The recycling of AMPA receptors/GABAa receptors is related to neuronal excitation/inhibition imbalance and may be regulated by KIF5A
Introduction
In recent years, it has been established that the mechanism underlying seizures involves an increase in neuroexcitatory function mediated by glutamatergic neurons and a decrease in neuroinhibitory function mediated by gamma aminobutyric acid (GABA) neurons. The balance between these 2 functions is the excitation/inhibition (E/I) balance. E/I imbalance is a well-recognized factor in seizures (1). Recurrent seizures can cause hippocampal sclerosis which could lead to cognitive impairment (2). The longer the E/I imbalance would lead to Status epilepticus (SE), which is a neurological emergency (3,4). Patients could benefit from the timely termination of seizures, which in turn could prevent the development of hippocampal sclerosis (5) and SE (6).
Clarification of the mechanism underlying the E/I imbalance will provide theoretical guidance in the treatment of epilepsy. The main changes in E/I imbalance are the increase of glutamate (Glu) (7) and/or Glu receptors (8) and the decrease of GABA (9) and/or GABA type A receptors (GABAaRs) (10). Compared with change of neurotransmitter, the change of neurotransmitter receptor on cell surface is more related to seizures (11). There is ample evidence to suggest that increased glutamate (Glu) receptors (7) and/or decreased GABA type A receptors (8) (GABAaRs) on the neuron surface are key factors of E/I imbalance, which may lead to seizures. These receptors are acknowledged to be synthesized in the endoplasmic reticulum, assembled in the Golgi apparatus, and transported to the cell membrane. However, the number and location of these receptors on the cell membrane are often heterogeneous due to the characteristics of receptor subunits (12). Subunits of the receptor bind to specific proteins that help stabilize the receptor (13) in the cell membrane and facilitate internalized receptor transport to the cell membrane (14), a process called recycling.
Research has shown that kinesin superfamily proteins (KIFs) are important proteins involved in receptor recycling (15). KIFs act as microtubule-dependent intracellular transport molecular motors located in the cytoplasm. KIFs can transport various cargos, including organelles, synaptic vesicle precursors, neurotransmitter receptors, and cellular signaling molecules (16). KIF5 was the first KIF to be discovered and consists of KIF5A, KIF5B, and KIF5C. KIF5B is widely expressed in various cells, while KIF5A and KIF5C are neuron-specific (17). Additionally, KIF5A can reportedly bind to cytoplasmic cargos, helping them during transport to the cell membrane (18). KIF5A lacking the N-terminal motor domain could significantly decrease postsynaptic density protein 95 (PSD-95) level in the dendrites, which demonstrates N-terminal motor domain of KIF5A can bind to PSD-95,a pivotal postsynaptic scaffolding protein in neurons (19). Interestingly, mutations in the N-terminal motor domain of KIF5A are causative for two neurodegenerative diseases: hereditary spastic paraplegia (SPG10) and Charcot-Marie-Tooth type 2 (CMT2) (20).
Alpha-amino-3-hydroxy-5-methyl-4-Isooxazole (AMPA) receptors (AMPARs) have been established to be the main Glu receptors that mediate rapid excitatory synaptic transmission of >90% of Glu energy synapses; their number and subunit composition play an important role in their synaptic activity and plasticity (21). AMPARs are tetramers composed of glutamate receptor subunit-1 (GluR1), GluR2, GluR3, and GluR4 subunits. The AMPARs of the hippocampus and cerebral cortex are composed of GluR1/GluR2 or GluR2/GluR3 (22). AMPARs can be transported back to the membrane by KIF5A, which binds to GluR2 (18,23). Additionally, GABAaR has been identified as a key receptor for the rapid termination of seizures mediated by neuroinhibitory mechanisms (24). The subunits of GABAaRs contain 2 alpha subunits, 2 beta subunits, and 1 gamma subunit (25,26). β2 (Gabrb2) and β3 (Gabrb3) are the most abundant among the β subunits and can be re-transported to the cell membrane by binding to KIF5A (10). However, the regulatory effect of KIF5A on these 2 receptors in the E/I imbalance remains unclear.
There have been many studies on seizures. These studies have focused on changes in AMPARs/GABAaRs on the surface of cell membranes, but have not delved deeper into what causes these changes (8,10). In particular, whether recycling of AMPARs/GABAaRs is involved in seizures has not been demonstrated. To better understand the recycling of AMPARs/GABAaRs and the regulatory mechanism of KIF5A on GluR2 and Gabrb2+3 in seizures, in vivo and in vitro seizure models were used to quantify the expression of KIF5A, GluR2, and Gabrb2+3 and the interactions between KIF5A and GluR2/Gabrb2+3. We present the following article in accordance with the ARRIVE reporting checklist (available at https://atm.amegroups.com/article/view/10.21037/atm-22-4337/rc).
