This article has an erratum available at: http://dx.doi.org/10.21037/atm-2024-39 the article has been update on 2024-10-18 at here.
Mesenchymal stem cells alleviate aging in vitro and in vivo
Introduction
Aging is a natural and multi-factorial phenomenon characterized by the accumulation of degenerative processes, reduction of individual organ function, unbalanced homeostasis of the internal environment, decline in resistance, and gradual trajectory towards death with increasing age (1,2). More than five decades ago, Leonard Hayflick and Paul Moorhead reported the finite proliferative capacity of normal human fibroblasts, a phenomenon that they defined as ‘cellular senescence’, and speculated that it could be an underlying cause of aging (3).
With the increase of the aged population, aging and aging-related diseases have become a major global challenge (4,5). Over the last century, chronic health problems have replaced infectious diseases as the dominant health care burden. Aging is the most profound risk factor for almost all non-communicable diseases, such as cardiovascular diseases (6,7), neurological diseases (8,9), type II diabetes (10), fibrosis (11), and osteoporosis (12).
For several decades, understanding aging and the mechanisms underlying aging processes that limit lifespan has challenged biologists. The proposed mechanisms that contribute to the aging process and the development of age-associated diseases involve DNA damage, alterations in gene and non-coding RNA expression, oxidative stress, genotoxicity, and the incidence of shorter telomeres (13-15). However, none of the theories have been able to perfectly explain the potential mechanisms in the process of aging due to the result of complex interactions involving multiple pathways and molecules (1,16).
Mesenchymal stem cells (MSCs), which were originally called osteogenic precursor cells, were first reported by Friedenstein et al. in 1976 (17). Later, MSCs were found to possess the potential for differentiation of a variety of cells and were named MSCs by Caplan (18). Predominantly, MSCs are distributed in connective tissues and organ stroma, especially in the bone marrow. As an important member of the stem cell family, MSCs have the ability of self-renewal and the potential for multidirectional differentiation (19). They possess immunomodulatory properties, which regulate the proliferation and immune reconstruction of immune cells through intercellular interaction or regulation of various cytokines (20,21). In addition, MSCs have certain advantages in tissue damage repair, hematological diseases, and clinical application due to their ease of isolation and culture and no or low level of immune rejection. Although MSCs have been used in a variety of diseases, including pulmonary fibrosis (22), spinal cord injury (23), fatty liver (24), and optic nerve injury (25), little is known about their anti-aging effects. In particular, a systemic study of the role of MSCs in treatment of aging and aging-related conditions has not been conducted.
In the present study, we investigated the effects of MSCs on aging in both aged cells and mice, which revealed that MSCs can not only inhibit the aging-related secretory phenotype at the cellular level but also alleviate the aging phenotype of various organs and prolong the life of mice. We present the following article in accordance with the ARRIVE reporting checklist (available at https://atm.amegroups.com/article/view/10.21037/atm-22-1206/rc).
Methods
Animals
The C57BL/6 male mice were purchased from Sipeifu Biological Co., Ltd. (Beijing, China) and fed in separate cages in a temperature-controlled environment with a 12:12 hour light-dark cycle, with water and food ad libitum. There was potential for the mice to have died due to aging during the period of the experiment. Their body weight and mortality were recorded weekly until 24 months. All procedures of mice were performed under the approval of the Institute of Biophysics Committee for Animal Care (Approval No. SYXK2020053), in adherence with the Guide for the Care and Use of Laboratory Animals published by the U.S. National Institutes of Health (8th edition, revised in 2011).
IMR-90 cell culture and cell senescence induction
The IMR-90 cells were kindly provided by the Stem Cell Bank, Chinese Academy of Sciences (Beijing, China) and cultured in Dulbecco’s modified Eagle’s high-glucose medium (DMEM/HD) supplemented with 10% fetal bovine serum (FBS), 100 U/mL penicillin, and 100 µg/mL streptomycin at 37 ℃ and 5% CO2. Cells were passaged with the ratio of 1:3 when the confluence degree reached 80–90%. The cell-aging model was induced by adding 10 g/L D-galactose (#G0750, Sigma-Aldrich, St. Louis, MO, USA) to the culture medium for 3 generations.
Cell doubling proliferation assay
Control and senescent IMR-90 cells induced by D-gal were seeded in a 6 cm culture dish at a density of 105 cells/dish. The cells were counted every 3 days and passaged at a cell density of 105 cells/dish for up to 18 days. The values of the cell population doublings (PD) were calculated by the formula PD = log (NF/N0)/log2, where NF represents the number of final cells and N0 represents the number of initial cells.
SA-β-galactosidase staining
The β-galactosidase staining was performed using the Senescence β-Galactosidase Staining Kit [#9860s, Cell Signaling Technologies (CST), Danvers, MA, USA] according to the manufacturer’s instructions. In brief, cells were washed using phosphate-buffered saline (PBS) and fixed with 4% paraformaldehyde (PFA). The cells were then stained in freshly prepared 5-bromo-4-chloro-3-indolyl-β-D-galactopyranoside (X-Gal) staining solution and incubated at 37 ℃, and protected from light for 8–12 hours. Cells were then washed with PBS, imaged, and analyzed using ImageJ software (National Institutes of Health, Bethesda, MA, USA, https://imagej.nih.gov/ij/).
