Comparison of robot-assisted vitreoretinal surgery and manual surgery in different preclinical settings: a randomized trial
Introduction
Progress in the field of surgical robotics has allowed surgeons to reduce the limitations of human hands and has substantially improved the dexterity and accuracy of surgery (1-4). The most widespread and commercially available robotic surgical system is the da Vinci Surgical System (Intuitive Surgical, Sunnyvale, CA, USA), the introduction of which in the operating room has revolutionized several specialties. Robot-assisted surgery is mainly applied in the field of laparoscopic and thoracoscopic procedures; however, to date, it has been rare in ophthalmic surgery (5-7).
Vitreoretinal surgery is one of the most technically challenging microsurgeries (8,9). Fine, precise motions are essential for surgery on extremely delicate tissue within the small, constrained interspace of the eyeball, often requiring forces that are below the level of human tactile perception (10). Vitreoretinal surgery requires some of the most delicate instruments in the field of microsurgery. However, to date, the theoretical advantages of surgical robots in terms of tremor filtering and accuracy have not been evaluated in intraocular surgery.
In recent years, research on robots dedicated to intraocular surgery has increased (11-13). Technically challenging processes such as microcannulation in the retinal vessel may benefit from robotic surgery (14,15). Due to the small size of retinal vessels, these operations are either at or beyond the limitations of many vitreoretinal surgeons due to physiological tremors. The application of surgical robots in vitreoretinal surgery requires multidimensional and objective assessment.
A surgical simulator incorporates virtual reality (VR) technology into a bionic eyeball to create a dummy surgical scenario (16). The simulator can externalize comprehensive clinical characters with sophisticated programming, interactively react to the operator, and independently supply surgical evaluation and feedback (17,18). With its automatic and objective evaluation process, the simulator establishes a secure and standardized test setting, which provides quantifiable data regarding surgical performance. Therefore, surgical simulator tests are suitable options for the preclinical evaluation of surgical robots.
This study aimed to investigate robot-assisted vitreoretinal surgery compared with manual surgery performed by both residents and experienced vitreoretinal experts in a VR surgical simulator using donor porcine eyes. We present the following article in accordance with the CONSORT reporting checklist (available at https://atm.amegroups.com/article/view/10.21037/atm-22-1315/rc).
Methods
A single-center, randomized controlled trial (balanced randomization at a 1:1 ratio) was conducted. Data were collected at the Zhongshan Ophthalmic Center (ZOC), Sun Yat-sen University, between April 2021 and January 2022. The ethics committee of ZOC adjudicated that ethics review approval was not needed for this study. The full trial protocol was provided under the Footnote section. The principles outlined in the Declaration of Helsinki (as revised in 2013) were followed throughout the study.
Participants
Participants consisted of experienced vitreoretinal experts (10–15 years of experience) and residents without intraocular surgical experience (first- or second-year residents) from ZOC. After prior examination to reach an agreement of operating level, 10 participants in each group were included in the study. Experts and residents who had trained for more than 2 hours on the Eyesi Simulator (VRmagic, Mannheim, Germany) during the past 6 months were not eligible to partake in the research. All participants gave written content before being included in the research.
Robotic system
The robot (Oculotronics Medical Instrument, Guangzhou, China) consisted of 3 parts: a three-dimensional (3D) force sensor attached to the tool holder, a hybrid parallel-serial micromanipulator, which could change the remote center of motion (RCM) point during the motion process, and an RCM adjustment strategy based on online learning. The force sensor could obtain the force produced by the instrument in real-time, and the force value was used to decide the RCM strategy for the surgical system. Control of the RCM was implemented by the hybrid parallel-serial micromanipulator, which included 3 translations and 2 angles on its end-effector. Additionally, the surgeon could control the manipulator with the robot controller, which had 3 dimensions to control the rotation angle θ1, the rotation angle θ2, and the deep distance Z of the end effector. Accuracy was 10 µm in all 3 directions. In addition, the RCM adjustment strategy provided a compensation approach to adjust the RCM point and reduce the force of the system. This strategy achieved satisfying performance in trajectory deformation and force reduction. Moreover, dynamic motion scaling, tremor filtering, and freeze positioning were integrated into the surgical system, and these extra features were implemented using a software program, which provided an operator interface.