Methods
Animals
A total of 12 adult male Sprague-Dawley (SD) rats (weighing 200–250 g, aged 8–10 weeks), obtained from the Animal Experiment Center of Guangxi Medical University, China, were used for the experiments. The adult male SD rats were housed under the following conditions: temperature: 22–26 ℃; humidity; 50–60%; light-dark cycle: 12:12 hours (with lights on at 8:00); and rats per cage: 6. The rats had free access to food and water. Primary cultured neurons were obtained from 12 newborn SD rats (24-hour-old). Both the research team and the veterinary staff monitored animals twice daily. The minimum sample size of experimental animals was calculated by using statistical methods based on the pre-experimental data, so as to reduce the number of animals under the condition of ensuring the comparability of experimental data as much as possible. At the end of the experiment, the animals were given deep anesthesia (3% sodium pentobarbital) and then killed to alleviate their pain. Animal experiments were performed under a project license (No. 202201005) granted by The First Affiliated Hospital of Guangxi Medical University’s ethics board, in compliance with Chinese guidelines for the care and use of animals. Our protocol (including the research question, key design features, and analysis plan) was prepared before the study, and was not registered.
PTZ-induced seizure model in vivo
An intraperitoneal injection (i.p.) of pentylenetetrazol (PTZ) was administered to induce seizure in the rats. We selected a PTZ-induced seizure model in vivo that is characterized by robust, frequent spontaneous seizures, high success rate and low mortality (27). These animals recapitulate several key features of human seizures (28). The PTZ (Sigma-Aldrich, St. Louis, MO, USA) was dissolved in physiological saline before injections. The rats were divided randomly into the normal control (Ctl) group (n=6) and the PTZ-induced seizure (Sez) group (n=6). The rats in the control group were treated with physiological saline (0.9%). The rats in the Sez group received an i.p. injection of PTZ at an initial dose of 40 mg/kg. The time of PTZ injection was between 10.30 am to 12.30 am.
The seizures were classified as follows (29,30): Stage 0—No seizures; Stage 1—Ear and facial twitching; Stage 2—Strong myoclonus but no upright position; Stage 3—Strong myoclonus, upright position with bilateral forelimb clonus; Stage 4—Clonic-tonic seizures; and Stage 5—Generalized clonic-tonic seizures and loss of postural control. The rats that developed seizures > stage 4 were used for further investigations. Next, these rats were deeply anaesthetized, and their hippocampi were separated for the biochemistry experiment. in vivo model, a single animal was considered to be an experimental unit.
EEG recordings
The rats were anesthetized with isoflurane and immobilized. Electrodes were installed on the frontal, temporal, and forelimbs of the rats, and an electroencephalogram (EEG) was recorded by the Nuochen EEG system. The locations were marked as the left frontal Fp1-Avf, right frontal Fp2-Avf, left temporal T3-Avf, and right temporal T4-Avf. Criteria for seizures EEG recordings: epileptic discharges.
Mg2+-free induced seizure model in vitro
The primary neuron cultures were performed as described by Giordano et al. (31). The neurons were exposed to magnesium-free (Mg2+-free) extracellular fluid for 3 hours to establish the seizure model in vitro (32). On the 8th day of the culture, the neurons were divided into the Ctl and Mg2+-free extracellular fluid groups. The neurons in the Ctl group were exposed to a normal extracellular solution consisting of (in mM) NaCl [145], KCl [2.5], HEPES [10], CaCl2 [2], glucose [10], glycine [0.002], and MgCl2 [1]. Conversely, the neurons in the Mg2+-free extracellular fluid group were exposed to a normal extracellular solution consisting of (in mM) NaCl [145], KCl [2.5], HEPES [10], CaCl2 [2], glucose [10], and glycine [0.002] for 3 hours.
The action potentials (Aps) were obtained by whole-cell patch-clamp recording with a Digidata 1550B patch-clamp amplifier, Axon Digidata 1550B 16-bit data acquisition system, and pClamp 10.7 data acquisition software. All the recordings were performed in an extracellular recording solution containing (in mM) NaCl [122], KCl [2], HEPES [25], CaCl2 [2], MgCl2 [4], and glucose [10] with a pH of 7.4. The recordings were obtained using patch electrodes (with a resistance of 3–6 MΩ) filled with an intracellular solution containing (in mM) KCl [110], NaCl [1], EGTA [2], HEPES [25], Mg-ATP [4], Na2-GTP [0.3], and phosphocreatine [10] with a pH of 7.3. Whole-cell recordings were used, and the APs were recorded with a clamping voltage of –70 mV. Criteria for seizure model in vitro: compare to the control group, the amplitude and (or) frequency of APs were (was) significantly increased in Mg2+-free group
AMPAR-mediated miniature excitatory postsynaptic currents (AMPARs-mEPSCs) as described by Sara et al. (33). Miniature inhibitory postsynaptic currents (mIPSCs), which are mainly produced by postsynaptic GABAaRs (15), were recorded as described by Wyrembek et al. (34). All the measurements were recorded at 25℃. In vitro model, a single neuron was considered to be an experimental unit.
Western blot analysis
The total protein (35) and surface protein (plasma membrane protein) (36) of the hippocampus were extracted using the Protein Extraction Kit (Invent Biotechnologies, SD-001/SN-002; SM-005). The bicinchoninic acid (BCA) kit (Beyotime, P0012S) was used to evaluate protein concentration. Protein samples (20 µg protein/lane) were separated by sodium dodecyl sulfate-polyacrylamide gel electrophoresis and transferred to nitrocellulose (NC) membranes (Merck Millipore Ltd) by wet transfer (Bio-RAD) for western blot. The NC membranes were blocked by 5% Bovine Serum Albumin (BSA) and then incubated with a primary antibody at 4 ℃ overnight. The primary antibodies used included anti-KIF5A antibody 1:1,000 (SANTA, sc-376452), anti-GluR2 antibody 1:1,000 (BOSTER, PB9205), and anti-GABAaR β2+3 antibody 1:500 (Bioss, bs-12066R).
