, few studies selected IOP measurement as an index of glaucoma among RVO patients (15). In one of these retrospective observational studies, the prevalence of primary open angle glaucoma (POAG) was not significantly different in patients with BRVO than in those in the general population (15). However, there are other studies using a combination of methods to diagnose POAG in patients with RVO. In a nonrandomized comparative clinical trial, 674 consecutive patients with unilateral CRVO and HRVO with a normal fellow eye were enrolled (17). The prevalence of POAG (based on IOP more than 22 mmHg, visual field defect consistent with glaucoma, glaucomatous cupping of the optic disc) and ocular hypertension in that study were 9.9% and 16.2%, respectively, which was significantly higher than general population (17). In addition, in another prospective, observational study, 30 patients with unilateral RVO and 18 patients with RVO and POAG were evaluated for the potential association between optic disc head morphology and RVO. It has been shown that patients with RVO without POAG had higher cup area, a lower rim volume, and higher cup/disc ratio (19). New modalities such as optical coherence tomography angiography (OCT-A) has been recently utilized to assess peripapillary and optic nerve head perfusion, microvascular visualization, and microcirculation changes of the retina and choroid in glaucoma. OCT-A in glaucoma patients may reveal decreased optic disc perfusion and reduced density of blood vessels in the temporal or total area of optic nerve head which is related to the functional glaucomatous changes (20-22). The significant association between thinning of the RNFL in fellow eyes of RVO eyes and changes in the peripapillary microvascular parameters suggests a common pathway between RVO and glaucoma (20). In addition, increased retinal venous pressure, decreased ocular blood flow, lower peripapillary vessel density and perfusion density in the fellow eyes of RVO eyes are consistent with a common pathogenesis for glaucoma and RVO (20). Decreased RNFL thickness primarily in the superior and inferior temporal areas of the optic nerve head is associated with a higher risk of RVO (23).
The strong association between pre-existing glaucoma and the occurrence of RVO and the high frequency of secondary glaucoma in patients with RVO has been reported (3-6,24,25). In a prospective cross-sectional study, a detailed examination of 50 patients with RVO and POAG indicated a strong association between POAG and the occurrence of RVO (26). In population-based studies, Korean Health Insurance Review and Assessment data were evaluated over a three-year period to determine the incidence of POAG in individuals with the RVO compared to that in the general population (27,28). The results revealed the POAG incidence was 1,829.43 per 100,000 person/year among patients with RV, while this number was 233.98 per 100,000 person/year in the general population, which was statistically significant. The authors suggested that the close correlation between glaucoma development and the pathogenesis of RVO possibly occurred due to shared common vascular pathways and mechanical risk factors associated with the pathobiology of both RVO and glaucoma (27,28). In a prospective population-based study, the odds of incidental RVO among individuals with higher cup-disc ratio were 1.29-fold higher than in the control group (6). In this study, age, systolic blood pressure, diabetes, smoking status, and IOP were controlled as confounding factors (6). In a retrospective analysis of data from a randomized clinical trial evaluating 1,636 ocular hypertensive participants, a greater cup-disc ratio and aging were determined to be significant risk factors for the development of RVO (29). In a Korean nationwide and population-based 11-year longitudinal study, the risk for RVO development after POAG and the risk for POAG after RVO were assessed (30). The results revealed that POAG developed in 0.92% of the patients in the RVO group and RVO developed in 0.99% of the patients in the POAG group, indicating that RVO increases the risk for POAG (hazard ratio: 3.25) and vice versa (hazard ratio: 5.05). However, the effect of the comorbidities of diabetes, worse visual acuity and relative APD on presentation and hypertension also increased the risk for POAG development in patients with RVO (30,31). The increased risk for POAG following RVO may be confounded due to secondary steroid induced elevations in IOP associated with intravitreal corticosteroid injections for the treatment of macular edema in patients with RVO (32,33). Glaucoma in RVO patients can also be secondary to neovascularization of angle. A prospective, randomized, double-blind, sham-controlled study revealed that early treatment with anti-VEGF can decrease the risk for anterior segment neovascularization and neovascular glaucoma in patients with macular edema secondary to CRVO (34). Neovascular glaucoma developed among CRVO patients with pre-existing glaucoma, uncontrolled IOP and retinal non-perfusion status (35). In another study, the clinical characteristics of unilateral RVO among patients with glaucomatous and non-glaucomatous eyes were assessed (36). An association between RVO which involves optic disk without AV-crossing and glaucomatous changes in the fellow eye was observed; thus, careful monitoring for glaucoma among eyes with this feature was recommended (36). Furthermore, a retrospective, case-control study of 40 patients with unilateral BRVO revealed more rapid glaucoma progression in the fellow eyes without BRVO than in the eyes of the control patients (37). In addition, it has been reported that glaucoma is a significant risk factor for the development of ischemic CRVO and subsequent neovascular glaucoma and aggravates the visual outcome. However, early detection and treatment of ischemia as well as controlling the IOP may improve the final outcome (38).