Intervention
The 10 residents and 10 vitreoretinal experts were randomly divided into the robot-assisted and manual surgery groups. A computer-generated list of random numbers was used to randomly assign participants to the 2 conditions (manual or robot-assisted surgery) by following a balanced permutation (computer-generated random numbers). Thus, there were 4 groups in total, and each group comprised 5 participants. Group 1 included residents operating manually; Group 2 comprised residents operating with robot assistance; Group 3 included experts operating manually; and Group 4 comprised experts operating with robot assistance. Participants in Groups 2 and 4 needed to familiarize themselves with the robotic surgery until they could control the robotic instrument manipulator according to instructions provided by the researchers. All participants completed a warm-up session involving 10 min of introduction and 1 familiarization session consisting of either robot-assisted or manual surgery before the data collection process was initiated. The warm-up term was used to familiarize participants with the equipment and procedure. One of the researchers introduced the performance and procedural targets of each test. The data collection procedure comprised 3 repetitions of the test (Figure 1).
Outcomes of the simulator tests
The vitreoretinal interface of the Eyesi Simulator can provide automated assessments to test surgical performance. Two tests performed on the Eyesi Simulator were included in this study. Test 1 involved the navigation module, and Test 2 involved antitremor training. Detailed descriptions and characteristics of the 2 tests are shown in Table 1. We selected 4 outcomes for this study: the efficiency of operation, which was (I) the time taken following instrument insertion until test completion (min) and (II) the distance traveled by the instrument tip as measured by an odometer (mm); (III) the accuracy of operation, determined by whether the instrument slipped out of the target sphere (red ball in the vitreous); and (IV) the performance of tremor control, determined by the instrument tip exceeding the tolerance percentage.
Table 1
Test | Setting | Name | Description | Difficulty level* | Main outcomes |
---|---|---|---|---|---|
1 | Eyesi simulator | Navigation training | Move the instrument tip into the center of the green sphere and hold until the sphere turns green | Level 4 out of 4 | Efficiency, accuracy |
2 | Eyesi simulator | Anti-tremor training | Keep the handle tip inside the sphere and guide the sphere to follow a circular trajectory until the destination is reached | Level 5 out of 7 | Efficiency, tremor control |
3 | Harvested porcine eyes | Feasibility test | Perform retinal endovascular surgeries with microneedles | Hard for residents | Feasibility |
*, level 1 is the easiest, and the difficulty increases at higher levels.
Feasibility test in donor porcine eyes
This test was performed in harvested porcine eyes stuck on the orbital fossa of a facial model (Figure 2). A fabricated microneedle with an outer diameter of 50 µm and an inner diameter of 20 µm was used to pierce the retinal vein. When the microneedle was connected to a 1-mL syringe containing a sterile saline, the solution could be injected into a vessel. Each participant performed surgery 3 times, and the performances of the surgeries were recorded. Successful injection was defined as injecting 1-mL sterile saline at one time. In addition, the participant and 2 ophthalmologist recorders judged the performance of the surgeries, and the majority opinion was taken as the final result. The porcine eyes were slaughterhouse materials. Experiments were performed under a project license (No. 2019-146) granted by the Institutional Animal Care and Use Committee of ZOC, in compliance with the Association for Research in Vision and Ophthalmology guidelines for the care and use of animals.
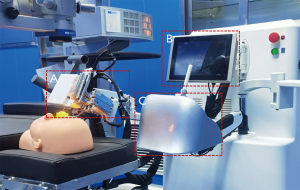
Statistical analysis
The researchers who undertook the outcomes assessment were blinded to group assignment. Data compilation processes and analyses were conducted using SPSS 26.0 statistical software (IBM Corp., Armonk, NY, USA). One-way analysis of variance (ANOVA) and Bonferroni’s tests were used to analyze the differences in the 3 outcomes of the simulator tests among the 4 groups. A chi-squared test was used to compare the feasibility tests. Values were shown as mean ± SD. All reported P values are 2-tailed, and statistical significance was defined at the α=0.05 level.
