Immunosenescence is a therapeutic target for frailty in older adults: a narrative review
Introduction
Aging refers to the progressive decrease in functional performance, leading to increased mortality risk (1). It is estimated that the proportion of the population aged ≥65 years will increase from 9% (1/9) in 2019 to 16% (1/6) by 2050. Currently, life expectancy is exhibiting an increasing trend worldwide and is expected to exceed 80 years by 2040 (2). This increase in life expectancy also indicates the potential increase in aging-associated diseases, such as cardiovascular, neurological, musculoskeletal, and oncological diseases (3,4). However, whether aging is a programmed process or the consequence of the accumulation of stress events remains debatable. Thus, more scientific research is needed to fully understand the underlying mechanisms involved in aging and age-related diseases to improve the management of complex and frail patients.
Frailty is a highly prevalent geriatric syndrome (5) that has attracted significant attention from physicians and researchers due to its associated increase in vulnerability and healthcare costs, especially in the elderly population. Generally, frail patients suffer from multiple chronic diseases, with comorbidities and polypharmacy greatly challenging their health management (5). Gerontologists suggest that targeting the common pathogenesis of comorbidities rather than a single disease is probably a better solution for older people.
Immunosenescence is defined as age-related changes in immune functions and has been extensively researched in human and animal models in the past decades (6). Recently, emerging evidence has supported immunosenescence as one of the common pathogeneses of comorbidity, which may lead to cancer, autoimmune disorder, repeated infection, chronic inflammation-related diseases, poor response to a vaccine, etc. (7-9). In particular, since early 2020 during the coronavirus disease 2019 (COVID-19) pandemic, severe respiratory syndrome coronavirus 2 has led to devastating effects on the entire population (10). In the United States, it was shown that 80% of the total deaths occurred in patients aged >65 years (11), and older adults were more likely to develop acute respiratory distress syndrome (ARDS) and end-stage multiple organ failure (12). These phenomena were highly associated with the aging immune system, making the elderly a highly vulnerable population (13). Thus, given the plethora of evidence suggesting immunosenescence as the underlying mechanism for frailty, we hypothesized that targeting specific age-related factors contributing to immunosenescence could potentially delay the aging process and provide time for preventing age-related diseases.
In the current literature, most reviews on immunosenescence and frailty focused more on specific pathways or cell types, and less on therapeutic methods for senile frailty syndrome. The search strategy summary as shown in Table 1. This review summarizes current knowledge on aging and the immune system, elaborates on the mechanism through which immunosenescence contributes to frailty, and discusses the possibility of targeting the aging immune system as a therapeutic method for senile frailty syndrome, with some emphasis on the effects of the COVID-19 pandemic on the elderly, objective to provide reference for clinical work. We present the following article in accordance with the Narrative Review reporting checklist (available at https://atm.amegroups.com/article/view/10.21037/atm-22-4405/rc).
Table 1
Items | Specification |
---|---|
Date of search | Jan 24th, 2022 |
Databases and other sources searched | PubMed, Google Scholar, Web of Science |
Search terms used | Immunosenescence, inflamm-aging, frailty, sarcopenia, skeletal muscle, immune aging |
Timeframe | 1989–2021 |
Inclusion and exclusion criteria | Content related to search keywords |
Selection process | Selection process was conducted independently by Chuanfeng Liu and Yuan Tian. If there were any discrepancy, they would be escalated to WenZhong for further discussion till final decision |
Methods
Literature probing for this narrative review was conducted by searching PubMed, Google Scholar and Web of Science database for articles published from 1989–2021. The search terms used (including MeSH and free text search terms and filters) are as follows: immunosenescence, inflamm-aging, frailty, sarcopenia, skeletal muscle and immune aging. There is no limitation set on study type during searching, but only articles in English are included in searching strategy. Selection process in conducted independently by Chuanfeng Liu and Yuan Tian. If any discrepancy, they will escalate issue to WenZhong for further discussion till final decision.
Defining frailty
Frailty is a critical public health problem in the older population. How we define frailty will determine the way we explore its management. In a consensus published by the International Association of Gerontology and Geriatrics in 2013, frailty was defined as reduced strength and physiological dysfunction, resulting in elevated weakness, dependence, or death (14). Frailty is a non-specific state wherein the decreased physiological reserve of older individuals increases their vulnerability and decreases anti-stress capability (15). Frailty involves pathological and physiological changes in multiple systems, including the neuroendocrine, musculoskeletal, metabolism, and immune systems (16).
The prevalence of frailty varies across countries and regions, not only because of the huge disparities in race, economic status, and medical care but also because of the lack of a universally accepted evaluation method for frailty syndrome. At present, two evaluation scales exist for frailty: the Fried Frailty Phenotype (17) and the Rockwood frailty index (18). Frailty phenotype refers to an independent clinical syndrome characterized by an imbalanced adaptive regulatory system and energy, which is the physiological basis of sarcopenia and fatigue. The frailty index is characterized by a wide range of dimensions, including physical function, comorbidities, cognitive and mental factors, etc. Irrespective of the scale used, both can predict the mortality, disability, falls, and hospitalization risks of older adults. Simultaneously, both evaluation tools prioritize lean body mass and physical capability. Thus, the Fried Frailty Phenotype (17) and Rockwood frailty index (18) scales are important referential tools that can be used to provide a more standardized assessment of frailty.