Isolation and culture of MSCs
White and brown adipose tissues were harvested from the groin and posterior neck of the C57BL/6J male mice at the age of 6–8 weeks. After being washed 2 times with ice-cold PBS, the lymph nodes and small blood vessels were dissected from white adipose tissue, and the white adipose tissue surrounding the brown adipose tissue was dissociated. The adipose tissue was then mechanically diced, digested, washed, filtered, and centrifuged. Bone marrow was extracted using a syringe with DMEM/HD from the thigh bone of the 6–8-week-old mice C57BL/6J male mice, and subsequently washed, filtered, and centrifuged. The adipose cell pellet and the bone marrow cell pellet were washed with DMEM/F12 and DMEM/HD one more time and then resuspended in the medium supplemented with 10% FBS, 100 U/mL penicillin, and 100 µg/mL streptomycin. Finally, MSCs were placed in a 10 cm dish and cultured in an incubator at 37 ℃ with 5% CO2.
Flow cytometry analysis
To confirm the purity of the MSCs, the expression of cell surface markers on the MSCs was evaluated by flow cytometry. Fluorescein isothiocyanate (FITC)-conjugated CD44, eFluor® 450-conjugated CD34, APC-conjugated CD105, and PE-conjugated CD73 and CD90.2 antibodies were purchased from Thermo Fisher Scientific Inc. (Waltham, MA, USA; #11-0441-81, #48-0341-80, #17-1051-80, #12-0731-81, and #12-0903-81, respectively). The cells were incubated with antibodies against the cell surface markers in the dark for 30 minutes and then subjected to flow cytometry analysis.
IMR-90 cells co-cultured with MSCs
When the confluence of the 3 MSCs and IMR-90 senescent cells reached 80–90%, they were inoculated into the lower hole and the upper chamber of the Transwell dishes (#3412; Corning, NY, USA), respectively. The IMR-90 senescent cells and MSCs were co-cultured in Transwell dishes for 48 hours followed by the isolation of total RNA.
Quantitative polymerase chain reaction (qPCR)
Total RNA was extracted from IMR-90 cells using Trizol reagent according to the manufacturer’s instructions (#R0016; Beyotime, Beijing, China). The total RNA was purified by successive treatments with chloroform (#151858, Sigma-Aldrich, USA), isopropanol (#I9516; Sigma-Aldrich, USA), and 75% ethanol (#E7023, Sigma-Aldrich, USA). Then, 1 µg of total RNA was reverse-transcribed using PrimeScript™ RT Reagent Kit with gDNA Eraser (#RR047A; TaKaRa, Dalian, China) for complementary DNA (cDNA) synthesis. The quantitative polymerase chain reaction (qPCR) assay was performed using the SYBR Premix Ex Taq™ II Kit (#RR820DS; TaKaRa,China). Relative expression of the target gene was calculated by the Comparative Ct (2−ΔΔCt) method with β-actin as a reference control. The primers used for the amplification are listed in Table 1.
Table 1
Name | Forward primer | Reverse primer |
---|---|---|
IL-6 | TAGTCCTTCCTACCCCAATTTCC | TTGGTCCTTAGCCACTCCTTC |
TGFβ1 | CTCCCGTGGCTTCTAGTGC | GCCTTAGTTTGGACAGGATCTG |
GDF11 | CTGCGCCTAGAGAGCATCAAG | TCTCGGTGGTAGCGTGGTA |
p21 | AGGTGGACCTGGAGACTCTCAG | TCCTCTTGGAGAAGATCAGCCG |
CBX4 | GCTGCTGATCGCCTTCCAGAAC | TTGGAACGACGGGCAAAGGTAG |
ND1 | TAATCGCCATAGCCTTCCTAACA | GGCGTCTGCAAATGGTTGTAA |
FOXO1 | CTACGAGTGGATGGTCAAGAGC | CCAGTTCCTTCATTCTGCACACG |
FOXO3 | TCTACGAGTGGATGGTGCGTTG | CTCTTGCCAGTTCCCTCATTCTG |
FOXO4 | ACGAGTGGATGGTCCGTACTGT | CCTTGATGAACTTGCTGTGCAGG |
β-actin | TCCACACCCGCCACCAG | TGACCCATTCCCACCATCACA |
IL-6, Interleukin-6; TGFβ1, transforming growth factor β1; GDF11, growth differentiation factor 11; CBX4, Chromobox 4; ND1, NADH-ubiquinone oxidoreductase chain 1; FOXO, Forkhead.
