Investigation of the hemostatic mechanism of Gardeniae fructus Praeparatus based on pharmacological evaluation and network pharmacology
Introduction
Drinking alcohol has become common in everyday life, and the risks that are brought by excessive and long-term alcohol consumption have been widely studied in recent years. Alcohol consumption can either partially or entirely cause various illnesses, such as upper gastrointestinal bleeding, gastric ulcers, infectious diseases, alcohol-related liver disease, cancer, and diabetes (1-3). Further, a high concentration of ethanol—the type of alcohol that can be consumed—directly deteriorates epithelial cells in the gastric mucosa surface (4), resulting in hyperemia, edema, hemorrhage, erosive lesions, and acute inflammation of the gastric mucosa (5). Some reports show that the consumption of ethanol can cause acute gastrointestinal bleeding and lesions (6,7). The mechanisms of gastrointestinal damage caused by alcohol consumption are manifold. For one, ethanol accelerates gastric damage by recruiting immune cell infiltration, which triggers an inflammatory cascade (8). For another, alcohol reduces the barrier function of the gastric mucosa by stimulating the secretion of gastric acid and pepsin (9). In parallel, ethanol, as a strong gastric mucosal injury irritant, damages the mucous cells and the mucous layer, resulting in widespread and acute gastrointestinal bleeding and hemorrhagic gastritis (10,11). Moreover, the intragastric administration of ethanol is a widely accepted model for investigating upper gastrointestinal injury and bleeding (12).
According to the theory of traditional Chinese medicine (TCM), acute hemorrhage mostly results from blood heat (13). Therefore, relevant treatment focuses on cooling the blood and clearing heat to stop bleeding. Growing evidence indicates that Chinese herbal medicines can alleviate the progression of bleeding disorders based on hemostatic characteristics and advantages of multi-component, multi-target and multi-pathway (14). After being processed, some TCMs enhance the hemostatic effect. For example, Gardeniae fructus (GF) can purge fire, relieve restlessness, clear heating, drain dampness, cool blood, and detoxify; meanwhile, GF Praeparatus (GFP) can cool blood, halt bleeding (15), and treat hemoptysis, epistaxis, hemoptysis, and various internal hemorrhagic diseases (16). Several studies have examined the antihemorrhagic activity of GFP. Using a mouse tail transection model, researchers found that the bleeding time and coagulation time of mice treated with GFP were reduced by 43.71% and 32.81%, respectively (17). In addition, the 95% alcohol elution part of GFP could shorten the prothrombin time of normal rats (18). Some researchers argue that the changes in chemical constituents of GFP associated with hemostasis are vital for stopping the bleeding. For example, the contents of iridoid glycoside and crocin with antithrombotic effects are lower in GFP compared to GF (19,20), while the effective hemostatic components, such as tannin and crocetin, are higher in GFP than in GF (21,22), which contributes to the hemostatic effect of GFP. These pharmacodynamic experiments have demonstrated initial success in the procoagulant activity of GFP but have not examined the mechanism of action. Previous network pharmacology analysis of GF in Jiangxi Province indicates that the bioactive iridoids of GF are involved in hemostasis and other signaling pathways, which provides a valuable foundation for further research on the hemostatic activity of GFP (23). However, the antihemorrhagic mechanism of GFP remains largely unknown and can be further understood through network pharmacology.
Network pharmacology-based approaches differ from conventional pharmacological strategies: the former study the interactions between a single component and a single target in isolation, while network pharmacology-based approaches measure the regulatory effects of herbs, diseases, and biological systems with a systemic and holistic perspective that reflects a similarly holistic philosophy to that of TCM (24,25). Due to the in-depth integration and analysis of vast amounts of information in chemistry, biology, and medicine, findings based on network pharmacology are systematic, relevant, and predictable (26). The network pharmacology strategy is important for research in TCM for clarifying pharmacological actions and mechanisms of TCM against diseases.
The scheme of this research is presented in Figure 1. The quality assessment of GFP with ultra-performance liquid chromatography (UPLC) was completed first (Figure 1A). Then, a rat model of acute upper gastrointestinal bleeding induced by absolute ethanol was established. Those rats with gastric injury subsequently received different doses of GFP to observe the therapeutic effect, which provided the preliminary experimental basis for evaluating the hemostatic effect of GFP (Figure 1B). Following this, the network pharmacology approach was used to clarify the potential hemostatic mechanism of GFP and to identify the main bioactive components and core targets (Figure 1C). Additionally, the interactions between the critical protein and small-molecule ligand were validated in silico using molecular docking simulations (Figure 1D). Concerning the traditional efficacy of GFP on hemostasis, this study provides primary scientific evidence based on the pharmacological evaluation. The network pharmacology approach also provides clues to finding targets and pathways involved in the hemostasis of GFP, which, by extension, serves as the research basis for demonstrating the protective effect of GFP in gastrointestinal hemorrhage. We present the following article in accordance with the ARRIVE reporting checklist (available at https://atm.amegroups.com/article/view/10.21037/atm-21-6415/rc).
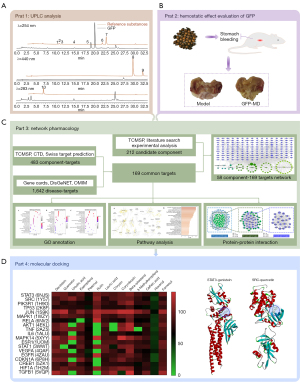
Methods
Experimental animals
Thirty healthy male Sprague-Dawley (SD) rats of specific pathogen-free (SPF) grade (weighing 230–250 g; 9 weeks old) were supplied by Beijing Vital River Laboratory Animal Technology Co., Ltd (Beijing, China). Rats were divided into 5 groups, with 6 rats in each, to objectivity and test for statistical significance. All rats were included in the study and maintained in a controllable environment with a constant temperature of 24±1 ℃, a humidity of 55%±10%, and a 12-h light-dark cycle. Before the establishment of the rat model, rats were adaptively fed with free access to water and diet for 3 days. Experiments were performed under a project license (No. 2021B008) granted by ethics board of China Academy of Chinese Medical Sciences, in compliance with the Laboratory Animal Care Center of China Academy of Chinese Medical Sciences institutional guidelines for the care and use of animals.
Materials
GF (lot No. 20200814) was purchased from Anguo city, Hebei Province, China, and identified by Prof. Cun Zhang as the desiccative ripe fruit of Gardenia jasminoides Ellis. GF was processed into GFP with black on the outside and brown on the inside by an experienced operator from Beijing BenCaoFangYuan Pharmaceutical Group Co., Ltd (Beijing, China). Ten reference substances were supplied by Chengdu Chroma-Biotechnology Co., Ltd (Chengdu, China): geniposidic acid, shanzhiside, deacetyl-asperulosidic acid methyl ester, gardenoside, scandosidemethyl ester, genipin-1-O-gentiobioside (G1), geniposide (G2), crocin-I (C-I), crocin-II (C-II), and 5-hydroxymethylfurfural (5-HMF). Enzyme-linked immunosorbent assay (ELISA) kits for the rat thromboxane B2 (TXB2) and 6-keto-prostaglandin-F1α (6-keto-PGF1α) were obtained from Jiancheng Bioengineering Institute (Nanjing, China). Absolute alcohol was of analytical grade and was provided by Sinopharm Chemical Reagent Co., Ltd (Shanghai, China).