Physical capability decline is a core phenotype of frailty
Muscle mass and functional loss are the typical clinical manifestation of frail patients, which can also serve as the key diagnostic criteria of sarcopenia (19). As a geriatric syndrome, sarcopenia shows generalized and progressive loss of muscle mass and malfunction. It increases the risk of falls and fractures, contributes to the loss of independence and poor quality of life, is associated with cardiac or respiratory disease and cognitive decline, and leads to high rates of hospitalization and death. In the previous study (20), the main diagnostic criteria were muscle mass, strength, and physical performance. Until 2018, muscle quality was based on the revised European consensus on sarcopenia (20). Physical capability is the main diagnostic criterion of both frailty and sarcopenia; thus, skeletal muscle health is the research focus of preventative and therapeutic strategies.
Multiple factors can contribute to skeletal muscle degenerative change in addition to aging; thus, sarcopenia is classified as primary and secondary accordingly. Emerging evidence has shown that numerous diseases or pathological statuses, such as diabetes mellitus, cancer, and kidney failure with hemodialysis, can lead to muscle wasting. Therefore, the coexistence of multiple diseases is a risk factor for both frailty and sarcopenia. The potential mechanism, including but not limited to proteostasis loss, chronic inflammation, and mitochondrial dysfunction, is still under investigation.
Pathophysiological basis of immunosenescence
Compared to younger people, the immune profile of older people exhibits a declining trend involving innate and adaptive immunity. The decline in immune capability can lead to several clinical manifestations, such as poor response to a vaccine, lower antitumor ability, repeated and persistent infections, and autoimmune diseases. This age-related process is called immunosenescence (21), a detrimental factor for healthy aging and lifespan. Immunosenescence accounts for senile morbidity and death (22) and has the following characteristics: antigen presentation impairment, naïve T-cell priming, diminished cluster of differentiation (CD) 8+ T cell cytotoxic function, shrinkage of naïve B-cell and T-cell repertoires, and the production of lower amounts of high acidity antibodies (23).
Adaptive immunity involves the self-activation, growth, and differentiation of antigen-specific T/B lymphocytes into effector cells following stimulation by an antigen, resulting in a series of biological effects. B cells, CD4+ T cells, and CD8+ T cells are the main effective lymphocytes in the adaptive immune system. In addition to producing cytokines, CD8+ T cells also play a role in directly killing pathogen-infected cells. However, the adaptive immune system wanes with aging, and thymic degeneration induces reduced production of naïve T cells. Typically, immunosenescence status is determined by obvious alterations in the adaptive immune system during the aging process (24).
Innate immunity is the intrinsic defense mechanism of the body under a physiological condition that produces related immune responses upon pathogen or foreign body invasion. The innate immune system mainly contains the following cell types: natural killer cells, macrophages, neutrophils, and dendritic cells (DCs), which react quickly to identify and eliminate pathogens that may cause infection. However, the proportion of macrophages, chemotaxis, antigen presentation ability, and phagocytosis will decrease with aging, resulting in increased susceptibility of older individuals (25). DCs are responsible for antigen presentation; a previous study revealed that DCs have a poor ability to activate CD4+ T cells in the elderly (26).
Inflamm-aging is a chronic inflammatory state that is completely different from acute inflammation, characterized by persistent production of undecomposed pro-inflammatory mediators. On the one hand, immunosenescence results in inflammation and pro-inflammatory factor accumulation. On the other hand, senescent cells can generate angiogenic factors, chemokines, cytokines, proteases, and growth factors, also referred to as the senescence-associated secretory phenotype (SASP) (27). With the accumulation of aging cells, SASP may also induce age-related inflammation. Thus, inflamm-aging may be caused by the interaction of complex factors such as SASP and innate immune cell dysfunction in older adults (28). Decades of accumulation of stress events alter the immune systems of elderly individuals, leading to time-related destruction of immune organ structure and dysfunction of immune responses due to varying levels of inflammation. Thus, immunosenescence and inflamm-aging are considered the common pathogenesis basis of multiple geriatric diseases.
Immunosenescence is associated with comorbidity in older adults
A study published in Nature in 2021 showed that the senescence immune system has a crucial effect on driving systemic aging (29). Emerging evidence has revealed that immunosenescence is closely associated with multiple chronic age-related diseases, whereas comorbidity is a risk factor for frailty syndrome.
Immunosenescence (particularly inflamm-aging) is associated with neurodegenerative disorders and cognitive decline. As observed in a cohort study involving 873 people without dementia aged 70–90 years, a higher expression of interleukin (IL)-6, IL-10, IL-12, IL-1β, and tumor necrosis factor (TNF)-α predicted low cognitive function when confounding factors such as age, sex, education, and obesity were adjusted (30). The underlying mechanism may be that long-run and/or excess pro-inflammatory factor release into the central nervous system results in decreased expression of brain-derived neurotrophic factor, which is associated with neurogenesis, glutamate activation, oxidative stress, and apoptosis induction (31). A previous study depicted the immune signature by counting the number of peripheral blood mononuclear cells (PBMCs) among patients with Alzheimer’s disease (AD): an increase in CD8+ T effector memory CD45 RA+ cells. These cells accumulate near the Aβ plaques of the brain and are inversely associated with cognitive ability (32).
