The silencing of SAAL1 suppresses pneumonia progression via modulating the NLR signaling pathway
Introduction
Pneumonia is one of the most frequently occurring infections in both children and elderly people (1,2). In recent years, pneumonia has become a severe infectious disease and is a leading cause of morbidity and mortality (3,4). The pathogenesis correlated with pneumonia is mainly pathogen invasion, including viruses, fungi, and bacteria, which causes a series of clinical symptoms, such as cough, fever, uneven breath, chest pain, and sometimes pulmonary failure and heart failure (5). When an infection with a pathogenic microorganism happens, the immune system of the host is activated, inflammation occurs, followed by the accumulation of inflammatory factors and neutrophils (6). In recent decades, increasing numbers of studies have discussed the molecular mechanisms underlying pneumonia (7-9). Regulatory factors, including cytokines, micro RNAs, and long non-coding RNAs are widely reported to be critical participants of pneumonia (10). However, no effective treatment is currently available to alleviate the damages caused by pneumonia; thus, novel therapies for pneumonia-associated symptoms urgently need to be explored.
Inflammation is the physiological response of organs and cells to various stimuli, including pathogens and antigens (11). An abnormal inflammatory response could lead to severe damages in major organs in the human body (12,13). Higher levels of inflammatory factors, such as tumor necrosis factor alpha (TNF-α) and interleukin-6 (IL-6), were observed in the blood and lung tissues of patients with pneumonia, and thus are regarded as clinical biomarkers (14). Studies have indicated that aged people with a higher concentration of inflammatory factors in lung tissues tend to suffer from pneumonia after years of accumulation (15,16). The inflammatory stimulation by microorganisms, such as endotoxin, is closely correlated with pneumonia (17,18). Lipopolysaccharide (LPS), the major component of cell wall of Gram-negative (G–) bacterium, is a potential active endotoxin that stimulates the inflammatory response (19,20). Numerous studies have used LPS as the stimulator to establish chronic inflammatory diseases, including pneumonia, in clinical and basic studies (21,22).
The gene of serum amyloid A-like 1 (SAAL1), localized to chromosome 11p15.1, consists of 12 exons and encodes a protein with 474 amino acids (23,24). SAAL1 is mainly distributed in the nucleus (25). As a newly reported acute phase responsive protein, SAAL1 participates in the abnormal activation and proliferation of synovial fibroblasts during synovitis occurrence (25). Currently, very few studies (in-depth and in in general) have been conducted on SAAL1. In a rheumatoid arthritis model, SAAL1 deficiency led to a delayed onset and the progression of collagen-induced arthritis, by downregulating the cell cycle-related CDK6 gene at the post-transcriptional level (26). A recent study defined SAAL1 as an oncogenic gene, SAAL1 was highly expressed in hepatocellular carcinoma and mainly acted by modulating inflammation genes (27). However, the expression and function of SAAL1 in pneumonia has not yet been elucidated.
In this study, we analyzed a data set from the Gene Expression Omnibus (GEO) database to determine the expression profile of SAAL1 in the LPS-stimulate rat model, screened the potential regulatory pathways, and determined the effects of SAAL1 depletion on the inflammatory response of LPS-stimulate mice. We present the following article in accordance with the ARRIVE reporting checklist (available at https://atm.amegroups.com/article/view/10.21037/atm-22-4013/rc).
Methods
Public database analysis
The GSE111242 data set was downloaded from the GEO (https://www.ncbi.nlm.nih.gov/geo/). This data set contains the information of 12 bronchoalveolar lavage fluid (BALF) samples from an LPS-stimulated lung injury rat model and was analyzed using the GPL1355 [Rat230_2] Affymetrix Rat Genome 230 2.0 Array.