Preparation of samples
Samples for UPLC analysis
First, 3 batches of GFP were processed based on the Chinese Pharmacopoeia 2020 Edition (lot No. 20201212). Crude GF was put into a frying machine at 260 ℃ and constantly stirred until the outer peel turned dark brown, charred points occasionally appeared in the endocarp, and the surface of the seed was tan in color. Subsequently, the samples for UPLC analysis were prepared. After GFP was pulverized to powder, a 0.50 g sample was extracted by ultrasound for 30 min with 50% methanol. After cooling to room temperature, the sample was adjusted to the original weight with 50% methanol. The solution was filtered through 0.22-µm nylon membrane filters for further analysis.
The mixed reference substances containing geniposidic acid (5.75 µg/mL), shanzhiside (64.00 µg/mL), deacetyl-asperulosidic acid methyl ester (15.90 µg/mL), gardenoside (31.80 µg/mL), scandosidemethyl ester (8.80 µg/mL), G1 (157.00 µg/mL), G2 (279.00 µg/mL), C-I (45.50 µg/mL), C-II (10.10 µg/mL), and 5-HMF (4.95 µg/mL) were dissolved with 50% methanol.
The decoction of GFP for animal experiments
A total of 900 g of GFP was soaked in 8 times its volume of water for 30 min and then boiled over high heat, which was followed by decoction for 30 min over low heat. After filtering the liquid medicine with gauze, the herb residue was decocted again, as completed in the previous steps. Medicinal liquid from the decoction was concentrated to a final volume of 500 mL with a concentration of 1.8 g/mL. This was diluted with hot water to 0.9 and 0.45 g/mL before use.
Determination of the major components of GFP
The contents of the main active components of the GFP decoction were determined by UPLC equipped with Waters Acquity UPLC BEH C18 column (2.1 mm × 100 mm; 1.7 µm), and the column temperature was maintained at 40 ℃. Chromatographic separation was performed according to a published report (27). Mobile phase A was 0.1% formic acid in the water, and mobile phase B was methanol. The gradient program for elution at a flow rate of 0.25 mL/min was as follows: 0–6 min, 94% eluent A; 6–11 min 94–86% eluent A; 11–19 min, 86–60% eluent A; 19–24 min, 60–55% eluent A; 24–29 min, 55–35% eluent A; 29–30 min, 35–0% eluent A; and 30–36 min, 0% eluent A. The injection volume of the sample was 1 µL. The detection was monitored at 254, 283 and 440 nm.
A animal model for gastrorrhagia and the dosing plan
A protocol was prepared before the study without registration. Thirty SD male rats were randomly allocated using the standard “= RAND()” function in Microsoft Excel, which is shown in the supplementary table at https://cdn.amegroups.cn/static/public/atm-21-6415-1.xlsx. There were 5 groups, with 6 rats in each group: the normal group, model group, GFP low-dose group (GFP-LD, 4.5 g/kg), GFP medium-dose group (GFP-MD, 9 g/kg), and GFP high-dose group (GFP-HD, 18 g/kg). The drugs were prepared by Y Zheng, and then rats were given corresponding drugs by Y Gao. The normal and model group rats were administered isotonic saline by oral gavage, while the GFP group rats were intragastrically administered an amount of 10 mL/kg. Two hours after administration, all rats, apart from rats in the normal group, received absolute ethanol at 5 mL/kg to establish a rat model of acute gastrointestinal hemorrhage. In the next hour, all rats were given the same drugs as those in the first administration. One hour after the second administration, the rats in each group were anesthetized with 10% chloral hydrate, dissected for blood samples from the abdominal aorta, and collected their gastric tissues.
Direct observation and histological evaluation of gastric tissues
The stomach tissues were taken for photographic observation to intuitively present the hemorrhage regions. After that, samples of the stomach were immersion-fixed in neutral buffered formalin for 48 h, which was followed by stepwise treatment with ethanol, xylene, and paraffin. Next, thick sections were stained with hematoxylin and eosin for light microscopy.
The levels of hemostasis-associated factors by ELISA
ELISA was used to determine the levels of 6-keto-PGF1α and TXB2 in the serum of rats.
Statistical analysis
Data were analyzed by L Zhang. All data are expressed as mean ± SD. GraphPad Prism 7 (GraphPad, San Diego, CA, USA) software was applied to analyze the significant differences among 3 or more groups with a 1-way analysis of variance (ANOVA). Pairwise comparisons were performed with the least significant difference test. All tests were 2-sided, and a P value <0.05 was considered statistically significant.
Network pharmacology analysis
Through network pharmacology, the components of TCM can be comprehensively mined, the drugs and disease targets can subsequently be selected from a large number of databases, and their complex relationships can be visualized. After this, protein-protein interaction (PPI), functional annotations, and pathway analysis can be used to systematically and comprehensively examine the mechanism of drug intervention in the disease networks. Here, Y Zheng carried out network pharmacology analyses to illuminate the potential mechanisms of GFP on gastrointestinal bleeding.
Screening of active components from Gardenia jasminoides
A comprehensive and accurate collection of active ingredients from GF is the first consideration concern. Here, compounds of GF were extracted from the TCM systems pharmacology database and analysis platform (TCMSP; https://tcmsp-e.com/) (28), a comprehensive database of Chinese herbal medicine. Moreover, the active compounds from GF in published literature and 10 compounds identified in this study were supplemented.
Target prediction of candidate components
The targets associated with these components were obtained by target fishing from the TCMSP, Search Tool for Interacting Chemicals (STITCH; http://stitch.embl.de/) (29), and Swiss TargetPrediction (http://www.swisstargetprediction.ch/) databases (30). In the present study, the more credible targets were selected based on compound-protein interactions with a score of more than 0.9 in STITCH (31) and a probability higher than 0.6 in Swiss TargetPrediction (32). After removing duplication and merging, compound-related protein targets were standardized to official gene symbols in the Uniprot protein database (https://www.uniprot.org/) (33).
Collection of disease targets
The known disease-related targets were retrieved from the Online Mendelian Inheritance in Man (OMIM; http://omim.org/) (34), GeneCards (https://www.genecards.org/) (35), and a database of gene-disease associations (DisGeNet; https://www.disgenet.org/) (36) databases using keywords of “gastrointestinal bleeding” and “gastrointestinal hemorrhage”. A higher score indicated a stronger interaction, so the targets with a relevance score >5 in GeneCards were collected (37). All target names were converted into gene symbols using UniProt databases, with the species limited to “Homo sapiens.” We gathered the genes of the active components from Gardenia jasminoides and gastrointestinal hemorrhage-related targets to determine the overlapping target genes using a Venn diagram.
Compound-target network construction
To visualize and analyze the various relationships between components and potential therapeutic targets, the complicated interactions through which active ingredients connected with their corresponding targets were visualized using Cytoscape software (version 3.6.1; http://cytoscape.org/) (38). Nodes in the network represented compounds or targets while the edges connecting nodes indicated their interactions.
Gene Ontology (GO) and pathway analysis
The screened targets were imported into the Metascape (http://metascape.org/) (39) platform for GO enrichment analysis, including biological processes, cellular components, and molecular functionals. The results of the GO category were visualized using an online platform (http://www.bioinformatics.com.cn/). Pathway analysis of the overlapping genes was performed using the Reactome (https://www.reactome.org/) (40), a relational database of signaling and metabolic molecules and their relations organized into biological pathways and processes.
PPI analysis
We first focused on the biological implications of PPI networks of the potential target genes. These related therapeutic targets of GFP for treating gastrointestinal hemorrhage were uploaded to the Metascape database to establish a PPI network. Some regions of high density in the complex PPI networks were potential subnets and were considered collections with biological significance. Therefore, a densely connected protein complex was identified based on the molecular complex detection (MCODE) algorithm, and the functional description of each MCODE component was summarized.