Moreover, a relationship between immunosenescence and metabolism disorder has been detected, although it is not a causal relationship. Previous view demonstrated that endocrinosenescence modulates the immune system and immunosenescence changes the endocrine system, forming a vicious circle (33). The decrease of hormones may be linked to the increase of cytokines such as IL-6 and TNF, while an increase of TNF and IL-1β in the vicinity of endocrine glands may inhibit hormone production and secretion. Furthermore, endocrine disorder may participate in the pathogenesis of frailty through aberrant regulation of glucocorticoid secretion, insulin-like growth factor signalling, androgen production as well as insulin resistance (34). A classic feature of type 2 diabetes mellitus (T2DM) immunosenescence is the decreased CD4+ naive T cell repertoire accompanied by the increased effector CD4+/CD8+ T cell and memory CD4+ T cell repertoires. The senescent or late-differentiated T cells (CD8+CD28−, CD8+CD57+) have recently been shown to predict hyperglycemia in humans (35). A study revealed that the increased expression of glycosylated hemoglobin is associated with the decreased phagocytosis of neutrophils and monocytes in circulation, whereas metformin treatment reversed its expression (36). In addition, plasma mediators in obese individuals may promote the early onset of immunosenescence. The exposure of healthy PBMCs in the plasma of obese people exhibits a decreased expression of CD28 (a representative marker for T cell aging) and an increased late-differentiated CD8+CD28− T cell count (37).
Immunosurveillance means that immune cells monitor and control defective or aging cells, thereby avoiding tumor cell development. The immune system may be responsible for recognizing and eliminating cancer precursors (38), mainly regulated by cytotoxic natural killer NK and CD8+ T cells. On the other hand, the interaction between immune and cancer cells occurs during the formation and development of cancer cells, a process known as immune editing (39). Tumor immunoediting includes elimination, balancing, and tumor escape, all of which are affected by immunosenescence. Thus, poor immunosurveillance is a non-negligible component of immunosenescence, which is one possible explanation for cancer occurrence.
Can a different immune profile affect prognosis or survival?
Emerging evidence has revealed that the immune profile varies between older individuals and their young counterparts and affects healthspan and mortality. A study that began in 1979 assessed the responses to mitogen polyhydroxyalkanoates among 246 people aged >65 years. According to their results, the different responses of baseline T cells resulted in differences in the 4-year survival after a mean follow-up of 36.5 years (40). In a Japanese study, people with a baseline age of 69–72 years were followed up for more than 7 years. The authors discovered that the reduced chemotaxis of polymorphonuclear leukocytes was significantly associated with mortality (41). In a Swedish study, the mortality-related immunological parameters were systemically analyzed in 102 patients aged 86–92 years at baseline who were followed up for 2 years. At the last follow-up, which was conducted 8 years after the study began, it was reported that non-survivors showed a decreased proportion of CD4+ T cells and an increased proportion of CD8+ T cells (42). In addition, some immune parameters exhibited a close association with early mortality in older patients, including poor T cell response to mitogen proliferation, a high percentage of CD8+ T cells, and a low percentage of CD4+ T cells and CD19+ B cells (40). Thus, age-related alterations of the immune system can indeed affect the prognosis of elderly patients and the mortality risk of these people.
Skeletal muscle plays a central role between sarcopenia and immunosenescence
Skeletal muscles are a well-recognized therapeutic target of sarcopenia and frailty. Recently, researchers have proposed that the skeletal muscle is not only the largest organ for physical movement but can also regulate an immunological process and the inflammatory response (43). Moreover, researchers even considered that skeletal muscle possibly plays a central role between sarcopenia and immunosenescence (44).
Emerging evidence has demonstrated a link between sarcopenia and a higher risk of infection among senile cases. Moreover, sarcopenia was found to be associated with a risk of infection after surgery (45). The risk of nosocomial infection increased two-fold in hospitalized patients following a 3-week treatment course in the hospital. In addition, sarcopenia can predict the community-acquired pneumonia risk in older people (46) and the 90-day mortality of older patients with pneumonia (47). Poor immune system response to a pathogen is a possible mechanism for the vulnerability of elderly adults to infectious diseases.
As an endocrine organ, skeletal muscle secretes more than 300 myokines (48); several myokines have exhibited immune system-modulating properties. For example, IL-15 plays a critical role in the immune system, regulating NK cell activation, growth, and distribution; modulating CD8 T-cell homeostasis (49); and reinforcing neutrophil migration and phagocytosis (50). Another myokine, IL-6, is well known for accelerating muscle protein degradation and interstitial fibrosis deposition by modulating the Signal Transducer And Activator Of Transcription 3 STAT3-IL-6 signaling pathway. Interestingly, another study showed that IL-6 induced the secretion of IL-1ra and IL-10 (51), both of which inhibit inflammation.
Accordingly, immune cells affect the regenerative ability of skeletal muscles because infiltrated immune cells produce growth factors, which are essential for muscle stem cell proliferation and differentiation, and remove necrotic cells (52). IL-15 is beneficial for skeletal muscles as it promotes myogenesis while regulating the distribution of adipose tissues. Another study showed that a certain proportion of regulatory T cells promote muscle repair by regulating amphiregulin, the growth factor targeting muscle stem cells (53). Thus, the interaction between skeletal muscles and immunosenescence determines the homeostasis of the immune-muscle system, which is worthy of further study.