Next, the NC membranes were incubated with horseradish peroxidase (HRP)-conjugated Affinipure goat anti-rabbit immunoglobulin G (IgG) (H+L) 1:8,000 (Proteintech, SA00001-2) and HRP-conjugated Affinipure goat anti-mouse IgG (H+L) 1:8,000 (Proteintech, SA00001-1) at room temperature for 1 hour. After the NC films were incubated with the Omni-ECL™Femto Light Chemiluminescence Kit (EpiZyme, SQ201) for 1–2 min, the ChemiScope6000 gel imaging system was used to observe and photograph the results.
Receptor recycling assay
The cultured hippocampal neurons were incubated with GluR2 antibody 1:100 (BOSTER, PB9205) and Gabrb2+3 antibody 1:100 (Bioss, bs-12066R) for 10 min at room temperature. The cells were then washed with phosphate buffered solution (PBS) at 37 ℃ to remove the unbound antibodies and incubated for 20 min in 37 ℃ antibody-free conditioned medium to induce the internalization of antibody binding receptors. The antibodies remaining on the cell surface were stripped away by incubating in 0.5 M stripping buffer (0.5 M NaCl and 0.2 M acetic acid) on ice for 4 min (37).
Next, the neurons were washed with frozen PBS and returned to a normal medium for incubation at 37 ℃ for 90 min to promote the recycling of the receptors. After recycling, the neurons were fixed by 4% polyoxymethylene and blocked by 5% BSA. Antigen-antibody complexes that recycled to the surface were detected by incubating the cells with a red fluorescent secondary antibody (CST#8889).
The neurons were then permeabilized by 0.25%Triton X-100, and the intracellular antigen-antibody complexes were detected with a green-fluorescent secondary antibody (Bioss, BA1105). A fluorescence microscope (OLYMPUS BX53) was used to examine the results. IamgeJ software was used to analyze the images. In total, 7 cells were selected from each group to calculate the optical density. The recycling ratio was defined as the ratio of the surface fluorescence signal to the total fluorescence signal; that is, the sum of surface and intracellular signals.
Co-IP
The total native protein of the hippocampi was extracted using the Protein Extraction Kit(Invent Biotechnologies, SD-001/SN-002) (36). After protein extraction and quantification, anti-KIF5A antibody (SANTA, sc-376452) was added to lysed samples (1–2 µg per 100 µg of total protein) and incubated overnight on a head-over-head shaker at 4 ℃ to form antigen-antibody complexes. The antigen-antibody complex was incubated with agarose Protein A/G at 4 ℃ overnight. The agarose beads were washed with immunoprecipitation (IP) buffer to obtain immune precipitates, and the corresponding proteins were detected by Western blot. The primary antibodies used include anti-KIF5A antibody 1:1,000 (SANTA, sc-376452), anti-GluR2 antibody 1:1,000 (BOSTER, PB9205), and anti-GABAaR β2+3 antibody 1:500 (Bioss, bs-12066R). The IP protein band KIF5A was used to normalize the pull-down protein levels obtained from the co-IP assay. The protein levels co-precipitated between each group were then compared.
Immunofluorescence co-localization analysis
The neurons were fixed with 4% paraformaldehyde for 30 min, penetrated with 0.1% TritonX-100 for 10 min, and blocked with 5% BSA for 1 hour. The neurons were stained using a multiplex fluorescent immunohistochemistry kit (absin, abs50012) (29). The primary antibodies used included anti-KIF5A antibody 1:50 (Bioworld, BS71526), anti-GluR2 antibody 1:100 (BOSTER, PB9205), and anti-GABAaR β2+3 antibody 1:100 (Bioss, bs-12066R).
An Olympus BX53 fluorescence mirror was employed to obtain the fluorescence signals of KIF5A (green) GluR2 (red), and Gabrb2+3 (red). ImageJ plug-in was used to calculate the Pearson’s correlation coefficients (PCCs) of KIF5A/GluR2 and KIF5A/Gabrb2+3.
Statistical analysis
The data are expressed as the mean ± standard deviation (mean ± SD). An independent sample t-test was used to assess the significance of differences between the 2 groups using SPSS 25.0. A P value <0.05 was considered statistically significant.
Results
Expression of KIF5A, GluR2, and Gabrb2+3 in the PTZ-induced seizure model
No significant seizures and epileptic discharge were observed in EEG (see Figure 1A) of the Ctl group. Conversely, rats in the Sez group exhibited significant seizures of stage 4 and above. The EEG showed epileptic discharges (see Figure 1A). Compared to the control group, the total protein expression of KIF5A, GluR2, and Gabrb2+3 in the Sez group did not change significantly (see Figure 1B). In the Sez group, the expression of GluR2 on the cell surface increased significantly to 181.74%±14.44% (vs. Ctl, P=0.00). Conversely, the expression of Gabrb2+3 on the cell surface decreased to 19.62%±8.01% (vs. Ctl, P=0.00) (see Figure 1C).