Next, the major hubs were screened using topological analysis. First, the STRING online tool was applied to construct a PPI network by identifying the highest confidence scores, which were greater than 0.9. The results were subsequently imported into Cytoscape for visualization. The network topological properties were calculated using NetworkAnalyzer, including the degree, betweenness centrality (BC), and closeness centrality (CC), to estimate the importance of nodes. According to the principle that the values of three parameters are greater than the median, the hub genes were extracted after two analyses.
Molecular docking
To clarify how active ingredients act on hub genes, we conducted molecular docking using Discovery Studio 2016 (Biovia Inc., Scottsdale, AZ, USA). The active ingredients were prepared through the PubChem database and Open Babel software and stored in PDB format, while the suitable crystal structures of these proteins were collected from the Protein Data Bank (RCSB PDB; https://www.rcsb.org/). Before docking, crystallographic water molecules were removed, followed by selection of the “Prepare Protein” module to modulate the polyconformation of the protein and its incomplete amino acid residues. Importantly, “LibDock” was used for the docking calculation. The LibDock score showed the affinity between the protein and the molecule, with higher numbers indicating higher affinity.
Results
Quantitative analysis of GFP samples
The quality of GFP was assessed using UPLC to accurately measure the major active constituents. The chromatograms of ten mixed reference substance solutions and GFP samples are shown in Figure 2A. The seven iridoids (geniposidic acid, shanzhiside, deacetyl-asperulosidic acid methyl ester, gardenoside, scandosidemethyl ester, G1, and G2) were distinctly detected at 254 nm, while crocins (C-I and C-II) were detected at 440 nm and 5-HMF at 283 nm. The contents of these components are shown in Figure 2B. According to the 2020 edition of the Chinese Pharmacopoeia, G2 was the chemical marker for the quality control of GFP. The content of G2 (6.3%) was consistent with the criterion of Chinese pharmacopoeia (not less than 1.0 percent), which indicated a good quality of GFP in this study.
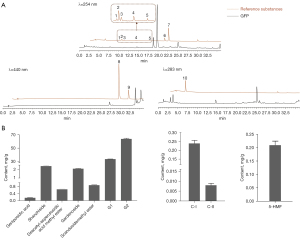
Efficacy evaluation of the protective effect of GFP on gastrointestinal bleeding
Macroscopic evaluation of gastric lesions
There were no adverse events during the medication. The gastric sections of the rats can be found in Figure 3A, which shows the intact architecture and a smooth surface of the gastric mucosa in the normal group of rats. In contrast, gastric tissues from the model group displayed severe gastric injuries triggered by absolute ethanol, including cordlike hemorrhagic injury and numerous scattered hemorrhagic spots. Treatment with a low dose of GFP slightly improved the ethanol-induced injury compared with the model group. Particularly, the medium- and high-GFP doses were found to ameliorate the gastric mucosal injury despite leaving behind slight redness and swelling, indicating their gastroprotective effect.
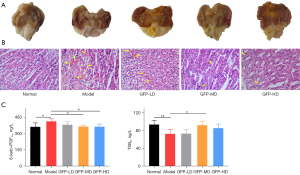
Histological analysis of gastric lesions
The histological sections further demonstrated the curative effect on gastric hemorrhage of GFP in different doses (Figure 3B). The gastric tissues of healthy normal rats showed complete gastric mucosa and neatly arranged glands. In contrast, there were apparent pathological changes in the stomach of the model group characterized by a large hemorrhagic area, severe edema, and abundant inflammatory cell infiltration. GFP ameliorated the histopathological manifestations of gastrorrhagia to varying degrees. The severity of bleeding of the gastric mucosa in the GFP-LD group was lighter than that of the model group. Further, an extremely low number of bleeding spots and little inflammatory cell infiltration were observed in the GFP-MD and GFP-HD groups.
Influence on hemostasis-related factors
To investigate how the hemostasis-related factors change during gastric bleeding, we measured the levels of 6-keto-PGF1α and TXB2 in the serum of rats using an ELISA assay (Figure 3C). The model group had a significantly higher content of 6-keto-PGF1α and lower levels of TXB2, coupled with severe gastric bleeding, than did those of the normal group (P<0.05; P<0.01). GFP-LD showed weak regulation on these 2 factors, but the difference was not statistically significant. The level of 6-keto-PGF1α declined in a dose-dependent manner, and it was lower when the medium and high doses were used compared with the model group. The content of TXB2 in the serum of rats in the GFP-HD group and GFP-LD group exhibited a nonsignificant increase. Accordingly, ethanol-induced gastric bleeding was ameliorated to varying degrees after treatment with different doses of GFP. Both GFP-MD and GFP-LD affected the active hemostatic factors, such as by promoting platelets to release thromboxane and inhibiting vascular endothelial cells to release prostacyclin, which enabled coagulation.
Network pharmacology-based investigations
Collection of active ingredients from GFP
GFP was found to contain abundant natural ingredients, such as iridoids, flavonoids, and organic acids. Combined with the retrieval results from TCMSP, content determination, and literature research, the chemical composition database of GFP was established. A total of 212 active ingredients are presented in the supplementary table at https://cdn.amegroups.cn/static/public/atm-21-6415-2.xlsx in the form of compound type classification.
Targets prediction of compounds and disease
To predict the target genes of these compounds thoroughly, we mined multiple databases, and 337, 110, and 128 targets were collected from TCMSP, STITCH, and SwissTargetPrediction, respectively. As shown in the supplementary table at https://cdn.amegroups.cn/static/public/atm-21-6415-3.xlsx, 483 targets that associated with compounds were retained after removing duplicates. Using “gastrointestinal bleeding” and “gastrointestinal hemorrhage” as keywords, 1,642 pathogenic-target genes were found through mining the GeneCards, DisGeNET, and OMIM databases (the supplementary table at https://cdn.amegroups.cn/static/public/atm-21-6415-4.xlsx). Ultimately, 169 potential gene targets related to the protective effect of GFP for gastrointestinal bleeding were obtained by intersecting the above results and were used as the focus for subsequent analysis (the supplementary table at https://cdn.amegroups.cn/static/public/atm-21-6415-5.xlsx).
Network construction of compounds and targets
We determined that 58 components acted on 169 potential targets, including iridoid glycosides, monoterpenoids, triterpenes, yellow pigments, flavonoids, phenolic acids and organic acid derivatives, sesquiterpenoids, coumarins, and other compounds. The component-target network of GFP in treating gastric bleeding was constructed to visually demonstrate the relationship between active ingredients and target genes (Figure 4). In this image, light purple nodes represent potential targets, circular nodes represent the compound, and lines represent interactions between them; the larger the nodes are in the network, the greater their influence on healing gastrointestinal hemorrhage. Compound F6, quercetin, was a noteworthy component because of numerous associations with multiple targets.
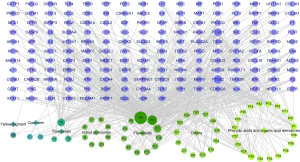
GO and pathway analysis
To gain a biological perspective on the 169 candidate targets, GO term enrichment analysis including biological processes, cellular components, and molecular functions was conducted using Metascape. The top 20 GO enriched terms are illustrated in Figure 5. Notably, the response to wounding and wound healing dominated the biological process, which strongly demonstrated the association with the pathogenesis of gastrointestinal hemorrhage. In terms of cellular components, most of the gene targets were located on the cellular membrane, vesicle lumen, and platelet alpha granule. Signaling receptor activator activity, receptor-ligand activity, and cytokine receptor binding were the major molecular functions of the targets on a large scale.