Results
Time efficiency of operations
After random assignment, there were 4 groups in total, with 5 participants in each group. The total time for completing Test 1 and Test 2 operations was recorded. In Test 1, Group 2 spent more time than Group 1 (P<0.001), and Group 4 spent more time than Group 3 (P<0.001, Figure 3A). In Test 2, Group 2 was slower than Group 1 (P<0.001), and Group 4 was slower than Group 3 (P<0.001, Figure 3B). The time efficiency of robot-assisted operation was less than that of manual operation. Figure 3C shows the intraocular odometer of the instrument for the 4 groups. There were no significant differences between the robot-assisted and manual groups in either Test 1 or Test 2 (Table 2).
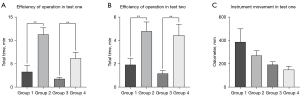
Table 2
Group | Test one (SD) | Test two (SD) | ||||
---|---|---|---|---|---|---|
Total time, min | Odometer, mm | Instrument slipped out of sphere, times | Total time, min | Out of tolerance percentage, % | ||
1 | 3.29 (1.36) | 388.10 (113.38) | 11.40 (4.16) | 1.95 (0.54) | 77.90 (4.63) | |
2 | 11.31 (1.42) | 271.40 (41.42) | 4.80 (2.05) | 4.83 (0.79) | 45.40 (5.02) | |
3 | 1.78 (0.31) | 193.30 (25.27) | 9.20 (3.77) | 1.19 (0.25) | 40.90 (7.09) | |
4 | 6.20 (1.25) | 150.30 (29.68) | 2.70 (1.89) | 4.49 (0.94) | 21.10 (10.01) |
Group 1: residents operating manually; Group 2: residents operating with robot assistance; Group 3: experts operating manually; Group 4: experts operating with robot assistance.
Surgical accuracy
One essential index for evaluating instrument handling in Test 1 was the number of times that the instrument slipped out of the target sphere. The fewer times the instrument slipped out of the target sphere, the more accurate the surgery. The number of times that the instrument slipped out of the target sphere in Group 2 was less than that in Group 1 (P=0.025), and likewise, the number of slips in Group 4 was less than that in Group 3 (P=0.028). This shows that robot-assisted operation is more precise than manual operation (Figure 4A, Table 2).
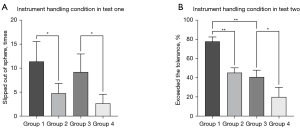
Performance of tremor control
The percentage of instrument tips exceeding the tolerance was a vital index for evaluating tremor control of operators in Test 2. The lower the excess degree of tolerance percentage, the better the tremor control performance. Figure 4B shows that the degree to which the instrument tip exceeded the tolerance percentage in Group 2 was less than that in Group 1 (P<0.001), and likewise, the degree in Group 4 was less than that in Group 3 (P=0.002). In addition, the difference between Group 1 and Group 3 was significant (P<0.001), yet Group 2 and Group 3 were not significantly different (P=1.0, Table 2). This finding demonstrates that the residents seemed to improve their ability to control tremors with the assistance of the robot and achieved a performance comparable to that of experts.
Feasibility performance in donor porcine eyes
Retinal endovascular surgeries with microneedles in harvested porcine eyes were used to evaluate the feasibility of robot operations in real eyeballs. The targeted retinal vessels had inner diameters of approximately 100 µm. The tip was made with an outer diameter of 50 µm. The feasibility performances of microcannulation in Group 2 were higher than those in Group 1 (P=0.038), yet Groups 3 and 4 were not significantly different (P=0.291). The manual performances of microcannulation of residents (Group 1) and vitreoretinal experts (Group 3) were significantly different (P=0.01). With the assistance of the robot, residents seemed to perform this surgery as well as vitreoretinal experts (P=0.49). Moreover, during robot-assisted operations, the microneedle was more stable during injections (Figure 5).
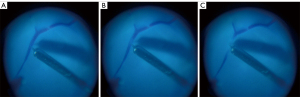
Discussion
Our research investigated the feasibility and potential advantages of robot-assisted surgery from multiple dimensions: a surgical simulator was utilized to test (I) performance accuracy (II) and antitremor performance, and harvested porcine eyes were used to test (III) the feasibility of microcannulation. Compared with manual surgery, robot-assisted vitreoretinal surgeries were more precise and stable, although robot-assisted surgery was slower than manual surgery. In particular, the performance of residents significantly improved with robot-assisted vitreoretinal surgery.