Management strategies for retinal vein occlusion with and without pre-existing glaucoma
A thorough examination to assess the indications for treatment of RVO is critical. The presence and absence of elevated IOP, glaucoma, ocular neovascularization and macular edema should be evaluated by slit lamp examination with non-dilated gonioscopy and dilated fundoscopy (39). Optical coherence tomography (OCT) can be used to estimate the severity of the disease by detecting the presence of vitreoretinal interface irregularities, neurosensory detachment, loss of outer retinal integrity, macular edema and thickness of edema (40). More information about capillary non-perfusion, detection of subtle neovascularization and macular ischemia as well as distinguishing collateralization from neovascularization can be obtained using FA (Figure 1B and Figure 2). FA allows the visualization of peripheral capillary non-perfusion, neovascularization and collateralization arising from neovascularization, and macular ischemia, which may not be clinically detected by other means (26). According to the data regarding different disc areas of capillary non-perfusion in the Branch Vein Occlusion Study (BVOS) and the Central Vein Occlusion Studies (CVOS), RVO can be categorized as perfused, non-perfused or indeterminate (41,42). In addition, visual field testing, electrophysiology and the presence of an APD can be used to evaluate the perfusion status (3). The presence of an APD and a greater magnitude of capillary non-perfusion may increase the probability of vision loss in RVO patients. Managing RVO complications is critical and more important than re-establishing normal venous flow. RVO symptoms can be improved by bypassing the obstruction; however, treatments to resolve vein obstruction, including creating anastomosis with surgery, laser, thrombolytic use and optic nerve sheathotomy, have not been successful (39,43-46).
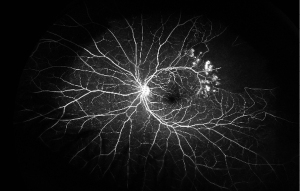
The cumulative prevalence of pre-existing POAG is a risk factor for RVO and it has been reported that the treatment of glaucoma prevents the progression of glaucoma and IOP elevation following RVO development over 3 years of follow-up (47). Angle closure is also a possible association with RVO specially in case of HRVO and CRVO. In a retrospective study of 19 patients with RVO, 75% of patients presented with RVO and diagnosed with primary angle-closure glaucoma in the same visit, and in 25% of cases the diagnosis of primary angle-closure glaucoma was delayed ranging from few months to 9 years after the diagnosis of RVO (48). In another retrospective analysis of data from a randomized clinical trial among cases with RVO and pre-existing glaucoma, the treatment with topical IOP-lowering medications to achieve the goal of IOP equal or less than 24 mmHg and a minimum 20% reduction from the baseline IOP was recommended (29). The results showed a higher incidence of RVO among cases who did not receive topical IOP-lowering medications compared to the medication group (29). IOP-lowering treatment and close monitoring of IOP in the treatment of macular edema due to RVO, in eyes with pre-existing glaucoma or ocular hypertension are also suggested (49).
Treatment options for CRVO and impact on secondary glaucoma
The BVOS and CVOS established a standard of care and treatment algorithm for patients with BRVO and CRVO to reduce vision loss and manage neovascular complications (41,42). Worse vision at presentation was reported among BRVO patients. Although a general improvement in vision was reported over time, this improvement has not been observed to exceed 20/40 (50). It has also been reported that more than a quarter of non-ischemic CRVO cases transformed into the ischemic form over time, and a quarter of these patients experienced advanced neovascular glaucoma within 15 months (41,42).
Macular edema as the most common complication of RVO can be improved by macular grid laser photocoagulation in individuals with 20/50 vision or worse; however, laser therapy does not improve the visual acuity (51). Pan retinal photocoagulation (PRP) can be used when neovascularization in the anterior segment, disc or retina is present and eyes with ischemic CRVO undergoing PRP shows less anterior segment neovascularization (3).
The role of platelet aggregation inhibitors and intravenous thrombolysis in improving vision outcomes among RVO patients has been studied (51). By lowering the hematocrit, hemodilution may improve the retinal flow; however, the exact function of antithrombotic agents is still unclear (2). The effects of low molecular weight heparin for the treatment of acute RVO have been reported to be superior to antiplatelet agents (52,53).
In a prospective randomized multicenter clinical trial, the beneficial effects of laser induced chorioretinal venous anastomosis among individuals with CRVO have been evaluated (44). With this method, the first spot of high-power argon or YAG laser ruptures the Bruch’s membrane and the second spot ruptures a major branch of the retinal vein to assist in the formation of an anastomosis in the retina; and choroidal circulation (44). A substantial drop in the retinal transit time of fluorescein after 18 months in patients treated with this approach has been reported. In addition, a mean improvement in visual acuity of 3.6 letters in the treatment group was demonstrated, while the control group experienced an 8.1-letter loss in visual acuity. Although 18.2% of the eyes treated in the treatment group required PRP due to the development of choroidal neovascularization, few patients in the treatment group developed ischemic CRVO (44).
Treatment options for BRVO and impact on glaucoma/IOP
The results of the BVOS showed that individuals with BRVO who received grid macular laser treatment gained a mean improvement of 1.33 letters over the 3 study years. The control group gained a mean improvement of 0.23 letters (42). A significant improvement in the visual acuity of treatment group compared to that in the control group has also been reported (42). It has been hypothesized that the grid laser method may lead to increased oxygen delivery to the inner retina. The autoregulatory mechanism in the inner retina can decrease capillary and vein hydrostatic pressure followed by reducing edema (54).
In a systematic review of all clinical trials related to BRVO, other treatment methods such as hemodilution, sheathotomy, pars plana vitrectomy and anti-thrombotic agents were evaluated (55). However, the lack of sufficient evidence for the efficacy of these approaches in the routine management of BRVO has limited their therapeutic application (2,55).