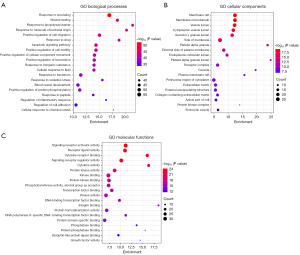
To further characterize signal pathways throughout which the predicted genes were distributed, these genes were subjected to Reactome pathway analysis. Each cluster corresponded to the network of biological functions. In Figure 6A, the enrichment pathways are highlighted in yellow in the networks; indicating that a large number of targets are gathered in signal transduction, immune system, gene expression (transcription), hemostasis, and other biological function networks. An analysis of the pathways provided the top 25 results in descending order by P value (shown in Figure 6B), which included interleukin 4 and interleukin 13 signaling, signaling by interleukins, cytokine signaling in the immune system, signaling by receptor tyrosine kinases, platelet activation, hemostasis, and others.
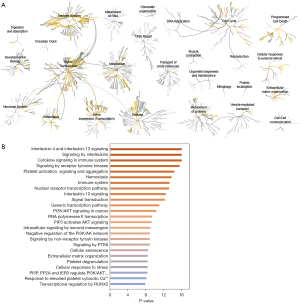
PPI network of gastrointestinal hemorrhage
Analysis of the biological significance of the PPI network
Therapeutic targets related to a protective effect of GFP for gastrointestinal bleeding were imported into the Metascape database to analyze PPI, as shown in Figure 7A. To gain insight into the biological significance of PPI networks, the densely connected subnetworks were identified based on the MCODE algorithm. The MCODE networks that resulted from topological and functional module identification were considered biologically significant (41). Figure 7B shows the 6 main subnetworks. Biological annotation was applied to each MCODE network independently, and the 3 terms with best-scoring P values from high to low were retained as the functional description of the corresponding subnetworks, as depicted in Table 1. These networks revealed biological functions, such as regulation of the cell cycle process and response to wounding and xenobiotic stimuli.
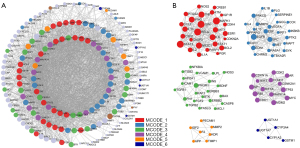
Table 1
Color | MCODE | GO | Description | Log10(P) |
---|---|---|---|---|
![]() |
MCODE_1 | GO:0071396 | Cellular response to lipid | −22 |
![]() |
MCODE_1 | GO:0032496 | Response to lipopolysaccharide | −19.7 |
![]() |
MCODE_1 | GO:0002237 | Response to molecule of bacterial origin | −19.3 |
![]() |
MCODE_2 | GO:0001934 | Positive regulation of protein phosphorylation | −19.2 |
![]() |
MCODE_2 | GO:0043549 | Regulation of kinase activity | −17.2 |
![]() |
MCODE_2 | GO:0033674 | Positive regulation of kinase activity | −16.4 |
![]() |
MCODE_3 | GO:0097190 | Apoptosis signaling pathway | −18.2 |
![]() |
MCODE_3 | GO:0097191 | Extrinsic apoptosis signaling pathway | −17.5 |
![]() |
MCODE_3 | GO:0009611 | Response to wounding | −13.1 |
![]() |
MCODE_4 | GO:1901987 | Regulation of cell cycle phase transition | −13.5 |
![]() |
MCODE_4 | GO:0010564 | Regulation of cell cycle process | −13.4 |
![]() |
MCODE_4 | GO:0000077 | DNA damage checkpoint signaling | −13.3 |
![]() |
MCODE_5 | GO:0001934 | Positive regulation of protein phosphorylation | −6.6 |
![]() |
MCODE_5 | GO:0018108 | Peptidyl-tyrosine phosphorylation | −6 |
![]() |
MCODE_5 | GO:0018212 | Peptidyl-tyrosine modification | −6 |
![]() |
MCODE_6 | GO:0006805 | Aerobiotic metabolic process | −12.3 |
![]() |
MCODE_6 | GO:0071466 | Cellular response to xenobiotic stimulus | −12.2 |
![]() |
MCODE_6 | GO:0009410 | Response to xenobiotic stimulus | −12.1 |
MCODE, molecular complex detection; GO, Gene Ontology.
Identification of hub targets from the PPI network
To further clarify the hub targets, 169 potential targets were analyzed using the STRING database. We created a PPI network incorporating 153 nodes and 860 interactions was after setting the confidence to larger than 0.9 and hiding the disconnected nodes. This was followed by analyzing the network in Cytoscape software. The median values of the three parameters (degree, BC, and CC) were considered as the screening criteria, so 22 hub targets in the ultimate network diagram were identified after double-screening (Figure 8; Table 2). The top 25 pathways they were involved in are shown in Ultimately, 169 potential gene targets related to the protective effect of GFP for gastrointestinal bleeding were obtained by intersecting the above results and were used as the focus for subsequent analysis (the supplementary table at https://cdn.amegroups.cn/static/public/atm-21-6415-6.xlsx. After the exclusion of EDN1, CAV1, and PTK2 due their being less involved in the pathways associated with hemostasis, the remaining 19 targets were analyzed further. By matching and mapping between components and targets, 14 major components acting on these core targets were acquired. Their relevant information is shown in Table 3.
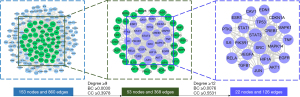
Table 2
Target | Description | UniProt | Degree | BC | CC |
---|---|---|---|---|---|
STAT3 | Signal transducer and activator of transcription 3 | P40763 | 52 | 0.115 | 0.545 |
SRC | Proto-oncogene tyrosine-protein kinase Src | P12931 | 45 | 0.097 | 0.538 |
PIK3R1 | Phosphatidylinositol 3-kinase regulatory subunit alpha | P27986 | 44 | 0.067 | 0.523 |
TP53 | Cellular tumor antigen p53 | P04637 | 43 | 0.109 | 0.512 |
JUN | Transcription factor AP-1 | P05412 | 41 | 0.070 | 0.517 |
MAPK1 | Mitogen-activated protein kinase 1 | P28482 | 39 | 0.064 | 0.510 |
RELA | Transcription factor p65 | Q04206 | 39 | 0.057 | 0.512 |
AKT1 | RAC-alpha serine/threonine-protein kinase | P31749 | 34 | 0.057 | 0.493 |
TNF | Tumor necrosis factor | P01375 | 32 | 0.027 | 0.472 |
IL6 | Interleukin-6 | P05231 | 30 | 0.027 | 0.473 |
MAPK14 | Mitogen-activated protein kinase 14 | Q16539 | 28 | 0.019 | 0.489 |
ESR1 | Estrogen receptor | P03372 | 25 | 0.013 | 0.469 |
STAT1 | Signal transducer and activator of transcription 1-alpha/beta | P42224 | 23 | 0.014 | 0.463 |
VEGFA | Vascular endothelial growth factor A | P15692 | 23 | 0.011 | 0.459 |
EGFR | Epidermal growth factor receptor | P00533 | 22 | 0.014 | 0.464 |
CDKN1A | Cyclin-dependent kinase inhibitor 1 | P38936 | 22 | 0.011 | 0.462 |
CREB1 | Cyclic AMP-responsive element-binding protein 1 | P16220 | 19 | 0.007 | 0.459 |
HIF1A | Hypoxia-inducible factor 1-alpha | Q16665 | 19 | 0.005 | 0.457 |
TGFB1 | Transforming growth factor beta-1 proprotein | P01137 | 15 | 0.010 | 0.449 |
GFP, Gardeniae fructus Praeparatus; BC, betweenness centrality; CC, closeness centrality.