Mice treated with MSCs
Mice were randomly treated with MSCs (16 mice) or PBS (16 mice) at the age of 20 months via tail-vein injection twice a month (1×106 cells at a time) for up to 4 months. After 4 months of treatment, mice were sacrificed by CO2 inhalation, and tissues of mice were harvested to be tested and defined at the age of 6 months as the young controls (4 mice).
Urine spot assay
The aging mice at the age of 23 months were treated with bone marrow mesenchymal stem cells (BMSCs) or PBS for 3 months and the young mice at the age of 5 months were adopted to perform the urine spot assay. The mice were placed in a carton covered with a layer of A4 paper. After free movement for 3 hours, the A4 paper was taken out, dried, and photographed under ultraviolet (UV) light. Finally, the number of urinary spots was counted using the ImageJ software.
Histopathological staining
After perfusion with PBS, the tissues of the mice were harvested, washed, fixed with 4% PFA, dehydrated with 60% alcohol, and embedded in paraffin. Paraffin sections (5 µm) were immersed for 10 minutes in xylene 3 times and then 100%, 100%, 90%, 80%, and 70% alcohol for 5 minutes for dewaxing and rehydration. The sections were soaked in hematoxylin and eosin (H&E) stain (#C0105; Beyotime, Shanghai, China) successively. Masson’s trichrome staining kit (#DC0033, Leagene Biotechnology Co., Ltd., Beijing, China) was used to perform the Masson staining. Then, sections were immersed in gradient alcohol and xylene solution for dehydration and transparency. Finally, the samples were observed under a light microscope after being sealed with neutral balsam on slides.
Measurement of malondialdehyde (MDA) content
A Lipid Peroxidation MDA Assay Kit (#S0131, Beyotime, China) was used to measure the content of MDA. Briefly, tissues were homogenized using the lysis buffer (#P0013, Beyotime, China) and the supernatant was collected. The supernatant was mixed with freshly prepared MDA solution, and the mixture was heated at 100 ℃ for 15 minutes and centrifuged at 1,000 g at room temperature for 10 minutes. Then, 200 µL of supernatant was added to the 96-well plate, and the absorbance at 532 nm was measured to calculate the content of MDA.
Statistical analysis
All values are expressed as mean ± standardized error of the mean (SEM). Differences in population doublings and MDA content were analyzed using two-way analysis of variance (ANOVA) followed by Dunn’s multiple comparisons test; differences in survival rate were analyzed using the Log-rank (Mantel-Cox) test; differences in other data types were analyzed using one-way ANOVA followed by Dunn’s multiple comparisons test. All the data were accessed using GraphPad Prism 9 (GraphPad Software Inc., San Diego, CA, USA). Levels of statistical significance were defined as follows: *, P<0.05, **, P<0.01, and ***, P<0.001.
Results
Preparation of senescent cells and mesenchymal stem cells
In order to investigate the effect of MSCs on aged cells, a model of IMR-90 cell senescence was created by D-gal induction, and the isolation and preparation of the BMSCs, brown adipose mesenchymal stem cells (BAMSCs), as well as white adipose mesenchymal stem cells (WAMSCs) stem cells were conducted. As seen in Figure 1, the number of SA-β-gal positive cells induced by D-gal was significantly increased compared with the control cells (Figure 1A,1B). The results of the cell doubling experiment showed that the proliferation rate of the senescent cells was significantly lower than that of the control cells (Figure 1C). These results indicated that the senescent cell model had been successfully established.
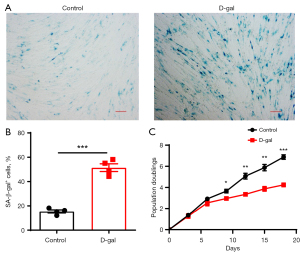
Next, to determine the quality of the BMSCs, BAMSCs, and WAMSCs, the MSCs were identified by flow cytometry loaded with anti-surface antigen. As shown in Figure S1, for the BMSCs, CD44, CD105, CD90.2, and CD34 positive cells accounted for 93.4%, 99.3%, 99.5%, and 3.51%, respectively, of the total cells, the percentages of CD44, CD105, CD73, and CD34 positive cells in the BAMSCs were 90.8%, 93.6%, 88.9%, and 2.37% respectively, and the results of the identification for the WAMSCs were similar to those of the BAMSCs. These results suggested that the preparation of the MSCs was qualified for our experiments.
MSCs alleviate D-gal-induced IMR-90 cell senescence
Next, the effects of MSCs derived from different tissues on aged IMR-90 cells, a classic senescent cell model, were examined and compared. First, the expression of aging related factors was detected after co-culturing D-gal-induced senescent cells with MSCs for 48 hours. As shown in Figure 2A, Figure S2A, the expression of inflammatory factor interleukin-6 (IL-6) in D-gal-induced senescent cells was significantly higher than that in the control group, and the increase in the IL-6 expression levels was significantly decreased by all three types of MSCs. Although it has been reported that up-regulated transforming growth factor β1 (TGFβ1) and down-regulated growth differentiation factor 11 (GDF11) are related to aging, the evidence is controversial (1,26). Thus, we then detected the expressions of TGFβ1 and GDF11, which revealed that the expressions of TGFβ1 and GDF11 were decreased in D-gal-induced aged cells, compared to controls. The decreased expressions of TGFβ1 and GDF11 were significantly reversed by BAMSC and BMSC treatment; WAMSC treatment only increased the expression of TGFβ1 but not GDF11 (Figure 2B,2C, and Figure S2B,S2C).