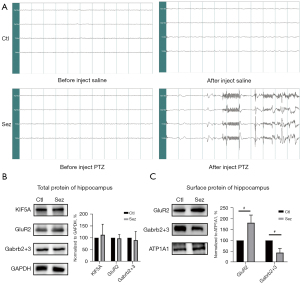
Recycling of GluR2 and Gabrb2+3 in the Mg2+-free-induced seizure model
To further confirm whether the recycling of the receptors was affected by seizures, we constructed an in vitro model of seizures and verified the success of the model by patch-clamp. The amplitude and frequency of the APs were both significantly increased in the Mg2+-free group. The amplitude of the APs was 54.39±2.76 mV in the Ctl group and 171.24±16.48 mV in the Mg2+-free group (vs. Ctl, P=0.00). The frequency of the APs was 1.50±0.79 Hz in the Ctl group and 84.10±14.44 Hz in the Mg2+-free group (vs. Ctl, P=0.00) (see Figure 2A).
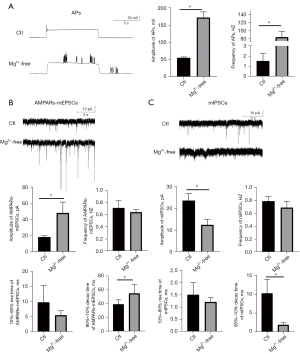
Compared to the control group, the frequency and 10–90% rise time of AMPAR-mediated mEPSC in the Mg2+-free group did not change significantly. The amplitude of AMPAR-mediated mEPSCs in the Mg2+-free group increased significantly. Specifically, the amplitude of AMPAR-mediated mEPSCs in the Ctl group was 18.19±1.74 pA and that of the Mg2+-free group was 48.18±13.51 pA (vs. Ctl, P=0.007). The 90–10% decay time of the AMPAR-mediated mEPSCs was 39.02±6.48 ms in the Ctl group and 54.72±12.61 ms in the Mg2+-free group (vs. Ctl, P=0.00) (see Figure 2B).
Compared to the control group, the frequency and 10–90% rise time of the mIPSCs in the Mg2+-free group did not change significantly. However, the amplitude of the mIPSCs in the Mg2+-free group increased significantly. Specifically, the amplitude of the mIPSCs in the Ctl group was 23.6±33.34pA, and that of the Mg2+-free group was 12.40±2.50 pA (vs. Ctl, P=0.00). The 90–10% decay time of mIPSCs was 10.19±3.69 ms in the Ctl group and 1.87±0.58 ms in the Mg2+-free group (vs. Ctl, P=0.001) (see Figure 2C).
The receptor recycling assay showed that the recycling ratio of GluR2 was 0.30±0.05 in the Ctl group and 0.60±0.07 in the Mg2+-free group (vs. Ctl, P=0.00), and the recycling ratio of Gabrb2+3 was 0.49±0.04 in the Ctl group and 0.32±0.05 in the Mg2+-free group (vs. Ctl, P=0.00) (see Figure 3).
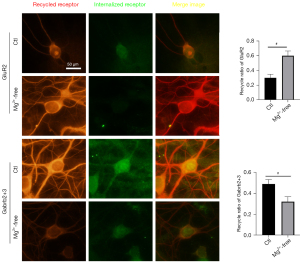
Interaction between KIF5A and GluR2 and Gabrb2+3 in the seizure model
To explore the interaction between KIF5A and GluR2/Gabrb2+3 in vivo, co-IP experiments were conducted using hippocampus lysates. The results demonstrated that KIF5A was related to GluR2 and Gabrb2+3. The pull-down protein of GluR2 in the Sez group increased to 130.42%±53.24% (vs. Ctl, P=0.046). The pull-down protein of Gabrb2+3 in the Sez group decreased to 50.86%±5.33% (vs. Ctl, P=0.00) (see Figure 4A). The immunofluorescence co-localization analysis showed that the PCC of KIF5A/GluR2 was 0.40±0.19 in the Ctl group and 0.87±0.11 (vs. Ctl, P=0.00) in the Mg2+-free solution group (see Figure 4B), and the PCC of KIF5A/Gabrb2+3 was 0.97±0.02 in the Ctl group and 0.32±0.11 in the Mg2+-free group (vs. Ctl, P=0.00) (see Figure 4C).
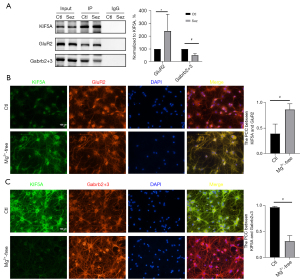
Discussion
It has been established that an E/I imbalance is the classic mechanism underlying seizures, which mainly involves strengthening the excitatory function and/or weakening the inhibitory function of neurons (3,4). There is a relationship between the function of inhibitory synapses and the number of GABAaRs on the cell surface. Notably, E/I imbalance is related to the number of AMPARs and GABAaRs on the neuron membrane. There is mounting evidence that an increased number of AMPARs (8) and decreased number of GABAaRs (10) on the surface of neurons play a pivotal role in seizures.