Table 3
No. | Compound | Formula | MW | OB (%) | DL | Count | Target |
---|---|---|---|---|---|---|---|
F5 | Genistein | C15H10O5 | 270.24 | 17.93 | 0.21 | 15 | AKT1, CDKN1A, EGFR, ESR1, HIF1A, IL6, MAPK1, MAPK14, RELA, STAT1, STAT3, TGFB1, TNF, TP53, VEGFA |
F6 | Quercetin | C15H10O7 | 302.25 | 46.43 | 0.28 | 15 | AKT1, CDKN1A, EGFR, HIF1A, IL6, JUN, MAPK1, PIK3R1, RELA, SRC, STAT1, TGFB1, TNF, TP53, VEGFA |
T2 | Ursolic acid | C30H48O3 | 456.78 | 16.77 | 0.75 | 8 | CDKN1A, CREB1, IL6, RELA, STAT3, TNF, TP53, VEGFA |
F7 | Kaempferol | C15H10O6 | 286.25 | 41.88 | 0.24 | 4 | AKT1, RELA, STAT1, TNF |
P12 | Paeonol | C9H10O3 | 166.19 | 28.79 | 0.04 | 4 | AKT1, MAPK1, RELA, TNF |
F1 | Rutin | C27H30O16 | 610.57 | 3.2 | 0.68 | 3 | IL6, RELA, TNF |
P42 | Lauric acid | C12H24O2 | 200.36 | 23.59 | 0.04 | 3 | AKT1, IL6, RELA |
F10 | Chrysin | C15H10O4 | 254.25 | 22.61 | 0.18 | 2 | CDKN1A, TGFB1 |
F11 | Corymbosin | C19H18O7 | 358.37 | 51.96 | 0.41 | 2 | ESR1, MAPK14 |
T9 | Beta-sitosterol | C29H50O | 414.79 | 36.91 | 0.75 | 1 | TGFB1 |
F8 | 3-Methylkempferol | C16H12O6 | 300.28 | 60.16 | 0.26 | 1 | MAPK14 |
P1 | Caffeic acid | C9H8O4 | 180.17 | 25.76 | 0.05 | 1 | TNF |
O6 | Hexanal | C6H12O | 100.18 | 55.71 | 0.01 | 1 | TNF |
O12 | Farnesol | C15H26O | 222.41 | 28.44 | 0.06 | 1 | IL6 |
MW, molecular weight; OB, oral bioavailability; DL, drug-likeness.
Molecular docking analysis
Binding activities between the 19 core targets and 14 active components were verified after molecular docking simulations. The results of the validation were analyzed using a heat map (Figure 9A). Genistein, quercetin, kaempferol, lauric acid, corymbosin, beta-sitosterol, 3-methylkempferol, caffeic acid, and farnesol appeared to demonstrate a better binding activity with most core targets, indicating that these compounds might play a crucial role in a protective effect for gastrointestinal bleeding. Notably, genistein and quercetin acted on most of the targets; representative schematic interactions in which they made contact with STAT3 and Proto-oncogene tyrosine-protein kinase Src (SRC) are shown in Figure 9B. STAT3-genistein binding and SRC-quercetin binding were mostly in the form of hydrogen bonds and Pi bonds, which exhibited reliable binding activity.
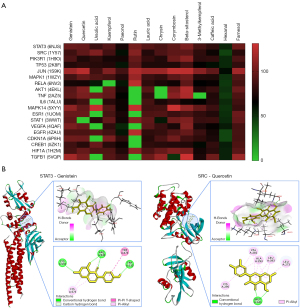
Discussion
GFP, a processed product of GF, contains multiple critical ingredients. First, we evaluated the quality of GFP with a simple and rapid UPLC method for determining the main components. Particularly, iridoids and crocins are well recognized components in GFP and are considered effective indicators for quality assessment (42). The UPLC analysis method achieved simultaneous and accurate quantitation for 7 iridoids, 2 crocins, and 5-HMF, among which the content of geniposide exactly complied with the provision shown in the Pharmacopoeia. Overall, this study provided a preliminary evaluation of the internal quality of GFP based on the simultaneous quantification of multiple compounds.
After being processed, GFP has a positive hemostatic effect as mentioned in the Pharmacopoeia. In our previous research, the therapeutic effect of different processed products of GFP on rats with gastric bleeding injury was verified with a dose of 4.5 g/kg (5 times higher than that of clinical dosage). However, the obtained results suggested that GFP had little effect on hemostasis, probably because of the low exposure. In addition, Hu (17) found that GFP had a good hemostatic effect at a dosage of 15.5 times higher than that of the clinical dosage when using mice as the research object. In this study, 4.5 g/kg was used as the initial dose, and the medium-dose and high-dose groups were given doses of 9 and 18 g/kg (at the dose of 10 and 20 times of clinical dose), respectively, to explore the hemostatic dose of GFP. Ethanol-induced gastric injury in experimental animals is considered a common research model for gastric lesions (43), which is why we used it to investigate the hemostatic effect of GFP in this study. Findings related to the levels of hemostatic biomarkers, direct observation, and histologic analysis of stomach tissues showed that hemorrhagic gastric lesions had a better rate of remission after treatment with a medium dose of GFP than those treated with a high dose. We speculated that high concentrations of GFP have some potential toxicity that hinders its protective ability for gastric hemorrhage; thus, a medium dose of GFP is more effective than is a high dosage in protecting against gastric bleeding.
In the case of a hemorrhage, the body rapidly initiates hemostasis-related reactions, affecting the release or expression of regulatory substances. For example, TXA2 could be secreted by activated platelets while causing positive feedback amplification, ensuring the immediate activation and recruitment of platelets into damaged areas to form platelet thrombus, which was the earliest reaction in the hemostatic process (44). In contrast, prostaglandin I2 (PGI2) is known to dilate blood vessels and inhibit platelet adhesion and aggregation (45). In addition to PGI2 and TXA2, other types of prostaglandins can produce platelet inhibition and influence vascular tone, including PGE1, PGE2, PGD2, and PGF2α (46). The order of potency of platelet activation inhibitors is as follows: PGI2 > 6-keto-PGE1 (the metabolite of PGE1) > PGD2 > PGE2 > PGF2α (47). Specifically, the antiplatelet aggregation activity of PGI2 has been reported to be 30 times higher than that of PGE1 and 10 times higher than that of PGD2. Meanwhile, the antiaggregatory actions of PGD2 on platelets of rats are difficult to verify because PGD2 inhibits platelet aggregation in rat plasma to a weaker extent than it does in human plasma (48). PGF2α is involved in various diseases and predominantly regulates reproductive functions of the female body (49). Given this, PGI2 was selected as a presentative prostaglandin with negative regulating blood coagulation to explore its activity. Taking into account the instability problem of PGI2 and TXA2, their stable metabolites 6-keto-PGF1α and TXB2 are often considered indicators to reflect their levels (50). As expected, the dynamic changes of 6-keto-PGF1α and TXB2 indicated the regulatory effects of different doses of GFP on the pathological conditions of bleeding, among which the moderating effects of GFP-MD and GFP-HD were quite apparent, showing ideal hemostatic efficacy. In addition, recent research on the GF regulation of prostaglandins suggests that GF can downregulate the expression of PGE2 (51,52). Compared to high concentrations of PGE2 inhibiting platelet aggregation, a low concentration of PGE2 can promote platelet aggregation and facilitate the hemostatic effect (53). Overall, these studies indicate the importance of prostaglandin in the hemostatic effect of GFP.