Corticosteroid therapy
Due to the known role of inflammatory mediators in the pathophysiology of RVO, off-label intravitreal corticosteroid (especially triamcinolone acetonide) has become a routine approach for the management of RVO. The short/intermediate efficacy of intravitreal triamcinolone has been reported in small scale studies (56,57); however, it has been shown that the efficacy of intravitreal triamcinolone is not maintained after 1 year of treatment (58). Regarding the timing of corticosteroid effectiveness, it has been reported that macular edema in RVO begins to resolve 1–6 hours post-intravitreal corticosteroid injection (59). In addition to the regulation of gene expression following the interaction between receptors and corticosteroids, a non-genomic pathway destabilizes mRNA in the cytoplasm (59). The Standard Care versus Corticosteroid for Retinal Vein Occlusion (SCORE) studies evaluated the efficacy of 1 and 4 mg intravitreal triamcinolone injection compared to the standard treatment (observation for macular edema in CRVO and grid laser photocoagulation for BRVO) (60,61). Although no significant differences in visual acuity arising from grid laser photocoagulation and intravitreal triamcinolone injection in BRVO patients after 1-year were observed (61), a quarter of the individuals with CRVO who received intravitreal triamcinolone injection had a 5-fold increase in likelihood to gain an improvement of 15 letters or more by the end of study compared the likelihood of those in the observation group to achieve such improvement (60). A 3-4-fold increase in the likelihood for IOP elevation after treatment with the higher dose of intravitreal triamcinolone injection was also reported. It has been reported that ocular hypertension following intravitreal steroids is probably less well tolerated by the optic nerve in glaucoma patients.
The phase III Global Evaluation of implantable dexamethasone in retinal VEIN occlusion (GENEVA) trial evaluating an FDA-approved biodegradable intravitreal dexamethasone implant (350 and 700 µg) for the treatment of macular edema in RVO cases showed that a higher number of patients gained an improvement of 15 letters or more in the 90 days following dexamethasone injection (62-64). However, the results of 6-month injections, evaluated after one year, did not show any significant effect. In addition, 25% of patients had elevated IOP that peaked at 60 days and returned to the baseline level by 180 days (63,64). Moreover, the incidence of cataract progression increased from 4% to 26% over one year of treatment.
Corticosteroids can also be administered as a sustained-release drug with activity that persists over several months; however, the high likelihood of IOP increase and the development of cataracts make these drugs less favorable.
In a multicenter retrospective chart review of patients with CRVO and BRVO with persistent macular edema, the long-term cumulative likelihood of IOP elevation following treatment with intravitreal dexamethasone implant has been shown. However, the IOP elevation was mild and resolved with topical treatment without any need for surgical intervention (65). Another multicenter retrospective chart review of the efficacy of dexamethasone intravitreal implants for patients with persistent macular edema due to CRVO and BRVO showed that the treatment reduced central macular thickness. In addition, ocular hypertension in this study was reported in 17% of the patients (66). In a recent retrospective, consecutive series, the incidence of ocular hypertension in all patients who received intravitreal injection of 2 mg triamcinolone acetonide was reported 13.2% (67). Moreover, in another chart review of 221 patients with RVO, diabetic macular edema, uveitis, and macular edema secondary to other causes showed a higher rate of ocular hypertension after treatment with dexamethasone intravitreal implants (68). It has been concluded that the secondary ocular hypertension following dexamethasone intravitreal implant can be managed by medications; however, 4.62% of the patients with ocular hypertension needed glaucoma surgery (68). Transient ocular hypertension following intravitreal dexamethasone implant (Ozurdex) was managed only with topical medications in another study (69).
In another recent study, 78 patients received 152 intravitreal injections of 0.7 mg dexamethasone implants over 4 years. Although some patients developed ocular hypertension after the first round of injections, the incidence and severity of the intravitreal dexamethasone injections is more evident 1 to 3 months after the implant procedure (70).
Anti-VEGF therapy
VEGF is an inflammatory cytokine upregulated in eyes with RVO and responsible for vascular permeability and macular edema (14,71). The anti-VEGF monoclonal antibody, bevacizumab, was first described for resolving macular edema secondary to CRVO (72); however, this drug has been used off-label as a less expensive treatment for macular edema in individuals with all types of RVO (2). In a prospective study of patients with macular edema receiving bevacizumab for RVO, a mean improvement of 16 letters after one-year follow-up has been reported (73). Although patients with CRVO or BRVO had a reduction in central retinal thickness on OCT, a better response was noted in patients with BRVO than in those with CRVO. The same discrepancy between anatomical and functional results has also been reported in the SCORE-CRVO study, in which a better visual outcome was reported among intravitreal treatment group; nevertheless, both groups showed a reduction in central retinal thickness on OCT (60).
The results of the phase III BRAVO clinical trial evaluating the beneficial effects of the monoclonal antibody fragment ranibizumab on macular edema in RVO cases revealed a mean improvement of 7.6, 7.4 and 1.9 letters in the 0.3 mg ranibizumab, 0.5 mg ranibizumab and control groups, respectively (74). The CRUISE study demonstrated a mean improvement of 8.8, 9.3 and 1.1 letters in the 0.3 mg ranibizumab, 0.5 mg ranibizumab and control groups, respectively (74). Furthermore, both the BRVO and the CRUISE studies showed a good safety profile for ranibizumab in patients with BRVO (74). In contrast, rebound macular edema following treatment with bevacizumab has been shown (75).
In the HORIZON trial, an open label extension of the CRUISE study, the importance of early treatment for macular edema within 6 months of onset was reported with visual acuity as an endpoint (76). In the VIBRANT trial, the effectiveness of intravitreal aflibercept as a competitive receptor decoy of VEGF has been compared with the grid laser approach (77). Fifty-three percent of the patients in the aflibercept group showed an improvement in visual acuity by 3 or more lines, while 27% of the patients in the laser group showed the same improvement. The analysis revealed that the improvement in the aflibercept group was much higher than the laser group. In addition, an improvement in visual acuity by 3 or more lines in 56% of the patients in the group who received aflibercept compared to 12% of the patients in the control group was reported in the results of the GALILEO/COPERNICUS trial (78,79). However, due to the cross-trial nature of this study, the efficacy of aflibercept cannot be interpreted.