PTZ, an antagonist of GABAaRs, not only blocks neuronal inhibition (38), but also enhances AMPAR-mediated neuronal excitation (39). GluR2s are the key subunits of AMPARs that is responsible for excitatory neurotransmission (40). Gabrb2+3, the most abundant subunits of GABAaRs in the brain, are predominantly found in the hippocampus (41), and their deficiency decreases GABAergic inhibitory transmission (42). In the present study, we found that in seizure animal models, the amount of GluR2 on the cell membrane surface was increase, and was accompanied by a decrease in the amount of Gabrb2+3. These findings suggest that E/I imbalance is not only a change in function but also the result of the simultaneous effect of a more potent excitatory function and a weaker inhibitory function.
To further investigate whether the recycling of AMPARs and GABAaRs in neurons is involved in the pathogenesis of seizures, we constructed a seizure model in vitro, which was induced by the excitatory discharge of neurons through the Mg2+-free extracellular solution. The amplitude and frequency of the APs in the Mg2+-free group was significantly increased, which was similar to the results of Blair (43) and Gong (44).
These results also revealed an abnormal increase in neuronal excitability after treatment with Mg2+-free extracellular solution. We further investigated the AMPAR-mediated mEPSCs and mIPSCs. The frequency of micro-postsynaptic currents is mainly influenced by presynaptic neurotransmitters, while the amplitude is influenced by postsynaptic neurotransmitter receptors (45). The frequency of AMPAR-mediated mEPSCs and mIPSCs in the Mg2+-free group did not change significantly, suggesting that presynaptic mechanisms were not affected in acute seizures. An increased amplitude of AMPAR-mediated mIEPSCs results in increased postsynaptic AMPARs. A reduced amplitude of mIPSCs results in reduced post-synaptic GABAaRs. These results also demonstrated that E/I imbalance is the key factor in seizures.
AMPARs and GABAaRs have different roles; however, their dynamics in neurons are very similar. These 2 receptors are synthesized in the endoplasmic reticulum (46,47) and assembled in the Golgi apparatus (48,49). With changes in neuronal excitability, AMPARs and GABAaRs leave the postsynaptic membrane site and initiate internalization into the cytoplasm mediated by Clathrin (50,51). After entering the cytoplasm, the receptor can be recycled back to the cell membrane. The balance of the recycling of excitatory and inhibitory receptors is dynamic (52) and could be disrupted by seizures (53). Naik et al. suggested that epilepsy can cause a large number of glutamate receptors to return to the cell membrane (54).The inhibited recycling of GABAaRS may also be involved in the pathogenesis of seizures (37,42).
We found that the recycling ratio of GluR2 was increased and the recycling ratio of Gabrb2+3 was decreased. The recycling assay results suggested that recycling of neurotransmitter receptors could be affected by seizures. Accordingly, E/I imbalance may be related to enhanced AMPAR recycling and inhibited GABAaR recycling, which is also an important reason for increased AMPARs and decreased GABAaRs on the cell surface. However, the mechanisms underlying the disordered recycling of both receptors are not yet well understood.
Physiologically, a receptor that enters the cytoplasm can be degraded by lysosomes (55), or it can bind to transporters and return to the membrane along the cytoskeleton (56). The altered interaction between KIF5A and cargo in neurons is an important cause of seizures (57). KIF5A can transport a variety of substances, including AMPARs and GABAaRs. KIF5A binds to GluR2 (18) and Gabrb2+3 (10) to promote the transport of AMPARs and GABAaRs, respectively, from the cytoplasm back to the cell membrane.
We found that the expression level of KIF5A did not change significantly in the seizure model, but the interaction between KIF5A and GluR2 was significantly increased, while the interaction between KIF5A and Gabrb2+3 was decreased. These results indicate that seizures may lead to the dysregulated KIF5A-mediated transport of AMPARs/GABAaRs.
Currently, the main way to terminate seizures is to activate GABAaRs on the surface of the cell membrane through benzodiazepines (58). Whereas, benzodiazepines only terminated seizures in 43–89% of patients, and some patients did not achieve therapeutic effect (59). There are two main reasons for the ineffectiveness of benzodiazepines: (I) decreased number of GABAaRs (10) on the surface of neurons, resulting in no target for benzodiazepines; (II) increased number of AMPARs on surface of neurons (8), causing neurons to fire continuously. Therefore, increasing GABAaRs and decreasing the number of AMPARs on the cell membrane is an important treatment for seizure termination. Our study revealed the changes of receptors recycling mechanism in seizures. Based on our results, we believe that enhancing the recycling of GABAaRs and inhibiting the recycling of AMPARs may be an important method to terminate epileptic seizures, and KIF5A may play a key role.
However, this study did not explore the underlying mechanism of abnormal binding of KIF5A to GluR2/Gabrb2+3, which has been reported to be related to some connexins, such as glutamate receptor-interacting protein (60) and GABA receptor-associated protein (61), which are mainly responsible for connecting KIF5A and the receptor, and acting as intermediaries. Accordingly, abnormalities in their structure can affect the transport of KIF5A to the receptor. The role of these proteins in the pathogenesis of seizures will be the focus of our future studies.
Acknowledgments
We thank Home for Researchers’ editorial team (www.home-for-researchers.com) for language editing service.
Funding: This work was supported by Natural Science Foundation of Guangxi Province (No. 2020GXNSFDA297012).