Network pharmacology-based strategies can help to decipher the underlying hemostatic mechanism of GFP. First, we screened 58 components of GFP and 169 targets to construct a network diagram that showed the interactions between multiple proteins and their corresponding components. Further, 169 candidate targets were analyzed by GO and Reactome enrichment analyses. Response to wounding, wound healing, and response to lipopolysaccharide were highly represented based on the analysis of the biological processes associated with antihemorrhagic development. Recent research shows that lipopolysaccharide can trigger platelet activation (54). Using pathway enrichment analysis, we found that GFP effectively exerted a protective effect against gastric bleeding through multiple pathways, influencing signaling by interleukins, cytokine signaling in the immune system, signaling by receptor tyrosine kinases, platelet activation, and others. It is widely acknowledged that the inflammatory mechanism is closely correlated with hemorrhage and with the regulation of several interleukins (55,56). In particular, interleukin 4 and interleukin 13 can induce downstream anti-inflammatory responses and result in hematoma and healing (57). Wound repair is associated with hemostasis, and cytokine signaling is one of the well-known pathways for wound healing (58). In addition, receptor tyrosine kinases stimulate hemostasis by promoting platelet stabilization and modulating inflammation (56).
A PPI network can be used to identify relationships at the molecular level by identifying subnetworks with biological significance (59). There were 6 main subnetworks decomposed out from the whole PPI network. The MCODE_1 network showed that biological functions concentrated on the cellular response to lipids and lipopolysaccharide. The MCODE_2 network revealed positive protein phosphorylation and kinase activity regulation, which had important effects on the pathogenesis of hemorrhage. Response to wounding was mentioned again in the MCODE_3 network. More importantly, the topological attributes of PPI networks were analyzed to highlight the key core targets and the corresponding main components. After 2 screenings, STAT3 was confirmed as one of the hub genes related to antihemorrhagic effects. Studies report that STAT3, a nuclear transcription factor activated by cytokine-induced intracellular signals has a vital role in platelet function and that STAT3 signaling can lead to platelet activation and aggregation (60). Specifically, STAT3 participates in the expression of thrombopoietin, ultimately promoting platelet production (61). SRC was the first proto-oncogene to be found; it has a central role in platelets and affected proplatelet formation (62). Clinical cases suggest that diseases arising from SRC mutation could present as thrombocytopenia (63). Beyond that, reports show some disorders of Mitogen-activated protein kinases (MAPKs) in gastric lesions. Thus, inhibition of either MAPK would relieve ethanol-induced gastric damage (64). First, ethanol significantly triggers hemorrhagic gastric mucosal injury, which can augment inflammatory mediators, such as tumor necrosis factor alpha (TNF-α) and interleukin 6 (65). The molecular docking results demonstrated that genistein and quercetin had a strong binding ability to STAT3 and SRC proteins, and could exert a hemostatic effect by regulating these relevant targets. Existing literature shows that genistein not only enhances antioxidant capacity by improving the redox environment of damaged tissues to accelerate wound healing but also regulates the expression of coagulation and fibrinolytic genes in human liver cell lines and plays a role in the hemostatic system (66,67). Reports also show that quercetin is an important hemostatic factor that promotes blood coagulation by decreasing activated partial prothrombin kinase time and increasing fibrin in rats (68). Finally, we summarized some representative pathways that GFP might act on to achieve the desired coagulation effect (Figure 10). Based on this evidence, it is increasingly clear that GFP might exert protective effects against gastrointestinal hemorrhage via multiple components acting on these hub genes.
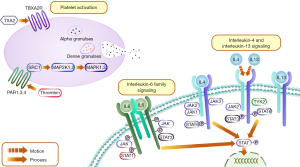
A network pharmacology strategy can qualitatively predict targets and pathways that drugs affect, but its reliability and related pharmacological mechanisms need to be verified through animal experiments or even clinical trials. Therefore, similar problems also appeared in this study. Further research on the mechanisms connected to the antihemorrhagic effect of GFP is necessary. For example, results from network pharmacology analysis could be pursued further through Western blots to verify that STAT3, SRC, and MAPK1 are indeed key targets of GFP in the treatment of bleeding. In addition, platelet aggregation and activation could be examined experimentally. Meanwhile, proteomics—a promising way to decipher the mechanisms from the molecular level—could be applied to investigate the expression levels of proteins that regulate platelet aggregation and activation.
Conclusions
Collectively, GFP played a protective effect in ethanol-induced gastrointestinal hemorrhage in rats, and the optimal dosage of 9 g/kg achieved the desired effect. We predicted that hemostatic targets regulated by GFP engaged in the biological process of response to wounding and wound healing. The main pathways involving cytokine signaling in an immune system and platelet activation might have an important role in healing bleeding. These findings remain to be further confirmed in future research.
Acknowledgments
Funding: This work was supported by the scientific and technological innovation project of China Academy of Chinese Medical Sciences (No. CI2021A04204); the National Natural Science Foundation of China (Nos. 81873010, 82173979, 81703708); and the Fundamental Research Funds for the Central public welfare research institutes of China Academy of Chinese Medical Sciences (Nos. zz13-019, zz13-YQ-050).
Footnote
Reporting Checklist: The authors have completed the ARRIVE reporting checklist. Available at https://atm.amegroups.com/article/view/10.21037/atm-21-6415/rc
Data Sharing Statement: Available at https://atm.amegroups.com/article/view/10.21037/atm-21-6415/dss
Conflicts of Interest: All authors have completed the ICMJE uniform disclosure form (available at https://atm.amegroups.com/article/view/10.21037/atm-21-6415/coif). The authors have no conflicts of interest to declare.
Ethical Statement: The authors are accountable for all aspects of the work in ensuring that questions related to the accuracy or integrity of any part of the work are appropriately investigated and resolved. Experiments were performed under a project license (No. 2021B008) granted by ethics board of China Academy of Chinese Medical Sciences, in compliance with the Laboratory Animal Care Center of China Academy of Chinese Medical Sciences institutional guidelines for the care and use of animals.
Open Access Statement: This is an Open Access article distributed in accordance with the Creative Commons Attribution-NonCommercial-NoDerivs 4.0 International License (CC BY-NC-ND 4.0), which permits the non-commercial replication and distribution of the article with the strict proviso that no changes or edits are made and the original work is properly cited (including links to both the formal publication through the relevant DOI and the license). See: https://creativecommons.org/licenses/by-nc-nd/4.0/.