The SCORE 2 trial is the most robust study on the effect of bevacizumab compared with aflibercept on macular edema in RVO patients (80). In this trial, treatment with a monthly bevacizumab injection was compared with treatment with a monthly aflibercept injection for the first 6 months. Bevacizumab was found to be noninferior to aflibercept for the treatment of macular edema; however, this study is still ongoing, and the follow-up data may reveal more comprehensive results.
Nonetheless, intravitreal bevacizumab has been widely applied for the treatment of RVO; exceptional complications, such as acute angle closure glaucoma in patients with narrow angle or hyperopic eyes and RNFL thickness, should be taken into consideration. High IOP in some patients can be managed with medication, though laser iridectomy might be needed in severe and refractory cases (81). Furthermore, the association between the progression of glaucoma and changes in the RNFL thickness from multiple intravitreal anti-VEGF injections has been reported. However, the decreased central macular thickness in the RNFL might occur after the anatomical improvement of macular lesions (82). The results of the VEGA randomized clinical trial also demonstrated the beneficial effects of intravitreal aflibercept for the improvement of IOP control among patients with neovascular glaucoma (83). A meta-analysis of 74 studies on the short-term and long-term effects of intravitreal injection of anti-VEGF reported a significant short-term increase in IOP and no significant long-term IOP changes. Furthermore, decreased RNFL thickness was also reported at 6 and 12 months after intravitreal anti-VEGF injection. Nevertheless, the IOP of one month after the intravitreal injection of a dexamethasone implant was significantly higher than the baseline, indicating that patients with advanced glaucoma would benefit from IOP-lowering medications (84).
In addition, in recent studies, the application of peripheral targeted laser photocoagulation to reduce the burden of anti-VEGF treatment in the case of upregulated VEGF levels in the peripheral ischemic retina has been proposed (85-87). However, no beneficial effects of using peripheral laser therapy for macular edema has been reported.
Based on the European Society of Retina Specialists (EURETINA) guidelines for the management of RVO, initial history, physical exam and basic systemic workup for systemic conditions are highly recommended (88). They also suggested that imaging modalities such as OCT-A allow us to assess the amount of retinal ischemia and late onset retinal complications which is in line with the Preferred Practice Pattern® (PPP) Guidelines released in 2016 (89). In addition, per EURETINA guideline, anti-VEGF treatment with the immediate inhibition of vascular leakage, is the treatment of choice; however, long-term monitoring and individualized assessment for treatment plan such as steroid therapy in case of cardiovascular risk, laser treatment if follow-up is not feasible and surgery in few cases is recommended (88,89). Moreover, Royal College of Ophthalmologists Retinal Vein Occlusion Guideline recommends that anti-VEGF treatment improves macular edema resulted from CRVO, although non-ischemic CRVO might resolve without complication and macular edema is the most common complication of CRVO (90). Regarding BRVO, early treatment is recommended since the chance of spontaneous improvement is low and the delayed presentation may occur in some patients. Furthermore, medical investigations including medical history, blood pressure and blood sugar measurement and laboratory tests for complete blood count and ESR are recommended as to evaluate for atherosclerosis, diabetes mellitus, high blood pressure and abnormal lipid profile (90). Based on this guideline, OCT can be utilized to diagnose, follow-up and evaluate the treatment response of RVO induced macular edema. Moreover, FA/OCTA is recommended for evaluating the retinal nonperfusion areas to detect the eyes with ischemic CRVO (90).
Summary
RVO is a chronic condition that threatens vision and has a spectrum of clinical manifestations. There is no definitive treatment to improve perfusion in RVO, and current approaches mainly focus on diminishing RVO complications, such as macular edema and neovascularization. Based on the possible shared pathogenesis between RVO and glaucoma and common risk factors between these two, a few considerations may be warranted in treating RVO patients. Most importantly, close follow-up for glaucoma development of fellow eye especially in patients with non-AV-crossing RVO is important. Use of anti-VEGF antibodies, or corticosteroids, as these medications may themselves cause elevated IOP, may complicate glaucoma management. In case of Ozurdex, IOP risk peaks in 2 months and may be managed by addition of IOP-lowering medications if monitored closely. However, with preexisting glaucoma, 3% of patients may need shunting procedures to reduce post-injection IOP elevation. There is a need to better evaluate the benefit of deferred grid laser therapy following anti-VEGF treatment in RVO patients. This modality may reduce the need for injections and IOP spikes in patients with preexisting POAG. In addition, early treatment of macular edema in RVO is recommended, as it would both result in better visual outcome and fewer injections, which may reduce the unwanted side effects in POAG patients. With the increasing use of OCT-A in everyday practice, there is also a need to describe the potential changes in optic nerve for glaucoma-suspect individuals as well as optic nerve perfusion in glaucoma patients to assess the risk and prevalence of ischemic RVO based on the optic nerve vascular distribution and the location of RVO.
Acknowledgments
Funding: None.
Footnote
Reporting Checklist: The authors have completed the Narrative Review reporting checklist. Available at https://atm.amegroups.com/article/view/10.21037/atm-22-2730/rc
Peer Review File: Available at https://atm.amegroups.com/article/view/10.21037/atm-22-2730/prf
Conflicts of Interest: All authors have completed the ICMJE uniform disclosure form (available at https://atm.amegroups.com/article/view/10.21037/atm-22-2730/coif). The authors have no conflicts of interest to declare.