Mouse model
Male BALB/c mice (six-week-old, 16–20 g, n=20) were brought from Jinan Pengyue experimental animal breeding Co., Ltd (China) and housed in a standard environment with free access to water and food. Mice were randomly divided into control, LPS, shNC + LPS, and sh-SAAL1 + LPS group. For random grouping, the randomization was generated using a table of random numbers. Through literature review, 5 mice were included in each group. All the research protocols in this study were approved by the Animal Care and Use Committee of Zhejiang University of Traditional Chinese Medicine (No. 2021-05-20), in compliance with institutional guidelines for the care and use of animals. A protocol is prepared before the study without registration. To establish the pneumonia model, the mice were fasted overnight and anesthetized. Next, LPS (5 mg/kg; Sigma, USA) was intratracheally administrated. The mice in the control group received an intratracheal injection of the same volume of phosphate buffered solution (PBS). For the short-hairpin RNA (shRNA) treatment, the lentivirus that contained sh-negative control (NC) and sh-SAAL1 were injected 2 days before the administration of LPS. The BALF was collected 3 days after LPS stimulation by instilling ice-cold PBS (0.5 mL) into the lungs 3 times. The lung tissues were collected and weighed and the wet/dry (W/D) weight ratios of the lungs were calculated. Myeloperoxidase (MPO) activity was assessed using the MPO Activity Assay Kit (ab105136, Abcam, USA) in accordance with the manufacturer’s instructions.
Histological analysis
The lung tissues collected from the mice were fixed in 4% formaldehyde for 48 hours, dehydrated and embedded in paraffin. Next, 5 µm-thick slices were made and processed with hematoxylin and eosin (H&E) staining. Images were then taken under a light microscope (Leica, Germany). Pathological changes were assessed by 2 blinded investigators.
Immunohistochemistry analysis
The paraffine-embedded samples were rehydrated with serial ethanol, antigen retrieval, and blockade with goat serum at room temperature for 30 minutes, and then incubated with anti-SAAL1 antibody overnight at 4 ℃. The next day, the samples were washed with PBS and hatched with 3,3N-Diaminobenzidine Tertrahydrochloride (DAB) reagent (P0202, Beyotime, China) for visualization. Images were taken under a light microscope.
Cell line and treatment
Human lung cancer cell line A549 was purchased from American Type Culture Collection (ATCC, USA), and maintained in Dulbecco’s modified Eagle’s medium (Thermo, USA) containing 10% fetal bovine serum (FBS; Gibco, USA) and 1% penicillin/streptomycin (Gibco, USA) in a 37 ℃ incubator filled with 5% carbon dioxide. To establish the in vitro injury model, the cells were placed in 6-well plates (3×105 per well) and incubated for 12 hours, and then incubated with LPS (10 µg/mL) for 24 hours.
Cell transfection
The small interfering RNA (si)-SAAL1-1, si-SAAL1-2, si- negative control (NC), and nucleotide-binding and oligomerization domain (NOD)-like receptor (NLR) family pyrin domain containing 3 (NLRP3) overexpressing vectors (pCMV-NLRP3), and the empty vectors were synthesized by GenePharma (Shanghai). The cells were seeded into 6-well plate at a density of 3×105 cells/well, then Lipofectamine 3000 reagent (Invitrogen, USA) was used to transfect si-NC, si-SAAL1-1, si-SAAL1-2, pCMV-NLRP3, and the empty vectors into cells. After transfection for 48 hours, the cells were taken to perform assays (see below).
Cell apoptosis
The apoptosis of the A549 cells was checked using an annexin V apoptosis kit (CA1020, Solarbio, China) in accordance with the manufacturer’s protocol. In short, the cells were collected after the indicated treatment, and re-suspended in binding buffer. Next, cells were treated with annexin V-fluorescein Isothiocyanate (FITC) and propidium iodide (PI) reagent in the dark for 20 minutes. The samples were immediately analyzed by flow cytometry.