References
- Akanda MR, Kim IS, Ahn D, et al. Anti-Inflammatory and Gastroprotective Roles of Rabdosia inflexa through Downregulation of Pro-Inflammatory Cytokines and MAPK/NF-κB Signaling Pathways. Int J Mol Sci 2018;19:584. [Crossref] [PubMed]
- Chi X, Pan CQ, Liu S, et al. Regulating Intestinal Microbiota in the Prevention and Treatment of Alcohol-Related Liver Disease. Can J Gastroenterol Hepatol 2020;2020:6629196. [Crossref] [PubMed]
- Kelly JP, Kaufman DW, Koff RS, et al. Alcohol consumption and the risk of major upper gastrointestinal bleeding. Am J Gastroenterol 1995;90:1058-64. [PubMed]
- Song SH, Kim JE, Sung JE, et al. Anti-ulcer effect of Gallarhois extract with anti-oxidant activity in an ICR model of ethanol/hydrochloride acid-induced gastric injury. J Tradit Complement Med 2019;9:372-82. [Crossref] [PubMed]
- Zhang J, Fu ZL, Chu ZX, et al. Gastroprotective Activity of the Total Flavones from Abelmoschus manihot (L.) Medic Flowers. Evid Based Complement Alternat Med 2020;2020:6584945. [Crossref] [PubMed]
- Jung MK, Callaci JJ, Lauing KL, et al. Alcohol exposure and mechanisms of tissue injury and repair. Alcohol Clin Exp Res 2011;35:392-9. [Crossref] [PubMed]
- Goso Y, Ueno M, Hotta K, et al. Protective effects of the whisky congeners on ethanol-induced gastric mucosal damage. Alcohol Clin Exp Res 2007;31:390-4. [Crossref] [PubMed]
- Shin JK, Park JH, Kim KS, et al. Antiulcer Activity of Steamed Ginger Extract against Ethanol/HCl-Induced Gastric Mucosal Injury in Rats. Molecules 2020;25:4663. [Crossref] [PubMed]
- Chang W, Bai J, Tian S, et al. Autophagy protects gastric mucosal epithelial cells from ethanol-induced oxidative damage via mTOR signaling pathway. Exp Biol Med (Maywood) 2017;242:1025-33. [Crossref] [PubMed]
- Czekaj R, Majka J, Magierowska K, et al. Mechanisms of curcumin-induced gastroprotection against ethanol-induced gastric mucosal lesions. J Gastroenterol 2018;53:618-30. [Crossref] [PubMed]
- Franke A, Singer MV. Effects of Beer on the Gastric Mucosa as Determined by Endoscopy - ScienceDirect. Beer in Health and Disease Prevention 2009:577-80.
- Kwiecien S, Jasnos K, Magierowski M, et al. Lipid peroxidation, reactive oxygen species and antioxidative factors in the pathogenesis of gastric mucosal lesions and mechanism of protection against oxidative stress - induced gastric injury. J Physiol Pharmacol 2014;65:613-22. [PubMed]
- Chu Y, Zhang L, Zhu X, et al. Investigation of hemostatic effect of spleen-invigorating, qi-replenishing and blood-arresting formula on simvastatin-induced zebrafish hemorrhage model. Journal of Traditional Chinese Medical Sciences 2016;3:226-33. [Crossref]
- He X, Fan P, Li M, et al. Advances in study of traditional Chinese hemostyptic drugs and related mechanisms. Chinese Journal of Experimental Traditional Medical Formulae 2010;16:217-21.
- Commission CP. Pharmacopeia of the People’s Republic of China, Volume 1. Chinese Pharmacopoeia Commission ed. Beijing: Chemical and Industrial Publisher, 2020.
- Ma L. A brief discussion on the pharmacological action of Gardeniae Fructus Praeparatus and its therapeutic effect on blood syndrome. Contemporary Medical Symposium 2016;14:21-2.
- Hu J. Optimization of processing technology of Fructus Gardeniae Praeparatus and its effect on hemostasis and coagulation. Pharmacy and Clinics of Chinese Materia Medica 2018;9:9-11.
- Li W, Zhang C, Chen H, et al. Pharmacological study on elution parts of Fructus Gardeniae Ellis and stir-baked Fructus Gardeniae Praeparatus by 50% or 95% alcohol separately. Chinese Journal of Experimental Traditional Medical Formulae 2007;13.
- Li H, Yang H, Xue X, et al. A metabolomics approach to study the dual modulation by characterization of chemical alteration during processing of Gardeniae Fructus using UPLC-ESI-QTOF. Analytical Methods 2016;8:3629-35. [Crossref]
- Zhang HY, Liu H, Yang M, et al. Antithrombotic activities of aqueous extract from Gardenia jasminoides and its main constituent. Pharm Biol 2013;51:221-5. [Crossref] [PubMed]
- Li W, Zhang C, Chen H, et al. Comparative pharmacodynamic study on crocin-1 and crocetin. Chinese Journal of Experimental Traditional Medical Formulae 2007;13:24-7.
- Yao L, Meng J, Zhang C, et al. Content of the tanning and absorption capacity of carbon in different processed Gardenia slices. Chinese Journal of Experimental Traditional Medical Formulae 2014;20:45-8.
- Liu F, Li Y, Li M, et al. Study on Mechanism of Iridoid Glycosides Derivatives from Fructus Gardeniae in Jiangxi Province by Network Pharmacology. Evid Based Complement Alternat Med 2020;2020:4062813. [Crossref] [PubMed]
- Shi H, Dong C, Wang M, et al. Exploring the mechanism of Yizhi Tongmai decoction in the treatment of vascular dementia through network pharmacology and molecular docking. Ann Transl Med 2021;9:164. [Crossref] [PubMed]
- Zhang R, Zhu X, Bai H, et al. Network Pharmacology Databases for Traditional Chinese Medicine: Review and Assessment. Front Pharmacol 2019;10:123. [Crossref] [PubMed]
- Wang X, Wang ZY, Zheng JH, et al. TCM network pharmacology: A new trend towards combining computational, experimental and clinical approaches. Chin J Nat Med 2021;19:1-11. [Crossref] [PubMed]
- Xia MY, Wang Y, Zheng Y, et al. Quality changes of Gardeniae Fructus Praeparatus processed by different frying methods: a color-component correlation analysis. Zhongguo Zhong Yao Za Zhi 2021;46:2197-206. [PubMed]
- Ru J, Li P, Wang J, et al. TCMSP: a database of systems pharmacology for drug discovery from herbal medicines. J Cheminform 2014;6:13. [Crossref] [PubMed]
- Kuhn M, Szklarczyk D, Pletscher-Frankild S, et al. STITCH 4: integration of protein-chemical interactions with user data. Nucleic Acids Res 2014;42:D401-7. [Crossref] [PubMed]
- Daina A, Michielin O, Zoete V. SwissTargetPrediction: updated data and new features for efficient prediction of protein targets of small molecules. Nucleic Acids Res 2019;47:W357-64. [Crossref] [PubMed]
- Wang X, Wang GC, Rong J, et al. Identification of Steroidogenic Components Derived From Gardenia jasminoides Ellis Potentially Useful for Treating Postmenopausal Syndrome. Front Pharmacol 2018;9:390. [Crossref] [PubMed]
- Shi H, Tian S, Tian H. Network Pharmacology Interpretation of Fuzheng-Jiedu Decoction against Colorectal Cancer. Evid Based Complement Alternat Med 2021;2021:4652492. [Crossref] [PubMed]
- UniProt Consortium. UniProt: the universal protein knowledgebase in 2021. Nucleic Acids Res 2021;49:D480-9. [Crossref] [PubMed]
- Amberger JS, Hamosh A. Searching Online Mendelian Inheritance in Man (OMIM): A Knowledgebase of Human Genes and Genetic Phenotypes. Curr Protoc Bioinformatics 2017;58:1.2.1-1.2.12.
- Stelzer G, Rosen N, Plaschkes I, et al. The GeneCards Suite: From Gene Data Mining to Disease Genome Sequence Analyses. Curr Protoc Bioinformatics 2016;54:1.30.1-1.30.33.