Ethical Statement: The authors are accountable for all aspects of the work in ensuring that questions related to the accuracy or integrity of any part of the work are appropriately investigated and resolved.
Open Access Statement: This is an Open Access article distributed in accordance with the Creative Commons Attribution-NonCommercial-NoDerivs 4.0 International License (CC BY-NC-ND 4.0), which permits the non-commercial replication and distribution of the article with the strict proviso that no changes or edits are made and the original work is properly cited (including links to both the formal publication through the relevant DOI and the license). See: https://creativecommons.org/licenses/by-nc-nd/4.0/.
References
- Cugati S, Wang JJ, Rochtchina E, et al. Ten-year incidence of retinal vein occlusion in an older population: the Blue Mountains Eye Study. Arch Ophthalmol 2006;124:726-32. [Crossref] [PubMed]
- Karia N. Retinal vein occlusion: pathophysiology and treatment options. Clin Ophthalmol 2010;4:809-16. [Crossref] [PubMed]
- Hayreh SS. Prevalent misconceptions about acute retinal vascular occlusive disorders. Prog Retin Eye Res 2005;24:493-519. [Crossref] [PubMed]
- Risk factors for branch retinal vein occlusion. The Eye Disease Case-control Study Group. Am J Ophthalmol 1993;116:286-96. [Crossref] [PubMed]
- Risk factors for central retinal vein occlusion. The Eye Disease Case-Control Study Group. Arch Ophthalmol 1996;114:545-54. [Crossref] [PubMed]
- Klein BE, Meuer SM, Knudtson MD, et al. The relationship of optic disk cupping to retinal vein occlusion: the Beaver Dam Eye Study. Am J Ophthalmol 2006;141:859-62. [Crossref] [PubMed]
- Quigley HA, Broman AT. The number of people with glaucoma worldwide in 2010 and 2020. Br J Ophthalmol 2006;90:262-7. [Crossref] [PubMed]
- Weinreb RN, Aung T, Medeiros FA. The pathophysiology and treatment of glaucoma: a review. JAMA 2014;311:1901-11. [Crossref] [PubMed]
- Tham YC, Li X, Wong TY, et al. Global prevalence of glaucoma and projections of glaucoma burden through 2040: a systematic review and meta-analysis. Ophthalmology 2014;121:2081-90. [Crossref] [PubMed]
- Weinreb RN, Khaw PT. Primary open-angle glaucoma. Lancet 2004;363:1711-20. [Crossref] [PubMed]
- Green WR, Chan CC, Hutchins GM, et al. Central retinal vein occlusion: a prospective histopathologic study of 29 eyes in 28 cases. Retina 1981;1:27-55. [PubMed]
- Campochiaro PA, Hafiz G, Shah SM, et al. Ranibizumab for macular edema due to retinal vein occlusions: implication of VEGF as a critical stimulator. Mol Ther 2008;16:791-9. [Crossref] [PubMed]
- Yoshimura T, Sonoda KH, Sugahara M, et al. Comprehensive analysis of inflammatory immune mediators in vitreoretinal diseases. PLoS One 2009;4:e8158. [Crossref] [PubMed]
- Funk M, Kriechbaum K, Prager F, et al. Intraocular concentrations of growth factors and cytokines in retinal vein occlusion and the effect of therapy with bevacizumab. Invest Ophthalmol Vis Sci 2009;50:1025-32. [Crossref] [PubMed]
- Han JC, Eo DR, Lee TK, et al. Does Glaucoma Share Common Pathogenesis with Branch Retinal Vein Occlusion? PLoS One 2016;11:e0156966. [Crossref] [PubMed]
- Pasquale LR, Kang JH, Manson JE, et al. Prospective study of type 2 diabetes mellitus and risk of primary open-angle glaucoma in women. Ophthalmology 2006;113:1081-6. [Crossref] [PubMed]
- Hayreh SS, Zimmerman MB, Beri M, et al. Intraocular pressure abnormalities associated with central and hemicentral retinal vein occlusion. Ophthalmology 2004;111:133-41. [Crossref] [PubMed]
- Yin X, Li J, Zhang B, et al. Association of glaucoma with risk of retinal vein occlusion: A meta-analysis. Acta Ophthalmol 2019;97:652-9. [Crossref] [PubMed]
- Actis AG, Belli L, Dall'orto L, et al. Morphology of optic disc through heidelberg retina tomograph in retinal vein occlusions alone or in combination with primary open angle glaucoma. Open Ophthalmol J 2013;7:34-41. [Crossref] [PubMed]
- Shin YI, Nam KY, Lee SE, et al. Changes in Peripapillary Microvasculature and Retinal Thickness in the Fellow Eyes of Patients With Unilateral Retinal Vein Occlusion: An OCTA Study. Invest Ophthalmol Vis Sci 2019;60:823-9. [Crossref] [PubMed]
- Lévêque PM, Zéboulon P, Brasnu E, et al. Optic Disc Vascularization in Glaucoma: Value of Spectral-Domain Optical Coherence Tomography Angiography. J Ophthalmol 2016;2016:6956717. [Crossref] [PubMed]