Intervention for immunosenescence and frailty
Multiple factors contribute to development of frailty with advancing age, thus the therapeutic target is diversed depends on specific condition. Nutrition supplements and physical exercise are proved to be helpful in preventing and treatment of frailty, however, valid pharmaceutical intervention is scarce. Mesenchymal stem cells (MSCs) can exert regenerative effects and possess anti-inflammatory properties, offering a promising therapeutic strategy to address the pathophysiologic problems of frail syndrome (54). Currently, MSC therapy is undergoing the phase I and II trials in human subjects to endorse the safety and efficacy of MSCs for aging frailty. Furthermore, as a gradually shifting of treatment concept for elderly, “one disease at a time” model is no longer appropriate in geriatric clinical practice (55). To targeting one common mechanism of frailty and multiple chronic diseases of elderly is potential therapeutic strategy, while immunosenescence is one of several common mechanisms which not only causes physiological decline but also be involved in development of several chronic geriatric diseases.
Immune aging, or immunosenescence, contributes to the morbidity and mortality of the elderly (56). Matthew’s study selectively deleted Ercc1 in mouse haematopoietic cells to increase the burden of endogenous DNA damage and thereby senescence (57) in the immune system only and found senescent immune system has a causal role in driving systemic ageing and therefore represents a crucial therapeutic target to extend healthspan (29).
Numerous studies have shown that rapamycin and rapalogs, considered novel and promising longevity agents, can extend lifespan (58). Interestingly, these agents showed an immunosuppressive effect at high doses and an immune stimulatory effect at low doses (59). However, the reason for these immunity-boosting effects is unclear. The inhibition of mammalian target of rapamycin (mTORC1) is a possible explanation, as mTOR can regulate the STAT signaling pathway. A study showed a significant difference in STAT phosphorylation levels in the T cells of healthy people compared with unhealthy senescent people (60).
Senolytics are a novel type of agent. The interference of stem cell signaling pathways temporarily disables senescent cell anti-apoptotic pathway (SCAP), thus targeting selectively senescent cells. In addition to its main effect on clearing senescent cells, senolytics can also eliminate pro-inflammatory cytokines. According to the study (61), inflammation symptoms are relieved after the administration of senolytics. A recent study found reduced SASP and coronavirus-related mortality in old mice after the administration of senolytics (62).
A low level of nicotinamide adenine dinucleotide (NDA)+ is reportedly associated with the poor function of mitochondria and metabolic reprogramming of immune cells (63); therefore, NDA+ is also recognized as a therapeutic target for aging immunity. Promising data has demonstrated that administrating nicotinamide mononucleotide, the NAD+ precursor, into mice could maintain NAD+ levels and mitochondrial function (64), with the mitochondrial function of immunocytes being essential for controlling virus propagation (65).
A centenarian study revealed that longevity is associated with gut microbial structures, making individuals more potent against age-associated disorders and leading to a longer life (66). The microbiota-targeting probiotic and dietary interventions affect natural aging by enhancing oxidation resistance, regulating metabolism, suppressing chronic inflammation, and promoting immune homeostasis (67). Immunosenescence may have a certain influence on human microbial composition, function, and diversity. In addition, fecal microbiota transplantation or prebiotic/probiotic/synbiotic supplementation in the diet is beneficial for restoring active microbiota and extending a healthy lifespan (68). Thus, there are multiple ongoing developments in this field to ease the process of aging and reduce the risk of potential disabilities that could lead to a significant decrease in the quality of life of elderly individuals.
Effects of the COVID-19 pandemic on the elderly
The COVID-19 pandemic began in late 2019 and has spread worldwide. At the time of writing this manuscript, more than 6 million people have died, and nearly 551 million people have been infected, with up to 18 million active cases worldwide (69). Taking the age group of 18–29 years as a reference, it is reported that the rates of hospitalization in the 65–74, 75–84, and ≥85 years old groups are 5×, 8×, and 10× greater than the reference group and their corresponding COVID-19 related deaths are 60×, 140×, and 330× that of the reference group, respectively (70). Thus, these data indicate a very strong correlation between age and COVID-19 severity and mortality.
When SARS-CoV-2 viruses enter the body, their viral DNA is detected by immune cells such as macrophages and DCs, which stimulate inflammasome and lead to the secretion of cytokines such as IL-6, Monocyte chemoattractant protein-1 (MCP-1), Macrophage inflammatory protein-1 alpha (MIP-1α), TNF-α, and virus-protective type 1 interferons (71). These directly activate the innate responses and indirectly guide adaptive responses to the virus in multiple organs such as the lungs, brain, intestines, kidneys, etc. Although these responses may have protective effects against the infection, they might also lead to the overactivation of inflammasomes, leading to “cytokine storms”, damage to affected organs, disseminated intravascular coagulation (DIC), ARDS, multi-organ failure, and ultimately death.
Older people are at higher risk of developing cytokine storms due to their vulnerability to mitochondrial dysfunction, increased oxidative stress, poor nutrition, restricted activity (especially during frailty syndrome), and immune dysregulation (72-75); of which the latter two are important processes of immunosenescence and inflamm-aging. It has also been reported that increased inflamm-aging is associated with an increase in COVID-19 susceptibility (76). The functional effectiveness of the adaptive immune cells depends mainly on their diversity to recognize antigen receptors, ability to initiate robust proliferative responses (importantly, the production of large numbers of relevant lymphocyte clones), and manufacture of effector and immunoregulatory humoral factors (77). However, aging lymphocytes (lymphocytes in older people) have lower effectiveness than those in younger people, and most older adults have comparatively fewer B and T cells to respond to proliferative stimulation due to the decreased output of the thymus with aging and a higher proportion of non-proliferating lymphocytes in the elderly (78). Thus, the lower output of important immune organs to produce timely, viable, and lasting immune responses, the decreased capability of vital organs in excreting toxins, and the impairment of old immune cells due to changes in autophagy, mitophagy, activities of the enzymes in lysosomes and proteasomes, etc. (79,80), all impair the functions of immune cells in elderly, significantly affecting their antiviral capabilities.