- Piñero J, Queralt-Rosinach N, Bravo À, et al. DisGeNET: a discovery platform for the dynamical exploration of human diseases and their genes. Database (Oxford) 2015;2015:bav028. [Crossref] [PubMed]
- Li X, Xu Y. Unraveling the Molecular Mechanisms of Fructus Anisi Stellati as a Remedy for Infantile Colic by Network Pharmacology. Evid Based Complement Alternat Med 2020;2020:9210304. [Crossref] [PubMed]
- Shannon P, Markiel A, Ozier O, et al. Cytoscape: a software environment for integrated models of biomolecular interaction networks. Genome Res 2003;13:2498-504. [Crossref] [PubMed]
- Zhou Y, Zhou B, Pache L, et al. Metascape provides a biologist-oriented resource for the analysis of systems-level datasets. Nat Commun 2019;10:1523. [Crossref] [PubMed]
- Fabregat A, Sidiropoulos K, Viteri G, et al. Reactome diagram viewer: data structures and strategies to boost performance. Bioinformatics 2018;34:1208-14. [Crossref] [PubMed]
- Vella D, Marini S, Vitali F, et al. MTGO: PPI Network Analysis Via Topological and Functional Module Identification. Sci Rep 2018;8:5499. [Crossref] [PubMed]
- Chen S, Shi Y, Yang N, et al. UPLC-PDA fingerprint of Gardeniae Fructus from Houshan District, Chongqing. Chinese Traditional Patent Medicine 2012;34:2376-80.
- Jiang M, Gao PF, Li HQ, et al. Ghrelin inhibition of ethanol-induced gastric epithelial cell apoptosis is mediated by miR-21. Int J Clin Exp Pathol 2015;8:4662-72. [PubMed]
- Li Q, Fan J, Han J. Chinese herbal remedies affecting thrombosis and hemostasis: a review. World Journal of Traditional Chinese Medicine 2015;1:40-51. [Crossref]
- Kasimu R, Wang X, Wang X, et al. Antithrombotic effects and related mechanisms of Salvia deserta Schang root EtOAc extracts. Sci Rep 2018;8:17753. [Crossref] [PubMed]
- Badimon L, Vilahur G, Rocca B, et al. The key contribution of platelet and vascular arachidonic acid metabolism to the pathophysiology of atherothrombosis. Cardiovasc Res 2021;117:2001-15. [Crossref] [PubMed]
- Braune S, Küpper JH, Jung F. Effect of prostanoids on human platelet function: en overview. Int J Mol Sci 2020;21:9020. [Crossref] [PubMed]
- Whittle BJ, Hamid S, Lidbury P, et al. Specificity between the anti-aggregatory actions of prostacyclin, prostaglandin E1 and D2 on platelets. Adv Exp Med Biol 1985;192:109-25. [Crossref] [PubMed]
- Basu S. Novel cyclooxygenase-catalyzed bioactive prostaglandin F2α from physiology to new principles in inflammation. Med Res Rev 2007;27:435-68. [Crossref] [PubMed]
- Wu H, Tang C, Tai LW, et al. Flurbiprofen axetil attenuates cerebral ischemia/reperfusion injury by reducing inflammation in a rat model of transient global cerebral ischemia/reperfusion. Biosci Rep 2018;38:BSR20171562. [Crossref] [PubMed]
- Li F, Qiu S, Yin J, et al. Effects of common-used bitter-cold herbs on gastrointestinal hormone in rats. Journal of TCM Univ of Hunan 2007;27:9-11.
- Wu H, Chen Y, Wei W, et al. Effects and mechanisms of treating adjuvant arthritis by total glucosides of Cape Jasmine in rat. Chinese Journal of Experimental Traditional Medical Formulae 2008;14:49-52.
- Crescente M, Menke L, Chan MV, et al. Eicosanoids in platelets and the effect of their modulation by aspirin in the cardiovascular system (and beyond). Br J Pharmacol 2019;176:988-99. [Crossref] [PubMed]
- Li RHL, Nguyen N, Tablin F. Canine platelets express functional Toll-like receptor-4: lipopolysaccharide-triggered platelet activation is dependent on adenosine diphosphate and thromboxane A2 in dogs. BMC Vet Res 2019;15:245. [Crossref] [PubMed]
- Megale AAA, Portaro FC, Da Silva WD. Bitis arietans Snake Venom Induces an Inflammatory Response Which Is Partially Dependent on Lipid Mediators. Toxins (Basel) 2020;12:594. [Crossref] [PubMed]
- van der Meer JH, van der Poll T, van 't Veer C. TAM receptors, Gas6, and protein S: roles in inflammation and hemostasis. Blood 2014;123:2460-9. [Crossref] [PubMed]
- Tschoe C, Bushnell CD, Duncan PW, et al. Neuroinflammation after Intracerebral Hemorrhage and Potential Therapeutic Targets. J Stroke 2020;22:29-46. [Crossref] [PubMed]
- St Laurent G 3rd, Seilheimer B, Tackett M, et al. Deep Sequencing Transcriptome Analysis of Murine Wound Healing: Effects of a Multicomponent, Multitarget Natural Product Therapy-Tr14. Front Mol Biosci 2017;4:57. [Crossref] [PubMed]
- Wada Y, Suzuki A, Ishiguro H, et al. Chronological Gene Expression of Human Gingival Fibroblasts with Low Reactive Level Laser (LLL) Irradiation. J Clin Med 2021;10:1952. [Crossref] [PubMed]
- Xu Z, Xu YJ, Hao YN, et al. A novel STAT3 inhibitor negatively modulates platelet activation and aggregation. Acta Pharmacol Sin 2017;38:651-9. [Crossref] [PubMed]
- Wang Q, Chang H, Shen Q, et al. Photobiomodulation therapy for thrombocytopenia by upregulating thrombopoietin expression via the ROS-dependent Src/ERK/STAT3 signaling pathway. J Thromb Haemost 2021;19:2029-43. [Crossref] [PubMed]
- De Kock L, Freson K. The (Patho)Biology of SRC Kinase in Platelets and Megakaryocytes. Medicina (Kaunas) 2020;56:633. [Crossref] [PubMed]
- Barozzi S, Di Buduo CA, Marconi C, et al. Pathogenetic and clinical study of a patient with thrombocytopenia due to the p.E527K gain-of-function variant of SRC. Haematologica 2021;106:918-22. [Crossref] [PubMed]
- Zhao X, Sun P, Li G, et al. Polyphenols in Kuding tea help prevent HCl/ethanol-induced gastric injury in mice. Food Funct 2018;9:1713-25. [Crossref] [PubMed]
- Yang X, Yang L, Pan D, et al. Wheat peptide protects against ethanol-induced gastric mucosal damage through downregulation of TLR4 and MAPK. Journal of Functional Foods 2020;75:104271. [Crossref]
- Park E, Lee SM, Jung IK, et al. Effects of genistein on early-stage cutaneous wound healing. Biochem Biophys Res Commun 2011;410:514-9. [Crossref] [PubMed]
- Kelly LA, Seidlova-Wuttke D, Wuttke W, et al. Estrogen receptor alpha augments changes in hemostatic gene expression in HepG2 cells treated with estradiol and phytoestrogens. Phytomedicine 2014;21:155-8. [Crossref] [PubMed]
- Chen Y, Chen Q, Wang X, et al. Hemostatic action of lotus leaf charcoal is probably due to transformation of flavonol aglycons from flavonol glycosides in traditional Chinses medicine. J Ethnopharmacol 2020;249:112364. [Crossref] [PubMed]