- Bojikian KD, Chen CL, Wen JC, et al. Optic Disc Perfusion in Primary Open Angle and Normal Tension Glaucoma Eyes Using Optical Coherence Tomography-Based Microangiography. PLoS One 2016;11:e0154691. [Crossref] [PubMed]
- Kim MJ, Woo SJ, Park KH, et al. Retinal nerve fiber layer thickness is decreased in the fellow eyes of patients with unilateral retinal vein occlusion. Ophthalmology 2011;118:706-10. [Crossref] [PubMed]
- David R, Zangwill L, Badarna M, et al. Epidemiology of retinal vein occlusion and its association with glaucoma and increased intraocular pressure. Ophthalmologica 1988;197:69-74. [Crossref] [PubMed]
- Xu K, Wu L, Ma Z, et al. Primary angle closure and primary angle closure glaucoma in retinal vein occlusion. Acta Ophthalmol 2019;97:e364-72. [Crossref] [PubMed]
- Kolar P. Risk factors for central and branch retinal vein occlusion: a meta-analysis of published clinical data. J Ophthalmol 2014;2014:724780. [Crossref] [PubMed]
- Sherpa D, Shakya S, Shrestha JK. Association of primary glaucomas with retinal vein occlusion. Kathmandu Univ Med J (KUMJ) 2008;6:49-54. [PubMed]
- Na KI, Jeoung JW, Kim YK, et al. Incidence of Open-angle Glaucoma in Newly Diagnosed Retinal Vein Occlusion: A Nationwide Population-based Study. J Glaucoma 2019;28:111-8. [Crossref] [PubMed]
- Barnett EM, Fantin A, Wilson BS, et al. The incidence of retinal vein occlusion in the ocular hypertension treatment study. Ophthalmology 2010;117:484-8. [Crossref] [PubMed]
- Park HL, Jung Y, Han K, et al. Health care claims for primary open-angle glaucoma and retinal vein occlusion from an 11-year nationwide dataset. Sci Rep 2017;7:8038. [Crossref] [PubMed]
- Rong AJ, Swaminathan SS, Vanner EA, et al. Predictors of Neovascular Glaucoma in Central Retinal Vein Occlusion. Am J Ophthalmol 2019;204:62-9. [Crossref] [PubMed]
- Caillaux V, Valtot F, Souied EH, et al. Intraocular pressure after intravitreal injection of dexamethasone implant for macular edema resulting from retinal vein occlusion. Eur J Ophthalmol 2015;25:454-8. [Crossref] [PubMed]
- Aref AA, Scott IU, Oden NL, et al. Incidence, Risk Factors, and Timing of Elevated Intraocular Pressure After Intravitreal Triamcinolone Acetonide Injection for Macular Edema Secondary to Retinal Vein Occlusion: SCORE Study Report 15. JAMA Ophthalmol 2015;133:1022-9. [Crossref] [PubMed]
- Lucatto LFA, Magalhães-Junior O, Prazeres JMB, et al. Incidence of anterior segment neovascularization during intravitreal treatment for macular edema secondary to central retinal vein occlusion. Arq Bras Oftalmol 2017;80:97-103. [Crossref] [PubMed]
- Chen HF, Chen MC, Lai CC, et al. Neovascular glaucoma after central retinal vein occlusion in pre-existing glaucoma. BMC Ophthalmol 2014;14:119. [Crossref] [PubMed]
- Kim S, Sung KR, Joe SG, et al. Comparison between glaucomatous and non-glaucomatous eyes with unilateral retinal vein occlusion in the fellow eye. Korean J Ophthalmol 2013;27:440-5. [Crossref] [PubMed]
- Lopilly Park HY, Jeon S, Lee MY, et al. Glaucoma Progression in the Unaffected Fellow Eye of Glaucoma Patients Who Developed Unilateral Branch Retinal Vein Occlusion. Am J Ophthalmol 2017;175:194-200. [Crossref] [PubMed]
- Wittström E, Ponjavic V, Lövestam-Adrian M, et al. Electrophysiological evaluation and visual outcome in patients with central retinal vein occlusion, primary open-angle glaucoma and neovascular glaucoma. Acta Ophthalmol 2010;88:86-90. [Crossref] [PubMed]
- Ip M, Hendrick A. Retinal Vein Occlusion Review. Asia Pac J Ophthalmol (Phila) 2018;7:40-5. [PubMed]
- McAllister IL, Tan MH, Smithies LA, et al. The effect of central retinal venous pressure in patients with central retinal vein occlusion and a high mean area of nonperfusion. Ophthalmology 2014;121:2228-36. [Crossref] [PubMed]
- A randomized clinical trial of early panretinal photocoagulation for ischemic central vein occlusion. The Central Vein Occlusion Study Group N report. Ophthalmology 1995;102:1434-44. [Crossref] [PubMed]
- Argon laser scatter photocoagulation for prevention of neovascularization and vitreous hemorrhage in branch vein occlusion. A randomized clinical trial. Branch Vein Occlusion Study Group. Arch Ophthalmol 1986;104:34-41. [Crossref] [PubMed]