During intraocular surgery, experts operate on tiny, delicate tissues, which require delicate manipulation and steady hand movements (19). Therefore, it is considered one of the most challenging microsurgery procedures. For the past 30 years, robot-assisted surgical devices have focused on overcoming these challenges. Several systems have been developed to improve microsurgical accuracy by eliminating hand tremors and facilitating faster, safer, and more effective microsurgeries (20,21).
The robot in our research moved with 5 degrees of freedom and possessed a displacement accuracy of 10 µm. A novel master-slave remote control system was embedded in the robot. The slave-side robotic arm could handle different surgical instruments to perform various operations, and the operator used the master side for control. It has been reported that through the master-slave robotic system, tremor filtering, amplified force feedback, reduced motion mapping, and stable instrument handling could be improved to enhance the safety of surgical operations (22,23). In addition, intraocular surgery requires complex manipulation in a small, confined space through a scleral trocar. In robot-assisted retinal surgery, the RCM allows the surgical instrument to rotate around a distal fixed point without any lateral translations (24). The RCM is a mechanical property in the case of a hardware-based and/or mechanical RCM or a control approach in the case of a software-based RCM; it acts as a rotational fulcrum and restricts the translation of the end effector along the trocar axis except for the depth of penetration (25,26). This robotic system was designed with a unique software-based RCM, with a trocar as the virtual point position of the RCM, thereby reducing scleral traction, compression, and related complications caused by surgical instruments. In addition, to realign the RCM, the calculation of the new RCM took about 5 ms, and the communication time from the force sensor to the control board was less than 1 ms. During the master-slave control period, the new RCM point used at the next step was calculated using the force value, and the positions of the joints were read at the previous step. Thus, the lag time of the RCM realignment was only 6 ms, which could hardly influence the whole control loop.
Our results obtained during the simulator experiments demonstrated that the accuracy of robot-assisted surgery was significantly higher than that of manual surgery, both in the resident and vitreoretinal surgeon groups. Robot-assisted surgery also effectively reduced physiological tremors and improved surgeons’ control over the stability of the instrument tips. Experienced vitreoretinal surgeons acquire delicate microsurgical techniques through lifelong skills acquisition, which is reflected in the steep learning curve of junior ophthalmologists (27). Our results showed that robots helped residents to quickly achieve performance comparable to that of experts in terms of the stability of holding instruments, thereby improving their surgical skills and surgical safety.
There is some evidence that skills demonstrated on simulators correlate with real-life surgical performance (18). However, there is a large difference between a bionic eyeball in the simulator and a real eyeball. We cannot rule out that robot-assisted surgery will lead to different results in a simulated environment compared with real life. Therefore, we performed retinal vascularization with microneedles in harvested porcine eyes. The results showed that robots provided an efficient approach to sophisticated vitreoretinal surgeries, which might no longer be challenging for residents. Due to the high accuracy of the robot and the advantages of the RCM strategy, the robot’s performance was more stable during the entire surgical process, especially during the injection process following intubation.
At present, the efficiency of robot-assisted surgery is still far lower than that of manual surgery. In Test 1 and Test 2, in both the vitreoretinal expert group and the resident group, robot-assisted surgeries took longer than manual surgery. This is mainly because when approaching the target area (such as the target sphere in Test 1), the displacement accuracy of each step of the robot was 10 µm, which increased time consumption. Considering both accuracy and efficiency of robotic surgery, we set up multiple motion modes, such as intraocular and extraocular motion modes. Moreover, for intraocular motion the displacement speed was divided into 3 gears: high, medium, and low. However, even with these settings, the time it took to switch modes made robot-assisted surgery more time-consuming. In a clinical scenario, the more time a procedure takes, the more risks there are, such as the risks of anesthesia. Therefore, more research is needed on the efficiency of robotics-assisted surgery.
Conclusions
Robots enhance a surgeon’s ability to maintain the stability of instrument handling and the accuracy of surgery, especially for residents, but robot-assisted surgery is less efficient than manual surgery. Robot-assisted surgeries have the potential to improve the precision and safety of surgery by overcoming the physiological limits of physicians.