Immunosenescence is potential mechanism to elucidate poor vaccination response and infectious disease severity and lethality, however, the clinical data exploring associations between immunosenescence and vaccination in the elderly are incomplete. Lack of universally acknowledged biomarkers to depict the landscape of immune aging is possible explanation. “Immune risk phenotype”, with an inverted CD4/CD8 T cell ratio and presence of low grade inflammation, were used to evaluate immunosenescence (81). Although it might be prophylactically possible to protect the aging population from COVID-19, recent studies have shown that their lower response to vaccines might not be due to their immune system but rather related to the composition of the vaccines, as it was shown that modifying the antigen concentration and adjuvant concentration and composition in the flu, zoster, and anti-pneumococci vaccines could increase the response of the elderly immune system to that similar to obtained in vaccinated young people (82-85). Thus, further research is needed to fully determine the optimum compositions of anti-SARS-CoV-2 vaccines to maximize the immune system responses of elderly adults against the infection.
Conclusions
In conclusion, although immunosenescence is an unavoidable process of life, the process often differs from one subject to another. It is one of the multiple underlying pathogeneses for frailty and is the potential etiology of muscle aging. Developments in boosting the immune system and reducing inflammation during aging, such as through the use of pro-inflammatory cytokines to counterbalance inflammation and correct the modulation of immune responses and apoptosis, could allow people to age without significant disabilities. Thus, based on the combined literature findings, immunosenescence is a promising potential therapeutic target for frailty and is worthy of further investigation (Figure 1).
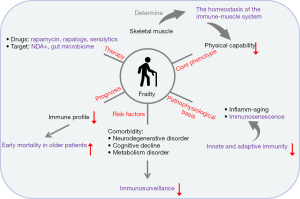
Acknowledgments
Funding: This research was supported by the National Natural Science Foundation of China (Grant No. 81804157), the China Scholarship Council (Grant No. 201908510004), and the 2019 Science and Technology Development Fund of Hospital of Chengdu University of Traditional Chinese Medicine (Grant No. 19HW05).
Footnote
Reporting Checklist: The authors have completed the Narrative Review reporting checklist. Available at https://atm.amegroups.com/article/view/10.21037/atm-22-4405/rc
Conflicts of Interest: All authors have completed the ICMJE uniform disclosure form (available at https://atm.amegroups.com/article/view/10.21037/atm-22-4405/coif). The authors have no conflicts of interest to declare.
Ethical Statement: The authors are accountable for all aspects of the work in ensuring that questions related to the accuracy or integrity of any part of the work are appropriately investigated and resolved.
Open Access Statement: This is an Open Access article distributed in accordance with the Creative Commons Attribution-NonCommercial-NoDerivs 4.0 International License (CC BY-NC-ND 4.0), which permits the non-commercial replication and distribution of the article with the strict proviso that no changes or edits are made and the original work is properly cited (including links to both the formal publication through the relevant DOI and the license). See: https://creativecommons.org/licenses/by-nc-nd/4.0/.
References
- da Costa JP, Vitorino R, Silva GM, et al. A synopsis on aging-Theories, mechanisms and future prospects. Ageing Res Rev 2016;29:90-112. [Crossref] [PubMed]
- Bleve A, Motta F, Durante B, et al. Immunosenescence, Inflammaging, and Frailty: Role of Myeloid Cells in Age-Related Diseases. Clin Rev Allergy Immunol 2022; Epub ahead of print. [Crossref] [PubMed]
- Foreman KJ, Marquez N, Dolgert A, et al. Forecasting life expectancy, years of life lost, and all-cause and cause-specific mortality for 250 causes of death: reference and alternative scenarios for 2016-40 for 195 countries and territories. Lancet 2018;392:2052-90. [Crossref] [PubMed]
- Wu Q, Yu X, Li J, et al. Metabolic regulation in the immune response to cancer. Cancer Commun (Lond) 2021;41:661-94. [Crossref] [PubMed]
- Thillainadesan J, Scott IA, Le Couteur DG. Frailty, a multisystem ageing syndrome. Age Ageing 2020;49:758-63. [Crossref] [PubMed]
- Kohut ML, Senchina DS. Reversing age-associated immunosenescence via exercise. Exerc Immunol Rev 2004;10:6-41. [PubMed]
- Allen JC, Toapanta FR, Chen W, et al. Understanding immunosenescence and its impact on vaccination of older adults. Vaccine 2020;38:8264-72. [Crossref] [PubMed]