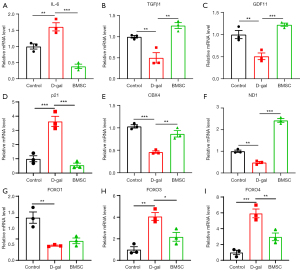
It is well known that p21 is a remarkable marker for senescent cells, and the expression level of p21 is significantly increased in senescent cells. Thus, we next examined the expression of p21. As shown in Figure 2D and Figure S2D, the expression level of p21 was significantly up-regulated in D-gal-induced aged cells. The up-regulated p21 level was effectively down-regulated by all the 3 types of MSCs, suggesting that MSCs have an anti-senescence function in the D-gal-induced aged cells. Chromobox 4 (CBX4), a component of the polycomb repressive complex 1 (PRC1), plays an important role in the maintenance of nucleolar homeostasis to alleviate senescence (27). Therefore, we detected the expression of CBX4, and the result showed a remarkable decrease in D-gal-induced aged cells, and the decreased CBX4 was increased by co-culture of the cells with BMSCs; however, BAMSCs and WAMSCs treatment failed to reverse the decrease of CBX4 expression in aged cells (Figure 2E, Figure S2E).
To understand the role and mechanism of mitochondria in aged cells and to determine the effect of MSCs on mitochondrial dysfunction caused by cell senescence, we detected the expression of NADH-ubiquinone oxidoreductase chain 1 (ND1), a gene which is located in mitochondria and represents the copy number of mitochondria. As seen in Figure 2F and Figure S2F, ND1 expression was significantly decreased in IMR-90 aged cells, and the decrease of ND1 expression was reversed by MSCs treatment.
Forkhead (FOXO) family members 1, 3, and 4 are known to be related to aging. Both of FOXO1 and FOXO3 are recognized as longevity factors and are involved in the proteasome system degradation of short-lived and regulatory cytosolic proteins (28,29). Therefore, we subsequently, tested the messenger RNA (mRNA) level of these 3 members of the FOXO family. The results indicated that the expression of FOXO1 statically significantly decreased. Conversely, the expression of FOXO3 and FOXO4 increased in aged cells induced by D-gal. The changes of FOXO family members’ expression levels in D-gal-induced aged cells were able to be altered by MSCs treatment; BAMSCs and WAMSCs reversed the down-regulation of FOXO1 expression, and BAMSCs and BMSCs treatment reduced the up-regulated expression of FOXO3 and FOXO4 (Figure 2G-2I, Figure S2G-S2I).
MSCs improve aging-related changes in mice
The results we have described above indicate that MSCs derived from different tissues can effectively improve cell senescence induced by D-gal, and BMSCs seem to be superior to the other two types of MSCs, to a certain extent. In order to further investigate and confirm their anti-aging role in vivo, we conducted experiments in young and aged mice. We treated both 20-month-old mice (defined as aged mice) and 6-month-old mice (defined as young mice) with MSCs/PBS and PBS, respectively. Mice were injected twice a month via the tail vein for up to 4 months. As shown in Figure 3A, compared to the young mice, all the aged mice showed an age-related sign of sparse, dull, and grey hairs. After 4 months of treatment with BMSCs, the dull, sparse, and grey hairs were restored to their normal state. The observation of BMSCs treatment on the survival rate of the aged mouse showed that the mortality of the aged mice treated with BMSCs was significantly lower than that in the PBS group. At the endpoint of the experiment, the survival rate of mice in the BMSCs treatment group was 66.67%, but only 40% in the PBS group (Figure 3B). Figure 3C,3D shows another aging-related feature observed in this study, bladder overactivity. It is known that the incidence of bladder overactivity increases with aging (30,31). An important sign of bladder overactivity is the significant increase in the frequency of urination. The experimental results indicated that the urine spot number (the frequency of urination) was markedly increased, but the volume of urine per session was significantly reduced in the aged mice. This phenomenon in the aged mice was able to be reversed with BMSCs treatment.
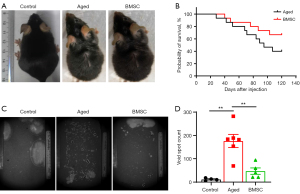
To confirm our findings described above, we harvested and analyzed different tissues from young and aged mice for analysis with H&E and Masson trichrome staining. Skin images showed that the hair follicles in the older mice were swollen, inactivated, and reduced in number, and the subcutaneous fibers were also reduced. After treatment with BMSCs, all the abnormal phenomena observed in the skin images of the old mice were greatly improved (Figure 4A).