- Fekrat S, de Juan E Jr. Chorioretinal venous anastomosis for central retinal vein occlusion: transvitreal venipuncture. Ophthalmic Surg Lasers 1999;30:52-5. [Crossref] [PubMed]
- McAllister IL, Gillies ME, Smithies LA, et al. The Central Retinal Vein Bypass Study: a trial of laser-induced chorioretinal venous anastomosis for central retinal vein occlusion. Ophthalmology 2010;117:954-65. [Crossref] [PubMed]
- Feltgen N, Junker B, Agostini H, et al. Retinal endovascular lysis in ischemic central retinal vein occlusion: one-year results of a pilot study. Ophthalmology 2007;114:716-23. [Crossref] [PubMed]
- Arevalo JF, Garcia RA, Wu L, et al. Radial optic neurotomy for central retinal vein occlusion: results of the Pan-American Collaborative Retina Study Group (PACORES). Retina 2008;28:1044-52. [Crossref] [PubMed]
- Călugăru D, Călugăru M. Management of the open angle glaucoma in patients with central/hemicentral retinal vein occlusions. Int J Ophthalmol 2019;12:436-41. [PubMed]
- Michaelides M, Foster PJ. Retinal vein occlusion and angle closure: a retrospective case series. J Glaucoma 2010;19:643-9. [Crossref] [PubMed]
- Theodoropoulou S, Ellabban AA, Johnston RL, et al. Short-term safety of dexamethasone implant for treatment of macular edema due to retinal vein occlusion, in eyes with glaucoma or treated ocular hypertension. Graefes Arch Clin Exp Ophthalmol 2017;255:725-32. [Crossref] [PubMed]
- Rogers SL, McIntosh RL, Lim L, et al. Natural history of branch retinal vein occlusion: an evidence-based systematic review. Ophthalmology 2010;117:1094-1101.e5. [Crossref] [PubMed]
- Mohamed Q, McIntosh RL, Saw SM, et al. Interventions for central retinal vein occlusion: an evidence-based systematic review. Ophthalmology 2007;114:507-19, 524. [Crossref] [PubMed]
- Squizzato A, Manfredi E, Bozzato S, et al. Antithrombotic and fibrinolytic drugs for retinal vein occlusion: a systematic review and a call for action. Thromb Haemost 2010;103:271-6. [Crossref] [PubMed]
- Lazo-Langner A, Hawel J, Ageno W, et al. Low molecular weight heparin for the treatment of retinal vein occlusion: a systematic review and meta-analysis of randomized trials. Haematologica 2010;95:1587-93. [Crossref] [PubMed]
- Arnarsson A, Stefánsson E. Laser treatment and the mechanism of edema reduction in branch retinal vein occlusion. Invest Ophthalmol Vis Sci 2000;41:877-9. [PubMed]
- McIntosh RL, Mohamed Q, Saw SM, et al. Interventions for branch retinal vein occlusion: an evidence-based systematic review. Ophthalmology 2007;114:835-54. [Crossref] [PubMed]
- Park CH, Jaffe GJ, Fekrat S. Intravitreal triamcinolone acetonide in eyes with cystoid macular edema associated with central retinal vein occlusion. Am J Ophthalmol 2003;136:419-25. [Crossref] [PubMed]
- Ip MS, Gottlieb JL, Kahana A, et al. Intravitreal triamcinolone for the treatment of macular edema associated with central retinal vein occlusion. Arch Ophthalmol 2004;122:1131-6. [Crossref] [PubMed]
- Patel PJ, Zaheer I, Karia N. Intravitreal triamcinolone acetonide for macular oedema owing to retinal vein occlusion. Eye (Lond) 2008;22:60-4. [Crossref] [PubMed]
- Miyamoto N, Iossifov D, Metge F, et al. Early effects of intravitreal triamcinolone on macular edema: mechanistic implication. Ophthalmology 2006;113:2048-53. [Crossref] [PubMed]
- Ip MS, Scott IU, VanVeldhuisen PC, et al. A randomized trial comparing the efficacy and safety of intravitreal triamcinolone with observation to treat vision loss associated with macular edema secondary to central retinal vein occlusion: the Standard Care vs Corticosteroid for Retinal Vein Occlusion (SCORE) study report 5. Arch Ophthalmol 2009;127:1101-14. [Crossref] [PubMed]
- Scott IU, Ip MS, VanVeldhuisen PC, et al. A randomized trial comparing the efficacy and safety of intravitreal triamcinolone with standard care to treat vision loss associated with macular Edema secondary to branch retinal vein occlusion: the Standard Care vs Corticosteroid for Retinal Vein Occlusion (SCORE) study report 6. Arch Ophthalmol 2009;127:1115-28. [Crossref] [PubMed]
- Dexamethasone Intravitreal Implant Approved for Retinal Vein Occlusion. Medscape Medical News. Available online: http://www.medscape.com/viewarticle/704854. Accessed March 1, 2010.