- Dema M, Eixarch H, Villar LM, et al. Immunosenescence in multiple sclerosis: the identification of new therapeutic targets. Autoimmun Rev 2021;20:102893. [Crossref] [PubMed]
- Li N, Wu P, Shen Y, et al. Predictions of mortality related to four major cancers in China, 2020 to 2030. Cancer Commun (Lond) 2021;41:404-13. [Crossref] [PubMed]
- Witkowski JM, Fulop T, Bryl E. Immunosenescence and COVID-19. Mech Ageing Dev 2022;204:111672. [Crossref] [PubMed]
- Centers for Disease Control and Prevention, "COVID Data Tracker". Available online: https://covid.cdc.gov/covid-data-tracker
- Wu C, Chen X, Cai Y, et al. Risk Factors Associated With Acute Respiratory Distress Syndrome and Death in Patients With Coronavirus Disease 2019 Pneumonia in Wuhan, China. JAMA Intern Med 2020;180:934-43. [Crossref] [PubMed]
- Bartleson JM, Radenkovic D, Covarrubias AJ, et al. SARS-CoV-2, COVID-19 and the Ageing Immune System. Nat Aging 2021;1:769-82. [Crossref] [PubMed]
- Morley JE, Vellas B, van Kan GA, et al. Frailty consensus: a call to action. J Am Med Dir Assoc 2013;14:392-7. [Crossref] [PubMed]
- Walston J, Hadley EC, Ferrucci L, et al. Research agenda for frailty in older adults: toward a better understanding of physiology and etiology: summary from the American Geriatrics Society/National Institute on Aging Research Conference on Frailty in Older Adults. J Am Geriatr Soc 2006;54:991-1001. [Crossref] [PubMed]
- Clegg A, Young J, Iliffe S, et al. Frailty in elderly people. Lancet 2013;381:752-62. [Crossref] [PubMed]
- Fried LP, Tangen CM, Walston J, et al. Frailty in older adults: evidence for a phenotype. J Gerontol A Biol Sci Med Sci 2001;56:M146-56. [Crossref] [PubMed]
- Mitnitski AB, Mogilner AJ, Rockwood K. Accumulation of deficits as a proxy measure of aging. ScientificWorldJournal 2001;1:323-36. [Crossref] [PubMed]
- Abe S, Ezaki O, Suzuki M. Effects of Timing of Medium-Chain Triglycerides (8:0 and 10:0) Supplementation during the Day on Muscle Mass, Function and Cognition in Frail Elderly Adults. J Frailty Aging 2022;11:100-8. [PubMed]
- Cruz-Jentoft AJ, Bahat G, Bauer J, et al. Sarcopenia: revised European consensus on definition and diagnosis. Age Ageing 2019;48:16-31. [Crossref] [PubMed]
- Pawelec G. Age and immunity: What is "immunosenescence"? Exp Gerontol 2018;105:4-9. [Crossref] [PubMed]
- St Sauver JL, Boyd CM, Grossardt BR, et al. Risk of developing multimorbidity across all ages in an historical cohort study: differences by sex and ethnicity. BMJ Open 2015;5:e006413. [Crossref] [PubMed]
- Weiskopf D, Weinberger B, Grubeck-Loebenstein B. The aging of the immune system. Transpl Int 2009;22:1041-50. [Crossref] [PubMed]
- Pawelec G. Hallmarks of human "immunosenescence": adaptation or dysregulation? Immun Ageing 2012;9:15. [Crossref] [PubMed]
- Ma HD, Deng YR, Tian Z, et al. Traditional Chinese medicine and immune regulation. Clin Rev Allergy Immunol 2013;44:229-41. [Crossref] [PubMed]
- Agrawal A, Agrawal S, Gupta S. Role of Dendritic Cells in Inflammation and Loss of Tolerance in the Elderly. Front Immunol 2017;8:896. [Crossref] [PubMed]
- Sun Y, Coppé JP, Lam EW. Cellular Senescence: The Sought or the Unwanted? Trends Mol Med 2018;24:871-85. [Crossref] [PubMed]
- Barbé-Tuana F, Funchal G, Schmitz CRR, et al. The interplay between immunosenescence and age-related diseases. Semin Immunopathol 2020;42:545-57. [Crossref] [PubMed]
- Yousefzadeh MJ, Flores RR, Zhu Y, et al. An aged immune system drives senescence and ageing of solid organs. Nature 2021;594:100-5. [Crossref] [PubMed]
- Trollor JN, Smith E, Agars E, et al. The association between systemic inflammation and cognitive performance in the elderly: the Sydney Memory and Ageing Study. Age (Dordr) 2012;34:1295-308. [Crossref] [PubMed]
- Miller AH, Maletic V, Raison CL. Inflammation and its discontents: the role of cytokines in the pathophysiology of major depression. Biol Psychiatry 2009;65:732-41. [Crossref] [PubMed]
- Gate D, Saligrama N, Leventhal O, et al. Clonally expanded CD8 T cells patrol the cerebrospinal fluid in Alzheimer's disease. Nature 2020;577:399-404. [Crossref] [PubMed]
- Straub RH, Miller LE, Schölmerich J, et al. Cytokines and hormones as possible links between endocrinosenescence and immunosenescence. J Neuroimmunol 2000;109:10-5. [Crossref] [PubMed]
- Clegg A, Hassan-Smith Z. Frailty and the endocrine system. Lancet Diabetes Endocrinol 2018;6:743-52. [Crossref] [PubMed]
- Lee YH, Kim SR, Han DH, et al. Senescent T Cells Predict the Development of Hyperglycemia in Humans. Diabetes 2019;68:156-62. [Crossref] [PubMed]