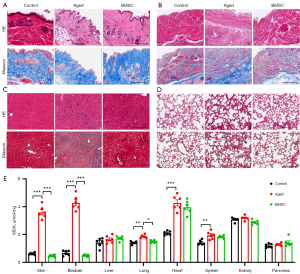
The staining results of bladder tissue showed obvious inflammatory cell infiltration, significant muscle fiber loss, and surplus of extracellular matrix, such as collagen fibers, in the aged mice compared with those in the control group. This change in the aged mouse bladder was significantly reversed by BMSCs treatment (Figure 4B).
Next, we observed the pathological characteristics of the liver and found that there were obvious inflammatory infiltration and fiber deposition in the livers of aged mice, which were alleviated with BMSCs treatment (Figure 4C). Lung H&E staining indicated that BMSCs treatment significantly alleviated the increase of protein exudation in old mice, and no obvious fiber deposition was observed in the lungs of the 3 groups of mice by Masson’s trichrome staining (Figure 4D).
It is known that oxidative stress is also closely related to aging. To access the degree of oxidative stress, we detected the content of MDA, the end product of fatty acid β-oxidation, in various organs. As shown in Figure 4E, MDA in the bladder, skin, heart, spleen, and lung of the aged mice was significantly higher than that of the young mice. Treatment with BMSCs significantly reduced the amount of MDA in the tissues mentioned above, except in the heart and spleen.
Discussion
Aging and age-related diseases are serious problems faced by developed countries today, for which there is currently no satisfactory solution. Therefore, research on aging and anti-aging has received extensive attention. In the present study, we investigated the roles and the possible mechanisms of MSCs on aging and aging-related changes in D-gal-induced aging cells and naturally aged mice. Our in vitro and in vivo results suggest that stem cell therapy can alleviate aging and aging-related changes, providing a new idea and potential approach for the prevention and treatment of aging and aging-related complications.
Multiple stressors and damaging agents activate, or trigger, the signaling cascades that converge on the activation of cell cycle inhibitors and tumor suppressors, including the DNA-damage response (DDR) activated by DNA damage agents and telomere loss, the epigenetic derepression of the cyclin-dependent kinase inhibitor 2A (CDKN2A) locus, the reactive oxygen species (ROS), the oncogenic signal or loss of tumor suppressors, and the senescence-associated secretory phenotype (SASP) pathway. The molecular mechanism underlying these processes contributes to the aging of these triggers, including directly activating p53 and its downstream transcriptional target p21 or indirectly activating the p53 and p21 through the kinases MAPKK3 and MAPKK6, p53 activator alternative reading frame (ARF), and the TGFβ/SMAD pathways (32-34).
Our study showed that MSCs alleviated cell aging by inhibiting the SASP component IL-6, improving mitochondrial homeostasis, and downregulating the expression of p21. However, our result of decreased TGFβ1 expression in the senescent cells was the opposite to those of a majority of studies, suggesting that TGFβ1 plays a vital role in the initial onset of aging, and the TGFβ1 expression level shows dynamic changes during the occurrence and development of aging. There are some researchers who claim that TGFβ1, as an anti-inflammatory cytokine, plays an essential role in inflammation, including the maintenance of immune response homeostasis and the prevention of undesirable self-targeted responses (35). Additionally, our finding that MSCs treatment results in the increased expression of TGFβ1 is consistent with that reported by a Japanese team, who found that serum TGFβ1 levels inversely decreased as age advanced (36). As a member of the TGF superfamily, GDF11 possesses anti-aging and pro-regenerative activities in heart, skeletal muscle, and blood vessel tissue of aged mice. While our results are consistent with previous studies indicating that GDF11 is down-regulated in senescent cells (37), the effects of GDF11 in the aging/rejuvenation process are also controversial (38,39). Here, we demonstrated that the down-regulated GDF11 in senescent cells was able to be reversed by MSCs treatment.
The MSCs derived from various sources have pluripotent capacity and the applicability of MSCs depends on the cell source and their different functions in vivo, despite having similar phenotypic and cytological characteristics. Although some studies have suggested that MSCs derived from bone and adipose (AMSCs) have similar therapeutic effects on hindlimb ischemic disease (40), other studies have suggested that BMSCs might be preferred over AMSCs in clinical application for therapeutic angiogenesis (41). The MSCs have been widely used in the treatment of aging-related diseases (42). Transplantation of MSCs alleviates some aging-associated phonotypes, including the loss of bone density (43), cardiac dysfunction (44), chronic injury of the spinal cord (23), and facial skin aging (45). However, few studies have comparatively studied the anti-aging efficacy of MSCs derived from different tissue sources. Here, we compared the anti-aging effects of MSCs from bone marrow, white fat, and brown fat in vitro, respectively. The results showed that the anti-aging effect of BMSCs was superior to a certain extent, with more aging-related genes corrected. The proliferation and aging of MSCs themselves are the key to their therapeutic effect. Studies have shown that BMSCs have higher proliferative ability and lower expression of aging-related factors than AMSCs in the first 5 generations (46). Based on the results at the cellular level, BMSCs have been used for in vivo studies in aged mice. We found that BMSCs attenuated the aging-related symptoms of mice, with the hair of mice denser and shinier, the overactive bladder symptoms less marked (30,47), and the survival rate of mice significantly increased (from 40% to 66.67%) at the 24th month. Additionally, BMSCs treatment was able to improve the structural changes of the skin, bladder, liver, and lungs of aging mice.