Acknowledgments
We thank all the residents and vitreoretinal experts who participated in this study.
Funding: This study was funded by the National Natural Science Foundation of China (No. 82171035). The project was supported by Hainan Province Clinical Medical Center.
Footnote
Reporting Checklist: The authors have completed the CONSORT reporting checklist. Available at https://atm.amegroups.com/article/view/10.21037/atm-22-1315/rc
Trial Protocol: Available at https://atm.amegroups.com/article/view/10.21037/atm-22-1315/tp
Data Sharing Statement: Available at https://atm.amegroups.com/article/view/10.21037/atm-22-1315/dss
Peer Review File: Available at https://atm.amegroups.com/article/view/10.21037/atm-22-1315/prf
Conflicts of Interest: All authors have completed the ICMJE uniform disclosure form (available at https://atm.amegroups.com/article/view/10.21037/atm-22-1315/coif). SL is the robot engineer in the Guangzhou Oculotronics Medical Instrument Co., Ltd. PY is the medical manager in Cloud Intelligent Care Technology (Guangzhou) Co., Ltd. The other authors have no conflicts of interest to declare.
Ethical Statement: The authors are accountable for all aspects of the work in ensuring that questions related to the accuracy or integrity of any part of the work are appropriately investigated and resolved. Because of the intervention object was surgical simulator, the ethics committee of ZOC adjudicated that ethics review approval was not required for this study. The principles outlined in the Declaration of Helsinki (as revised in 2013) were followed throughout the study. The porcine eyes were slaughterhouse materials. Experiments were performed under a project license (No. 2019-146) granted by the Institutional Animal Care and Use Committee of ZOC, in compliance with the Association for Research in Vision and Ophthalmology guidelines for the care and use of animals.
Open Access Statement: This is an Open Access article distributed in accordance with the Creative Commons Attribution-NonCommercial-NoDerivs 4.0 International License (CC BY-NC-ND 4.0), which permits the non-commercial replication and distribution of the article with the strict proviso that no changes or edits are made and the original work is properly cited (including links to both the formal publication through the relevant DOI and the license). See: https://creativecommons.org/licenses/by-nc-nd/4.0/.
References
- Billard A, Kragic D. Trends and challenges in robot manipulation. Science 2019;364:eaat8414. [Crossref] [PubMed]
- Roizenblatt M, Grupenmacher AT, Belfort R Junior, et al. Robot-assisted tremor control for performance enhancement of retinal microsurgeons. Br J Ophthalmol 2019;103:1195-200. [Crossref] [PubMed]
- Estai M, Bunt S. Best teaching practices in anatomy education: A critical review. Ann Anat 2016;208:151-7. [Crossref] [PubMed]
- Jacofsky DJ, Allen M. Robotics in Arthroplasty: A Comprehensive Review. J Arthroplasty 2016;31:2353-63. [Crossref] [PubMed]
- Tokas T, Gözen AS, Avgeris M, et al. Combining of ETHOS Operating Ergonomic Platform, Three-dimensional Laparoscopic Camera, and Radius Surgical System Manipulators Improves Ergonomy in Urologic Laparoscopy: Comparison with Conventional Laparoscopy and da Vinci in a Pelvi Trainer. Eur Urol Focus 2017;3:413-20. [Crossref] [PubMed]
- Keating MF, Zhang J, Feider CL, et al. Integrating the MasSpec Pen to the da Vinci Surgical System for In Vivo Tissue Analysis during a Robotic Assisted Porcine Surgery. Anal Chem 2020;92:11535-42. [Crossref] [PubMed]