- Lecube A, Pachón G, Petriz J, et al. Phagocytic activity is impaired in type 2 diabetes mellitus and increases after metabolic improvement. PLoS One 2011;6:e23366. [Crossref] [PubMed]
- Parisi MM, Grun LK, Lavandoski P, et al. Immunosenescence Induced by Plasma from Individuals with Obesity Caused Cell Signaling Dysfunction and Inflammation. Obesity (Silver Spring) 2017;25:1523-31. [Crossref] [PubMed]
- Zitvogel L, Tesniere A, Kroemer G. Cancer despite immunosurveillance: immunoselection and immunosubversion. Nat Rev Immunol 2006;6:715-27. [Crossref] [PubMed]
- Kim R, Emi M, Tanabe K. Cancer immunoediting from immune surveillance to immune escape. Immunology 2007;121:1-14. [Crossref] [PubMed]
- Murdoch JC, Elwood M, Aye PS. Serial tests of T-cell function predict long-term survival in an elderly cohort from a Scottish general practice. J Prim Health Care 2020;12:21-8. [Crossref] [PubMed]
- Niwa Y, Kasama T, Miyachi Y, et al. Neutrophil chemotaxis, phagocytosis and parameters of reactive oxygen species in human aging: cross-sectional and longitudinal studies. Life Sci 1989;44:1655-64. [Crossref] [PubMed]
- Olsson J, Wikby A, Johansson B, et al. Age-related change in peripheral blood T-lymphocyte subpopulations and cytomegalovirus infection in the very old: the Swedish longitudinal OCTO immune study. Mech Ageing Dev 2000;121:187-201. [Crossref] [PubMed]
- Afzali AM, Müntefering T, Wiendl H, et al. Skeletal muscle cells actively shape (auto)immune responses. Autoimmun Rev 2018;17:518-29. [Crossref] [PubMed]
- Nelke C, Dziewas R, Minnerup J, et al. Skeletal muscle as potential central link between sarcopenia and immune senescence. EBioMedicine 2019;49:381-8. [Crossref] [PubMed]
- Nakanishi R, Oki E, Sasaki S, et al. Sarcopenia is an independent predictor of complications after colorectal cancer surgery. Surg Today 2018;48:151-7. [Crossref] [PubMed]
- Altuna-Venegas S, Aliaga-Vega R, Maguiña JL, et al. Risk of community-acquired pneumonia in older adults with sarcopenia of a hospital from Callao, Peru 2010-2015. Arch Gerontol Geriatr 2019;82:100-5. [Crossref] [PubMed]
- Maeda K, Akagi J. Muscle Mass Loss Is a Potential Predictor of 90-Day Mortality in Older Adults with Aspiration Pneumonia. J Am Geriatr Soc 2017;65:e18-22. [Crossref] [PubMed]
- Giudice J, Taylor JM. Muscle as a paracrine and endocrine organ. Curr Opin Pharmacol 2017;34:49-55. [Crossref] [PubMed]
- Conlon KC, Lugli E, Welles HC, et al. Redistribution, hyperproliferation, activation of natural killer cells and CD8 T cells, and cytokine production during first-in-human clinical trial of recombinant human interleukin-15 in patients with cancer. J Clin Oncol 2015;33:74-82. [Crossref] [PubMed]
- Girard D, Paquet ME, Paquin R, et al. Differential effects of interleukin-15 (IL-15) and IL-2 on human neutrophils: modulation of phagocytosis, cytoskeleton rearrangement, gene expression, and apoptosis by IL-15. Blood 1996;88:3176-84. [Crossref] [PubMed]
- Steensberg A, Fischer CP, Keller C, et al. IL-6 enhances plasma IL-1ra, IL-10, and cortisol in humans. Am J Physiol Endocrinol Metab 2003;285:E433-7. [Crossref] [PubMed]
- Saini J, McPhee JS, Al-Dabbagh S, et al. Regenerative function of immune system: Modulation of muscle stem cells. Ageing Res Rev 2016;27:67-76. [Crossref] [PubMed]
- Burzyn D, Kuswanto W, Kolodin D, et al. A special population of regulatory T cells potentiates muscle repair. Cell 2013;155:1282-95. [Crossref] [PubMed]
- Zhu Y, Ge J, Huang C, et al. Application of mesenchymal stem cell therapy for aging frailty: from mechanisms to therapeutics. Theranostics 2021;11:5675-85. [Crossref] [PubMed]
- Rockwood K. Frailty and aging medicine. Aging Med (Milton) 2019;2:4-6. [Crossref] [PubMed]
- Kennedy BK, Berger SL, Brunet A, et al. Geroscience: linking aging to chronic disease. Cell 2014;159:709-13. [Crossref] [PubMed]
- Xu M, Pirtskhalava T, Farr JN, et al. Senolytics improve physical function and increase lifespan in old age. Nat Med 2018;24:1246-56. [Crossref] [PubMed]
- Robida-Stubbs S, Glover-Cutter K, Lamming DW, et al. TOR signaling and rapamycin influence longevity by regulating SKN-1/Nrf and DAF-16/FoxO. Cell Metab 2012;15:713-24. [Crossref] [PubMed]
- Ferrer IR, Araki K, Ford ML. Paradoxical aspects of rapamycin immunobiology in transplantation. Am J Transplant 2011;11:654-9. [Crossref] [PubMed]
- Shen-Orr SS, Furman D, Kidd BA, et al. Defective Signaling in the JAK-STAT Pathway Tracks with Chronic Inflammation and Cardiovascular Risk in Aging Humans. Cell Syst 2016;3:374-384.e4. [Crossref] [PubMed]