The mechanism underlying MSCs therapy includes, but is not limited to, damaged tissue regeneration, immunomodulation, and secretion of paracrine mediators. Paracrine effectors include soluble cytokines, growth factors, hormones, and microRNA (miRNA). They are transferred to target cells through the uptake of biologic-loaded extracellular vehicles (EVs) and immune-mediated phagocytosis (48-50). However, the anti-aging mechanism of MSCs is not clear. In the present study, in vitro anti-aging of MSCs was mainly manifested by inhibiting the secretion of SASP, increasing the release of growth factors, and reducing the expression of aging-related genes. Moreover, we inferred that the mechanism underlying the anti-aging effect of MSCs is to maintain the homeostasis and function of mitochondria due to the result that MSCs increased the level of ND1, which represents the number of mitochondria, in senescent cells. Telomere shortening is considered to be positively correlated with aging (14,51). Our study also showed that MSCs prolonged the telomere length of D-gal-induced senescent cells (data not shown). In the in vivo study, we found that BMSCs transplantation reduced the content of MDA and improved the state of oxidative stress of mitochondria in aging mice, suggesting oxidative stress reduction may be one of the anti-aging mechanisms of BMSCs. Therefore, in our study, the anti-aging mechanisms of MSCs mainly involved the maintenance of mitochondrial homeostasis and function, the extension of telomere length, and the reduction of oxidative stress. However, the detailed molecular mechanisms and pathways of the anti-aging effects of MSCs need to be further investigated.
Conclusions
In summary, our study indicates that MSCs treatment effectively improves aging-related phenotypes, both in vitro and in vivo. The underlying mechanism appears to be associated with the maintenance of mitochondrial homeostasis, the extension of telomere length, and the reduction of oxidative stress. Our results suggest that MSCs transplantation may be a potential therapeutic approach for aging and aging-related diseases, and we believe that MSCs also have great prospects in the clinical treatment of aging-related diseases.
Acknowledgments
Funding: This work was supported by grants from the National Key Research and Development Project (No. 2019YFA0110400 to GJ) and the National Foundation of Sciences and Technology (Nos. 31971051, 31771562 to GJ).
Footnote
Reporting Checklist: The authors have completed the ARRIVE reporting checklist. Available at https://atm.amegroups.com/article/view/10.21037/atm-22-1206/rc
Data Sharing Statement: Available at https://atm.amegroups.com/article/view/10.21037/atm-22-1206/dss
Peer Review File: Available at https://atm.amegroups.com/article/view/10.21037/atm-22-1206/prf
Conflicts of Interest: All authors have completed the ICMJE uniform disclosure form (available at https://atm.amegroups.com/article/view/10.21037/atm-22-1206/coif). The authors have no conflicts of interest to declare.
Ethical Statement: The authors are accountable for all aspects of the work in ensuring that questions related to the accuracy or integrity of any part of the work are appropriately investigated and resolved. All procedures of mice were performed under approval of the Institute of Biophysics Committee for Animal Care (Approval No. SYXK2020053), in adherence with the Guide for the Care and Use of Laboratory Animals published by the U.S. National Institutes of Health (8th edition, revised 2011).
Open Access Statement: This is an Open Access article distributed in accordance with the Creative Commons Attribution-NonCommercial-NoDerivs 4.0 International License (CC BY-NC-ND 4.0), which permits the non-commercial replication and distribution of the article with the strict proviso that no changes or edits are made and the original work is properly cited (including links to both the formal publication through the relevant DOI and the license). See: https://creativecommons.org/licenses/by-nc-nd/4.0/.