- Yang Y, Song L, Huang J, et al. A uniportal right upper lobectomy by three-arm robotic-assisted thoracoscopic surgery using the da Vinci (Xi) Surgical System in the treatment of early-stage lung cancer. Transl Lung Cancer Res 2021;10:1571-5. [Crossref] [PubMed]
- Gehlbach PL. Robotic surgery for the eye. Nat Biomed Eng 2018;2:627-8. [Crossref] [PubMed]
- de Smet MD, Naus GJL, Faridpooya K, et al. Robotic-assisted surgery in ophthalmology. Curr Opin Ophthalmol 2018;29:248-53. [Crossref] [PubMed]
- Ahronovich EZ, Simaan N, Joos KM. A Review of Robotic and OCT-Aided Systems for Vitreoretinal Surgery. Adv Ther 2021;38:2114-29. [Crossref] [PubMed]
- Yang S, Martel JN, Lobes LA Jr, et al. Techniques for robot-aided intraocular surgery using monocular vision. Int J Rob Res 2018;37:931-52. [Crossref] [PubMed]
- Yang S, MacLachlan RA, Martel JN, et al. Comparative Evaluation of Handheld Robot-Aided Intraocular Laser Surgery. IEEE Trans Robot 2016;32:246-51. [Crossref] [PubMed]
- Chen YQ, Tao JW, Li L, et al. Feasibility study on robot-assisted retinal vascular bypass surgery in an ex vivo porcine model. Acta Ophthalmol 2017;95:e462-7. [Crossref] [PubMed]
- Ebrahimi A, Urias MG, Patel N, et al. Adaptive Control Improves Sclera Force Safety in Robot-Assisted Eye Surgery: A Clinical Study. IEEE Trans Biomed Eng 2021;68:3356-65. [Crossref] [PubMed]
- Channa R, Iordachita I, Handa JT. Robotic Vitreoretinal Surgery. Retina 2017;37:1220-8. [Crossref] [PubMed]
- Bakshi SK, Lin SR, Ting DSW, et al. The era of artificial intelligence and virtual reality: transforming surgical education in ophthalmology. Br J Ophthalmol 2021;105:1325-8. [Crossref] [PubMed]
- Thomsen AS, Bach-Holm D, Kjærbo H, et al. Operating Room Performance Improves after Proficiency-Based Virtual Reality Cataract Surgery Training. Ophthalmology 2017;124:524-31. [Crossref] [PubMed]
- Jacobsen MF, Konge L, Bach-Holm D, et al. Correlation of virtual reality performance with real-life cataract surgery performance. J Cataract Refract Surg 2019;45:1246-51. [Crossref] [PubMed]
- Li Y, Hu J, Cao D, et al. Optical-Waveguide Based Tactile Sensing for Surgical Instruments of Minimally Invasive Surgery. Front Robot AI 2021;8:773166. [Crossref] [PubMed]
- Wilson JT, Gerber MJ, Prince SW, et al. Intraocular robotic interventional surgical system (IRISS): Mechanical design, evaluation, and master-slave manipulation. Int J Med Robot 2018;14: [Crossref] [PubMed]
- Gijbels A, Smits J, Schoevaerdts L, et al. In-Human Robot-Assisted Retinal Vein Cannulation, A World First. Ann Biomed Eng 2018;46:1676-85. [Crossref] [PubMed]
- Guo S, Wang Y, Xiao N, et al. Study on real-time force feedback for a master-slave interventional surgical robotic system. Biomed Microdevices 2018;20:37. [Crossref] [PubMed]
- Zuo S, Wang Z, Zhang T, et al. A novel master-slave intraocular surgical robot with force feedback. Int J Med Robot 2021;17:e2267. [Crossref] [PubMed]
- Su H, Hu Y, Karimi HR, et al. Improved recurrent neural network-based manipulator control with remote center of motion constraints: Experimental results. Neural Netw 2020;131:291-9. [Crossref] [PubMed]
- Zhou M, Wu J, Ebrahimi A, et al. Spotlight-based 3D Instrument Guidance for Autonomous Task in Robot-assisted Retinal Surgery. IEEE Robot Autom Lett 2021;6:7750-7. [Crossref] [PubMed]
- Sun Y, Pan B, Guo Y, et al. Vision-based hand-eye calibration for robot-assisted minimally invasive surgery. Int J Comput Assist Radiol Surg 2020;15:2061-9. [Crossref] [PubMed]
- Jacobsen MF, Konge L, la Cour M, et al. The learning curve of robot-assisted vitreoretinal surgery - A randomized trial in a simulated setting. Acta Ophthalmol 2021;99:e1509-16. [Crossref] [PubMed]