- Robbins PD, Jurk D, Khosla S, et al. Senolytic Drugs: Reducing Senescent Cell Viability to Extend Health Span. Annu Rev Pharmacol Toxicol 2021;61:779-803. [Crossref] [PubMed]
- Camell CD, Yousefzadeh MJ, Zhu Y, et al. Senolytics reduce coronavirus-related mortality in old mice. Science 2021;373:eabe4832. [Crossref] [PubMed]
- Covarrubias AJ, Perrone R, Grozio A, et al. NAD+ metabolism and its roles in cellular processes during ageing. Nat Rev Mol Cell Biol 2021;22:119-41. [Crossref] [PubMed]
- Kiss T, Nyúl-Tóth Á, Balasubramanian P, et al. Nicotinamide mononucleotide (NMN) supplementation promotes neurovascular rejuvenation in aged mice: transcriptional footprint of SIRT1 activation, mitochondrial protection, anti-inflammatory, and anti-apoptotic effects. Geroscience 2020;42:527-46. [Crossref] [PubMed]
- Miller B, Silverstein A, Flores M, et al. Host mitochondrial transcriptome response to SARS-CoV-2 in multiple cell models and clinical samples. Sci Rep 2021;11:3. [Crossref] [PubMed]
- Cӑtoi AF, Corina A, Katsiki N, et al. Gut microbiota and aging-A focus on centenarians. Biochim Biophys Acta Mol Basis Dis 2020;1866:165765. [Crossref] [PubMed]
- Vaiserman AM, Koliada AK, Marotta F. Gut microbiota: A player in aging and a target for anti-aging intervention. Ageing Res Rev 2017;35:36-45. [Crossref] [PubMed]
- Coman V, Vodnar DC. Gut microbiota and old age: Modulating factors and interventions for healthy longevity. Exp Gerontol 2020;141:111095. [Crossref] [PubMed]
- Worldometer. COVID-19 Coronavirus pandemic. Available online: https://www.worldometers.info/coronavirus/
- CDC.gov. Risk for COVID-19 Infection, Hospitalization, and Death By Age Group. Available online: https://www.cdc.gov/coronavirus/2019-ncov/covid-data/investigations-discovery/hospitalization-death-by-age.html#print
- Khanmohammadi S, Rezaei N. Role of Toll-like receptors in the pathogenesis of COVID-19. J Med Virol 2021;93:2735-9. [Crossref] [PubMed]
- Farshbafnadi M, Kamali Zonouzi S, Sabahi M, et al. Aging & COVID-19 susceptibility, disease severity, and clinical outcomes: The role of entangled risk factors. Exp Gerontol 2021;154:111507. [Crossref] [PubMed]
- Pinti M, Cevenini E, Nasi M, et al. Circulating mitochondrial DNA increases with age and is a familiar trait: Implications for "inflamm-aging". Eur J Immunol 2014;44:1552-62. [Crossref] [PubMed]
- Rydyznski Moderbacher C, Ramirez SI, Dan JM, et al. Antigen-Specific Adaptive Immunity to SARS-CoV-2 in Acute COVID-19 and Associations with Age and Disease Severity. Cell 2020;183:996-1012.e19. [Crossref] [PubMed]
- Wissler Gerdes EO, Vanichkachorn G, Verdoorn BP, et al. Role of senescence in the chronic health consequences of COVID-19. Transl Res 2022;241:96-108. [Crossref] [PubMed]
- Santoro A, Bientinesi E, Monti D. Immunosenescence and inflammaging in the aging process: age-related diseases or longevity? Ageing Res Rev 2021;71:101422. [Crossref] [PubMed]
- Bandaranayake T, Shaw AC. Host Resistance and Immune Aging. Clin Geriatr Med 2016;32:415-32. [Crossref] [PubMed]
- Robert L, Fulop T. Aging of cell communication: loss of receptor function. Interdiscip Top Gerontol 2014;39:142-62. [Crossref] [PubMed]
- Witkowski JM, Bryl E, Fulop T. Proteodynamics and aging of eukaryotic cells. Mech Ageing Dev 2021;194:111430. [Crossref] [PubMed]
- Witkowski JM, Mikosik A, Bryl E, et al. Proteodynamics in aging human T cells - The need for its comprehensive study to understand the fine regulation of T lymphocyte functions. Exp Gerontol 2018;107:161-8. [Crossref] [PubMed]
- Wikby A, Nilsson BO, Forsey R, et al. The immune risk phenotype is associated with IL-6 in the terminal decline stage: findings from the Swedish NONA immune longitudinal study of very late life functioning. Mech Ageing Dev 2006;127:695-704. [Crossref] [PubMed]
- Fielding JE, Lambert SB. Adjuvanted Herpes Zoster Subunit Vaccine in Older Adults. N Engl J Med 2015;373:1576. [PubMed]
- George IA, Lawrence SJ. Adjuvanted Herpes Zoster Subunit Vaccine in Older Adults. N Engl J Med 2015;373:1575-6. [Crossref] [PubMed]
- Goudsmit J, van den Biggelaar AHJ, Koudstaal W, et al. Immune age and biological age as determinants of vaccine responsiveness among elderly populations: the Human Immunomics Initiative research program. Eur J Epidemiol 2021;36:753-62. [Crossref] [PubMed]
- Lal H, Cunningham AL, Heineman TC. Adjuvanted Herpes Zoster Subunit Vaccine in Older Adults. N Engl J Med 2015;373:1576-7. [Crossref] [PubMed]
(English Language Editor: A. Kassem)