References
- Wagner KH, Cameron-Smith D, Wessner B, et al. Biomarkers of Aging: From Function to Molecular Biology. Nutrients 2016;8:338. [Crossref] [PubMed]
- Kirkwood TB. Understanding the odd science of aging. Cell 2005;120:437-47. [Crossref] [PubMed]
- Hayflick L, Moorhead PS. The serial cultivation of human diploid cell strains. Exp Cell Res 1961;25:585-621. [Crossref] [PubMed]
- Partridge L, Deelen J, Slagboom PE. Facing up to the global challenges of ageing. Nature 2018;561:45-56. [Crossref] [PubMed]
- Marengoni A, Angleman S, Melis R, et al. Aging with multimorbidity: a systematic review of the literature. Ageing Res Rev 2011;10:430-9. [Crossref] [PubMed]
- Evans MA, Sano S, Walsh K. Cardiovascular Disease, Aging, and Clonal Hematopoiesis. Annu Rev Pathol 2020;15:419-38. [Crossref] [PubMed]
- Zhang W, Song M, Qu J, et al. Epigenetic Modifications in Cardiovascular Aging and Diseases. Circ Res 2018;123:773-86. [Crossref] [PubMed]
- Wyss-Coray T. Ageing, neurodegeneration and brain rejuvenation. Nature 2016;539:180-6. [Crossref] [PubMed]
- Higgins-Chen AT, Thrush KL, Levine ME. Aging biomarkers and the brain. Semin Cell Dev Biol 2021;116:180-93. [Crossref] [PubMed]
- Halim M, Halim A. The effects of inflammation, aging and oxidative stress on the pathogenesis of diabetes mellitus (type 2 diabetes). Diabetes Metab Syndr 2019;13:1165-72. [Crossref] [PubMed]
- Jia H, Yan Y, Liang Z, et al. Autophagy: A new treatment strategy for MSC-based therapy in acute kidney injury Mol Med Rep 2018;17:3439-47. (Review). [PubMed]
- Pignolo RJ, Law SF, Chandra A. Bone Aging, Cellular Senescence, and Osteoporosis. JBMR Plus 2021;5:e10488. [Crossref] [PubMed]
- Kang C, Xu Q, Martin TD, et al. The DNA damage response induces inflammation and senescence by inhibiting autophagy of GATA4. Science 2015;349:aaa5612. [Crossref] [PubMed]
- Franzke B, Neubauer O, Wagner KH. Super DNAging-New insights into DNA integrity, genome stability and telomeres in the oldest old. Mutat Res Rev Mutat Res 2015;766:48-57. [Crossref] [PubMed]
- Li Z, Zhang Z, Ren Y, et al. Aging and age-related diseases: from mechanisms to therapeutic strategies. Biogerontology 2021;22:165-87. [Crossref] [PubMed]
- Borup MT, Trusina A, Andersson AM. Aging mechanism as the "down side" of adaptation: a network approach. J Theor Biol 2008;250:66-74. [Crossref] [PubMed]
- Friedenstein AJ, Gorskaja JF, Kulagina NN. Fibroblast precursors in normal and irradiated mouse hematopoietic organs. Exp Hematol 1976;4:267-74. [PubMed]
- Caplan AI. Mesenchymal stem cells. J Orthop Res 1991;9:641-50. [Crossref] [PubMed]
- Ankrum JA, Ong JF, Karp JM. Mesenchymal stem cells: immune evasive, not immune privileged. Nat Biotechnol 2014;32:252-60. [Crossref] [PubMed]
- Keating A. Mesenchymal stromal cells: new directions. Cell Stem Cell 2012;10:709-16. [Crossref] [PubMed]
- Fibbe WE, Dazzi F, LeBlanc K. MSCs: science and trials. Nat Med 2013;19:812-3. [Crossref] [PubMed]
- Ni K, Liu M, Zheng J, et al. PD-1/PD-L1 Pathway Mediates the Alleviation of Pulmonary Fibrosis by Human Mesenchymal Stem Cells in Humanized Mice. Am J Respir Cell Mol Biol 2018;58:684-95. [Crossref] [PubMed]
- Li X, Tan J, Xiao Z, et al. Transplantation of hUC-MSCs seeded collagen scaffolds reduces scar formation and promotes functional recovery in canines with chronic spinal cord injury. Sci Rep 2017;7:43559. [Crossref] [PubMed]
- Wang H, Zhang H, Huang B, et al. Mesenchymal stem cells reverse high fat diet induced non alcoholic fatty liver disease through suppression of CD4+ T lymphocytes in mice. Mol Med Rep 2018;17:3769-74. [PubMed]
- Chung S, Rho S, Kim G, et al. Human umbilical cord blood mononuclear cells and chorionic plate-derived mesenchymal stem cells promote axon survival in a rat model of optic nerve crush injury. Int J Mol Med 2016;37:1170-80. [Crossref] [PubMed]
- De Domenico E, D'Arcangelo G, Faraoni I, et al. Modulation of GDF11 expression and synaptic plasticity by age and training. Oncotarget 2017;8:57991-8002. [Crossref] [PubMed]
- Ren X, Hu B, Song M, et al. Maintenance of Nucleolar Homeostasis by CBX4 Alleviates Senescence and Osteoarthritis. Cell Rep 2019;26:3643-3656.e7. [Crossref] [PubMed]
- Willcox BJ, Donlon TA, He Q, et al. FOXO3A genotype is strongly associated with human longevity. Proc Natl Acad Sci U S A 2008;105:13987-92. [Crossref] [PubMed]