Footnote
Reporting Checklist: The authors have completed the ARRIVE reporting checklist. Available at https://atm.amegroups.com/article/view/10.21037/atm-22-4337/rc
Data Sharing Statement: Available at https://atm.amegroups.com/article/view/10.21037/atm-22-4337/dss
Conflicts of Interest: All authors have completed the ICMJE uniform disclosure form (available at https://atm.amegroups.com/article/view/10.21037/atm-22-4337/coif). The authors have no conflicts of interest to declare.
Ethical Statement: The authors are accountable for all aspects of the work in ensuring that questions related to the accuracy or integrity of any part of the work are appropriately investigated and resolved. Animal experiments were performed under a project license (No. 202201005) granted by The First Affiliated Hospital of Guangxi Medical University’s ethics board, in compliance with Chinese guidelines for the care and use of animals.
Open Access Statement: This is an Open Access article distributed in accordance with the Creative Commons Attribution-NonCommercial-NoDerivs 4.0 International License (CC BY-NC-ND 4.0), which permits the non-commercial replication and distribution of the article with the strict proviso that no changes or edits are made and the original work is properly cited (including links to both the formal publication through the relevant DOI and the license). See: https://creativecommons.org/licenses/by-nc-nd/4.0/.
References
- Betjemann JP, Lowenstein DH. Status epilepticus in adults. Lancet Neurol 2015;14:615-24. [Crossref] [PubMed]
- Mátyás A, Borbély E, Mihály A. Hippocampal Sclerosis in Pilocarpine Epilepsy: Survival of Peptide-Containing Neurons and Learning and Memory Disturbances in the Adult NMRI Strain Mouse. Int J Mol Sci 2021;23:204. [Crossref] [PubMed]
- Kapur J, Coulter DA. Experimental status epilepticus alters gamma-aminobutyric acid type A receptor function in CA1 pyramidal neurons. Ann Neurol 1995;38:893-900. [Crossref] [PubMed]
- Michelson HB, Kapur J, Lothman EW. Reduction of paired pulse inhibition in the CA1 region of the hippocampus by pilocarpine in naive and in amygdala-kindled rats. Exp Neurol 1989;104:264-71. [Crossref] [PubMed]
- Walker MC. Hippocampal Sclerosis: Causes and Prevention. Semin Neurol 2015;35:193-200. [Crossref] [PubMed]
- Moshé SL, Perucca E, Ryvlin P, et al. Epilepsy: new advances. Lancet 2015;385:884-98. [Crossref] [PubMed]
- Deng Y, Wang M, Wang W, et al. Comparison and effects of acute lamotrigine treatment on extracellular excitatory amino acids in the hippocampus of PTZ-kindled epileptic and PTZ-induced status epilepticus rats. Neurochem Res 2013;38:504-11. [Crossref] [PubMed]
- Wasterlain CG, Naylor DE, Liu H, et al. Trafficking of NMDA receptors during status epilepticus: therapeutic implications. Epilepsia 2013;54:78-80. [Crossref] [PubMed]
- Wasterlain CG, Baxter CF, Baldwin RA. GABA metabolism in the substantia nigra, cortex, and hippocampus during status epilepticus. Neurochem Res 1993;18:527-32. [Crossref] [PubMed]
- Goodkin HP, Joshi S, Mtchedlishvili Z, et al. Subunit-specific trafficking of GABA(A) receptors during status epilepticus. J Neurosci 2008;28:2527-38. [Crossref] [PubMed]
- Mo J, Wang B, Zhu X, et al. PRRT2 deficiency induces paroxysmal kinesigenic dyskinesia by influencing synaptic function in the primary motor cortex of rats. Neurobiol Dis 2019;121:274-85. [Crossref] [PubMed]
- Nathanson AJ, Zhang Y, Smalley JL, et al. Identification of a Core Amino Acid Motif within the α Subunit of GABAARs that Promotes Inhibitory Synaptogenesis and Resilience to Seizures. Cell Rep 2019;28:670-681.e8. [Crossref] [PubMed]
- Hines RM, Maric HM, Hines DJ, et al. Developmental seizures and mortality result from reducing GABAA receptor α2-subunit interaction with collybistin. Nat Commun 2018;9:3130. [Crossref] [PubMed]
- Umanah GKE, Ghasemi M, Yin X, et al. AMPA Receptor Surface Expression Is Regulated by S-Nitrosylation of Thorase and Transnitrosylation of NSF. Cell Rep 2020;33:108329. [Crossref] [PubMed]
- Nakajima K, Yin X, Takei Y, et al. Molecular motor KIF5A is essential for GABA(A) receptor transport, and KIF5A deletion causes epilepsy. Neuron 2012;76:945-61. [Crossref] [PubMed]
- Hirokawa N, Noda Y. Intracellular transport and kinesin superfamily proteins, KIFs: structure, function, and dynamics. Physiol Rev 2008;88:1089-118. [Crossref] [PubMed]