- 12-month evaluation of an applicator-delivered dexamethasone intravitreal implant in patients with macular edema due to branch or central retinal vein occlusion. the 33rd Annual Macula Society Meeting; Feb 26; Tuscan, AZ2010. Available online: http://www.maculasociety.org/files/Macula2010-ScientificProgram.pdf
- Haller JA, Bandello F, Belfort R Jr, et al. Randomized, sham-controlled trial of dexamethasone intravitreal implant in patients with macular edema due to retinal vein occlusion. Ophthalmology 2010;117:1134-1146.e3. [Crossref] [PubMed]
- Zarranz-Ventura J, Sala-Puigdollers A, Velazquez-Villoria D, et al. Long-term probability of intraocular pressure elevation with the intravitreal dexamethasone implant in the real-world. PLoS One 2019;14:e0209997. [Crossref] [PubMed]
- Houben I, De Zaeytijd J, Deghislage C, et al. Efficacy of Multiple Dexamethasone Intravitreal Implants for Refractory Retinal Vein Occlusion-Related Macular Edema and Effect of Prior Vitrectomy. J Ocul Pharmacol Ther 2018;34:710-9. [Crossref] [PubMed]
- Storey PP, Obeid A, Pancholy M, et al. Ocular hypertension after intravitreal injection of 2-MG triamcinolone. Retina 2020;40:75-9. [Crossref] [PubMed]
- Hemarat K, Kemmer JD, Porco TC, et al. Secondary Ocular Hypertension and the Risk of Glaucoma Surgery After Dexamethasone Intravitreal Implant in Routine Clinical Practice. Ophthalmic Surg Lasers Imaging Retina 2018;49:680-5. [Crossref] [PubMed]
- Malclès A, Dot C, Voirin N, et al. Safety of intravitreal dexamethasone implant (OZURDEX): The SAFODEX study. Incidence and Risk Factors of Ocular Hypertension. Retina 2017;37:1352-9. [Crossref] [PubMed]
- Pacella E, Loffredo L, Malvasi M, et al. Effects of Repeated Intravitreal Injections of Dexamethasone Implants on Intraocular Pressure: A 4-Year Study. Clin Ophthalmol 2020;14:3611-7. [Crossref] [PubMed]
- Aiello LP, Avery RL, Arrigg PG, et al. Vascular endothelial growth factor in ocular fluid of patients with diabetic retinopathy and other retinal disorders. N Engl J Med 1994;331:1480-7. [Crossref] [PubMed]
- Rosenfeld PJ, Fung AE, Puliafito CA. Optical coherence tomography findings after an intravitreal injection of bevacizumab (avastin) for macular edema from central retinal vein occlusion. Ophthalmic Surg Lasers Imaging 2005;36:336-9. [Crossref] [PubMed]
- Prager F, Michels S, Kriechbaum K, et al. Intravitreal bevacizumab (Avastin) for macular oedema secondary to retinal vein occlusion: 12-month results of a prospective clinical trial. Br J Ophthalmol 2009;93:452-6. [Crossref] [PubMed]
- Varma R, Bressler NM, Suñer I, et al. Improved vision-related function after ranibizumab for macular edema after retinal vein occlusion: results from the BRAVO and CRUISE trials. Ophthalmology 2012;119:2108-18. [Crossref] [PubMed]
- Matsumoto Y, Freund KB, Peiretti E, et al. Rebound macular edema following bevacizumab (Avastin) therapy for retinal venous occlusive disease. Retina 2007;27:426-31. [Crossref] [PubMed]
- Heier JS, Campochiaro PA, Yau L, et al. Ranibizumab for macular edema due to retinal vein occlusions: long-term follow-up in the HORIZON trial. Ophthalmology 2012;119:802-9. [Crossref] [PubMed]
- Campochiaro PA, Clark WL, Boyer DS, et al. Intravitreal aflibercept for macular edema following branch retinal vein occlusion: the 24-week results of the VIBRANT study. Ophthalmology 2015;122:538-44. [Crossref] [PubMed]
- Heier JS, Clark WL, Boyer DS, et al. Intravitreal aflibercept injection for macular edema due to central retinal vein occlusion: two-year results from the COPERNICUS study. Ophthalmology 2014;121:1414-1420.e1. [Crossref] [PubMed]
- Ogura Y, Roider J, Korobelnik JF, et al. Intravitreal aflibercept for macular edema secondary to central retinal vein occlusion: 18-month results of the phase 3 GALILEO study. Am J Ophthalmol 2014;158:1032-8. [Crossref] [PubMed]
- Scott IU, VanVeldhuisen PC, Ip MS, et al. Effect of Bevacizumab vs Aflibercept on Visual Acuity Among Patients With Macular Edema Due to Central Retinal Vein Occlusion: The SCORE2 Randomized Clinical Trial. JAMA 2017;317:2072-87. [Crossref] [PubMed]
- Jeong S, Sagong M, Chang W. Acute angle closure attack after an intravitreal bevacizumab injection for branch retinal vein occlusion: a case report. BMC Ophthalmol 2017;17:25. [Crossref] [PubMed]
- Ahn J, Jang K, Sohn J, et al. Effect of intravitreal ranibizumab and aflibercept injections on retinal nerve fiber layer thickness. Sci Rep 2021;11:5010. [Crossref] [PubMed]
- Inatani M, Higashide T, Matsushita K, et al. Intravitreal Aflibercept in Japanese Patients with Neovascular Glaucoma: The VEGA Randomized Clinical Trial. Adv Ther 2021;38:1116-29. [Crossref] [PubMed]
- de Vries VA, Bassil FL, Ramdas WD. The effects of intravitreal injections on intraocular pressure and retinal nerve fiber layer: a systematic review and meta-analysis. Sci Rep 2020;10:13248. [Crossref] [PubMed]
- Spaide RF. Peripheral areas of nonperfusion in treated central retinal vein occlusion as imaged by wide-field fluorescein angiography. Retina 2011;31:829-37. [Crossref] [PubMed]
- Campochiaro PA, Hafiz G, Mir TA, et al. Scatter Photocoagulation Does Not Reduce Macular Edema or Treatment Burden in Patients with Retinal Vein Occlusion: The RELATE Trial. Ophthalmology 2015;122:1426-37. [Crossref] [PubMed]
- Wykoff CC, Ou WC, Wang R, et al. Peripheral Laser for Recalcitrant Macular Edema Owing to Retinal Vein Occlusion: The WAVE Trial. Ophthalmology 2017;124:919-21. [Crossref] [PubMed]
- Schmidt-Erfurth U, Garcia-Arumi J, Gerendas BS, et al. Guidelines for the Management of Retinal Vein Occlusion by the European Society of Retina Specialists (EURETINA). Ophthalmologica 2019;242:123-62. [Crossref] [PubMed]
- Pulido JS, Flaxel CJ, Adelman RA, et al. Retinal Vein Occlusions Preferred Practice Pattern((R)) Guidelines. Ophthalmology 2016;123:182-208. [Crossref] [PubMed]
- Nicholson L, Talks SJ, Amoaku W, et al. Retinal vein occlusion (RVO) guideline: executive summary. Eye (Lond) 2022;36:909-12. [Crossref] [PubMed]