- Martins R, Lithgow GJ, Link W. Long live FOXO: unraveling the role of FOXO proteins in aging and longevity. Aging Cell 2016;15:196-207. [Crossref] [PubMed]
- Daly DM, Nocchi L, Liaskos M, et al. Age-related changes in afferent pathways and urothelial function in the male mouse bladder. J Physiol 2014;592:537-49. [Crossref] [PubMed]
- Gregorini F, Knüpfer SC, Liechti MD, et al. Sensory evoked potentials of the bladder and urethra in middle-aged women: the effect of age. BJU Int 2015;115:18-25. [Crossref] [PubMed]
- He S, Sharpless NE. Senescence in Health and Disease. Cell 2017;169:1000-11. [Crossref] [PubMed]
- Acosta JC, Banito A, Wuestefeld T, et al. A complex secretory program orchestrated by the inflammasome controls paracrine senescence. Nat Cell Biol 2013;15:978-90. [Crossref] [PubMed]
- Ogrodnik M. Cellular aging beyond cellular senescence: Markers of senescence prior to cell cycle arrest in vitro and in vivo. Aging Cell 2021;20:e13338. [Crossref] [PubMed]
- Minciullo PL, Catalano A, Mandraffino G, et al. Inflammaging and Anti-Inflammaging: The Role of Cytokines in Extreme Longevity. Arch Immunol Ther Exp (Warsz) 2016;64:111-26. [Crossref] [PubMed]
- Lin Y, Nakachi K, Ito Y, et al. Variations in serum transforming growth factor-beta1 levels with gender, age and lifestyle factors of healthy Japanese adults. Dis Markers 2009;27:23-8. [Crossref] [PubMed]
- Sinha M, Jang YC, Oh J, et al. Restoring systemic GDF11 levels reverses age-related dysfunction in mouse skeletal muscle. Science 2014;344:649-52. [Crossref] [PubMed]
- Zhang YH, Cheng F, Du XT, et al. GDF11/BMP11 activates both smad1/5/8 and smad2/3 signals but shows no significant effect on proliferation and migration of human umbilical vein endothelial cells. Oncotarget 2016;7:12063-74. [Crossref] [PubMed]
- Zimmers TA, Jiang Y, Wang M, et al. Exogenous GDF11 induces cardiac and skeletal muscle dysfunction and wasting. Basic Res Cardiol 2017;112:48. [Crossref] [PubMed]
- Jeon YJ, Kim J, Cho JH, et al. Comparative Analysis of Human Mesenchymal Stem Cells Derived From Bone Marrow, Placenta, and Adipose Tissue as Sources of Cell Therapy. J Cell Biochem 2016;117:1112-25. [Crossref] [PubMed]
- Du WJ, Chi Y, Yang ZX, et al. Heterogeneity of proangiogenic features in mesenchymal stem cells derived from bone marrow, adipose tissue, umbilical cord, and placenta. Stem Cell Res Ther 2016;7:163. [Crossref] [PubMed]
- Spees JL, Lee RH, Gregory CA. Mechanisms of mesenchymal stem/stromal cell function. Stem Cell Res Ther 2016;7:125. [Crossref] [PubMed]
- Deng P, Yuan Q, Cheng Y, et al. Loss of KDM4B exacerbates bone-fat imbalance and mesenchymal stromal cell exhaustion in skeletal aging. Cell Stem Cell 2021;28:1057-1073.e7. [Crossref] [PubMed]
- Zheng H, Liang X, Han Q, et al. Hemin enhances the cardioprotective effects of mesenchymal stem cell-derived exosomes against infarction via amelioration of cardiomyocyte senescence. J Nanobiotechnology 2021;19:332. [Crossref] [PubMed]
- Zarei F, Abbaszadeh A. Application of Cell Therapy for Anti-Aging Facial Skin. Curr Stem Cell Res Ther 2019;14:244-8. [Crossref] [PubMed]
- Jin HJ, Bae YK, Kim M, et al. Comparative analysis of human mesenchymal stem cells from bone marrow, adipose tissue, and umbilical cord blood as sources of cell therapy. Int J Mol Sci 2013;14:17986-8001. [Crossref] [PubMed]
- Camões J, Coelho A, Castro-Diaz D, et al. Lower Urinary Tract Symptoms and Aging: The Impact of Chronic Bladder Ischemia on Overactive Bladder Syndrome. Urol Int 2015;95:373-9. [Crossref] [PubMed]
- Levy O, Kuai R, Siren EMJ, et al. Shattering barriers toward clinically meaningful MSC therapies. Sci Adv 2020;6:eaba6884. [Crossref] [PubMed]
- Infante A, Rodríguez CI. Cell and Cell-Free Therapies to Counteract Human Premature and Physiological Aging: MSCs Come to Light. J Pers Med 2021;11:1043. [Crossref] [PubMed]
- Yin Y, Chen H, Wang Y, et al. Roles of extracellular vesicles in the aging microenvironment and age-related diseases. J Extracell Vesicles 2021;10:e12154. [Crossref] [PubMed]
- Slijepcevic P. DNA damage response, telomere maintenance and ageing in light of the integrative model. Mech Ageing Dev 2008;129:11-6. [Crossref] [PubMed]