- Fukuda Y, Pazyra-Murphy MF, Silagi ES, et al. Binding and transport of SFPQ-RNA granules by KIF5A/KLC1 motors promotes axon survival. J Cell Biol 2021;220:e202005051. [Crossref] [PubMed]
- Setou M, Seog DH, Tanaka Y, et al. Glutamate-receptor-interacting protein GRIP1 directly steers kinesin to dendrites. Nature 2002;417:83-7. [Crossref] [PubMed]
- Yoo KS, Lee K, Oh JY, et al. Postsynaptic density protein 95 (PSD-95) is transported by KIF5 to dendritic regions. Mol Brain 2019;12:97. [Crossref] [PubMed]
- Nicolas A, Kenna KP, Renton AE, et al. Genome-wide Analyses Identify KIF5A as a Novel ALS Gene. Neuron 2018;97:1268-1283.e6. [Crossref] [PubMed]
- Traynelis SF, Wollmuth LP, McBain CJ, et al. Glutamate receptor ion channels: structure, regulation, and function. Pharmacol Rev 2010;62:405-96. [Crossref] [PubMed]
- Schwenk J, Baehrens D, Haupt A, et al. Regional diversity and developmental dynamics of the AMPA-receptor proteome in the mammalian brain. Neuron 2014;84:41-54. [Crossref] [PubMed]
- Brachet A, Lario A, Fernández-Rodrigo A, et al. A kinesin 1-protrudin complex mediates AMPA receptor synaptic removal during long-term depression. Cell Rep 2021;36:109499. [Crossref] [PubMed]
- Raol YH, Lund IV, Bandyopadhyay S, et al. Enhancing GABA(A) receptor alpha 1 subunit levels in hippocampal dentate gyrus inhibits epilepsy development in an animal model of temporal lobe epilepsy. J Neurosci 2006;26:11342-6. [Crossref] [PubMed]
- Chang Y, Wang R, Barot S, et al. Stoichiometry of a recombinant GABAA receptor. J Neurosci 1996;16:5415-24. [Crossref] [PubMed]
- Tretter V, Ehya N, Fuchs K, et al. Stoichiometry and assembly of a recombinant GABAA receptor subtype. J Neurosci 1997;17:2728-37. [Crossref] [PubMed]
- Mareš P, Kubová H. Interaction of GABAA and GABAB antagonists after status epilepticus in immature rats. Epilepsy Behav 2020;102:106683. [Crossref] [PubMed]
- Mason CR, Cooper RM. A permanent change in convulsive threshold in normal and brain-damaged rats with repeated small doses of pentylenetetrazol. Epilepsia 1972;13:663-74. [Crossref] [PubMed]
- Racine R, Okujava V, Chipashvili S. Modification of seizure activity by electrical stimulation. 3. Mechanisms. Electroencephalogr Clin Neurophysiol 1972;32:295-9. [Crossref] [PubMed]
- Becker A, Grecksch G, Rüthrich HL, et al. Kindling and its consequences on learning in rats. Behav Neural Biol 1992;57:37-43. [Crossref] [PubMed]
- Giordano G, Costa LG. Primary neurons in culture and neuronal cell lines for in vitro neurotoxicological studies. Methods Mol Biol 2011;758:13-27. [Crossref] [PubMed]
- Goodkin HP, Yeh JL, Kapur J. Status epilepticus increases the intracellular accumulation of GABAA receptors. J Neurosci 2005;25:5511-20. [Crossref] [PubMed]
- Sara Y, Bal M, Adachi M, et al. Use-dependent AMPA receptor block reveals segregation of spontaneous and evoked glutamatergic neurotransmission. J Neurosci 2011;31:5378-82. [Crossref] [PubMed]
- Wyrembek P, Lebida K, Mercik K, et al. Block and allosteric modulation of GABAergic currents by oenanthotoxin in rat cultured hippocampal neurons. Br J Pharmacol 2010;160:1302-15. [Crossref] [PubMed]
- Zhang SH, Liu DX, Wang L, et al. A CASPR1-ATP1B3 protein interaction modulates plasma membrane localization of Na+/K+-ATPase in brain microvascular endothelial cells. J Biol Chem 2019;294:6375-86. [Crossref] [PubMed]
- Hendrix RD, Ou Y, Davis JE, et al. Alzheimer amyloid-β- peptide disrupts membrane localization of glucose transporter 1 in astrocytes: implications for glucose levels in brain and blood. Neurobiol Aging 2021;97:73-88. [Crossref] [PubMed]
- Mele M, Aspromonte MC, Duarte CB. Downregulation of GABAA Receptor Recycling Mediated by HAP1 Contributes to Neuronal Death in In Vitro Brain Ischemia. Mol Neurobiol 2017;54:45-57. [Crossref] [PubMed]
- Emami S, Valipour M, Kazemi Komishani F, et al. Synthesis, in silico, in vitro and in vivo evaluations of isatin aroylhydrazones as highly potent anticonvulsant agents. Bioorg Chem 2021;112:104943. [Crossref] [PubMed]
- Kovalenko AA, Zakharova MV, Zubareva OE, et al. Alterations in mRNA and Protein Expression of Glutamate Receptor Subunits Following Pentylenetetrazole-induced Acute Seizures in Young Rats. Neuroscience 2021;468:1-15. [Crossref] [PubMed]
- Jean WH, Huang CT, Hsu JH, et al. Anticonvulsive and Neuroprotective Effects of Eupafolin in Rats Are Associated with the Inhibition of Glutamate Overexcitation and Upregulation of the Wnt/β-Catenin Signaling Pathway. ACS Chem Neurosci 2022;13:1594-603. [Crossref] [PubMed]
- Persohn E, Malherbe P, Richards JG. Comparative molecular neuroanatomy of cloned GABAA receptor subunits in the rat CNS. J Comp Neurol 1992;326:193-216. [Crossref] [PubMed]
- Li R, Wu B, He M, et al. HAP1 Modulates Epileptic Seizures by Regulating GABAAR Function in Patients with Temporal Lobe Epilepsy and in the PTZ-Induced Epileptic Model. Neurochem Res 2020;45:1997-2008. [Crossref] [PubMed]
- Blair RE, Sombati S, Lawrence DC, et al. Epileptogenesis causes acute and chronic increases in GABAA receptor endocytosis that contributes to the induction and maintenance of seizures in the hippocampal culture model of acquired epilepsy. J Pharmacol Exp Ther 2004;310:871-80. [Crossref] [PubMed]
- Gong L, Han Y, Chen R, et al. LncRNA ZNF883-Mediated NLRP3 Inflammasome Activation and Epilepsy Development Involve USP47 Upregulation. Mol Neurobiol 2022;59:5207-21. [Crossref] [PubMed]
- Li C, Li Y, Zhao Z, et al. Aerobic exercise regulates synaptic transmission and reactive oxygen species production in the paraventricular nucleus of spontaneously hypertensive rats. Brain Res 2019;1712:82-92. [Crossref] [PubMed]
- Greger IH, Khatri L, Ziff EB. RNA editing at arg607 controls AMPA receptor exit from the endoplasmic reticulum. Neuron 2002;34:759-72. [Crossref] [PubMed]
- Saliba RS, Pangalos M, Moss SJ. The ubiquitin-like protein Plic-1 enhances the membrane insertion of GABAA receptors by increasing their stability within the endoplasmic reticulum. J Biol Chem 2008;283:18538-44. [Crossref] [PubMed]
- Rathenberg J, Kittler JT, Moss SJ. Palmitoylation regulates the clustering and cell surface stability of GABAA receptors. Mol Cell Neurosci 2004;26:251-7. [Crossref] [PubMed]
- Waites CL, Specht CG, Härtel K, et al. Synaptic SAP97 isoforms regulate AMPA receptor dynamics and access to presynaptic glutamate. J Neurosci 2009;29:4332-45. [Crossref] [PubMed]
- Carroll RC, Beattie EC, von Zastrow M, et al. Role of AMPA receptor endocytosis in synaptic plasticity. Nat Rev Neurosci 2001;2:315-24. [Crossref] [PubMed]
- Kanematsu T, Fujii M, Mizokami A, et al. Phospholipase C-related inactive protein is implicated in the constitutive internalization of GABAA receptors mediated by clathrin and AP2 adaptor complex. J Neurochem 2007;101:898-905. [Crossref] [PubMed]
- Xiao MY, Gustafsson B, Niu YP. Metabotropic glutamate receptors in the trafficking of ionotropic glutamate and GABA(A) receptors at central synapses. Curr Neuropharmacol 2006;4:77-86. [Crossref] [PubMed]
- Zhu J, Lee KY, Jewett KA, et al. Epilepsy-associated gene Nedd4-2 mediates neuronal activity and seizure susceptibility through AMPA receptors. PLoS Genet 2017;13:e1006634. [Crossref] [PubMed]
- Naik AA, Sun H, Williams CL, et al. Mechanism of seizure-induced retrograde amnesia. Prog Neurobiol 2021;200:101984. [Crossref] [PubMed]
- Kittler JT, Chen G, Honing S, et al. Phospho-dependent binding of the clathrin AP2 adaptor complex to GABAA receptors regulates the efficacy of inhibitory synaptic transmission. Proc Natl Acad Sci U S A 2005;102:14871-6. [Crossref] [PubMed]
- Xu X, Shangguan Y, Lu S, et al. Tubulin β-III modulates seizure activity in epilepsy. J Pathol 2017;242:297-308. [Crossref] [PubMed]
- Liu M, Pi H, Xi Y, et al. KIF5A-dependent axonal transport deficiency disrupts autophagic flux in trimethyltin chloride-induced neurotoxicity. Autophagy 2021;17:903-24. [Crossref] [PubMed]
- Jones-Davis DM, Macdonald RL. GABA(A) receptor function and pharmacology in epilepsy and status epilepticus. Curr Opin Pharmacol 2003;3:12-8. [Crossref] [PubMed]
- Alldredge BK, Gelb AM, Isaacs SM, et al. A comparison of lorazepam, diazepam, and placebo for the treatment of out-of-hospital status epilepticus. N Engl J Med 2001;345:631-7. [Crossref] [PubMed]
- Mao L, Takamiya K, Thomas G, et al. GRIP1 and 2 regulate activity-dependent AMPA receptor recycling via exocyst complex interactions. Proc Natl Acad Sci U S A 2010;107:19038-43. [Crossref] [PubMed]
- Khundakar AA, Hanson PS, Erskine D, et al. Analysis of primary visual cortex in dementia with Lewy bodies indicates GABAergic involvement associated with recurrent complex visual hallucinations. Acta Neuropathol Commun 2016;4:66. [Crossref] [PubMed]
(English Language Editor: L. Huleatt)