Cell viability assays
The Cell Counting Kit-8 (CCK-8; C0037, Beyotime, China) was used to measure cell viability. The cells were seeded into a 96-well plate (5×103/well), after the indicated treatment, and 20 µL of CCK-8 reagent was added to each well, and the cells were then incubated for another 4 hours. Next, the cells were incubated with dimethyl sulfoxide. The optical density at 490 nm was checked using a spectrophotometer.
Western blotting assays
The tissues and cells were lysed in ice-cold radio-immunoprecipitation assay buffer to extract the total proteins. The proteins were separated using sodium dodecyl sulfate–polyacrylamide gel electrophoresis, and shifted to polyvinylidene fluoride membranes. After blocking in 5% non-fat milk for 1 hour at room temperature, the membranes were probed with primary antibodies against SAAL1 (PA5-83445, Invitrogen, USA), TNF-α (ab183218, Abcam, USA), IL-6 (ab259341, Abcam, USA), IL-1β (ab254360, Abcam, USA), NLRP3 (ab263899, Abcam, USA), NOD1 (ab217798, Abcam, USA), mitogen-activated protein kinase 1 (MAPK 1) (ab170099, Abcam, USA), marker of proliferation Ki-67 (Ki67) (ab15580, Abcam, USA), caspase-1 (ab16883, Abcam, USA), Bcl2 associated X protein (Bax) (ab32503, Abcam, USA), B-cell lymphoma-2 (Bcl-2) (ab182858, Abcam, USA), glyceraldehyde 3-phosphate dehydrogenase (GAPDH; ab8245, Abcam, USA), and corresponding secondary anti-mouse (ab205719, Abcam, USA), and anti-rabbit (ab205718, Abcam, USA) antibodies. The visualization of the targeted protein bands was realized by reaction with Enhanced chemiluminescence reagent (Millipore, Germany).
qRT-PCR
The messenger RNA levels of SAAL1 were determined by quantitative real-time polymerase chain reaction (qRT-PCR) assays. The total RNA was extracted using Trizol reagent (Invitrogen, USA), and transcribed to complementary DNAs using a first-strand synthesis kit (Transgen, China). Next, quantification was performed with Synergy Brands Synergy Brands, Inc. (SYBR) Green (Thermo, USA) using the 2-ΔΔCt method. GAPDH was used as the internal control.
ELISA
The production of TNF-α, IL-6, and IL-1β was evaluated using a commercial enzyme-linked immunosorbent assay (ELISA) kit (Peprotech, USA) in accordance with the manufacturer’s instruction. In brief, the lung tissues collected from the mice were homogenized, and centrifuged. Next, the supernatant was collected and added into 96-well plates pre-coated with antibodies against the TNF-α, IL-6, and IL-1β. After incubation at 37 ℃, the absorbance values were measured by a microplate detector. To detect the inflammatory cytokines secreted by the A549 cells, the cell culture medium was collected and analyzed by kits after centrifuge to remove the debris.
Statistical analysis
The data in this work are presented as the mean ± standard error of the mean and analyzed using SPSS 19.0. Comparisons between 2 or more groups were performed using Student’s t test or a one-way analysis-of-variance test, followed by Tukey’s post-hoc test. A P value <0.05 was considered statistically significant.
Results
SAAL1 was upregulated in a mouse pneumonia model
To determine the function of SAAL1 in pneumonia pathogenesis, we first analyzed the expression of SAAL1 using the GEO data. As Figure 1A shows, the RNA level of SAAL1 in the BALF collected from the LPS-stimulated rat model was significantly elevated compared to that of the control group. We next established the LPS-stimulated mouse model to mimic pneumonia and analyzed SAAL1 expression. Consistent with the results from the GEO database, the level of SAAL1 in lung tissues of the LPS-stimulated mouse model was significantly higher than that of the control group (see Figure 1B). The results of the immunohistochemistry analysis and western blotting revealed significantly elevated protein levels of SAAL1 in the lung tissues after LPS stimulation (see Figure 1C,1D).
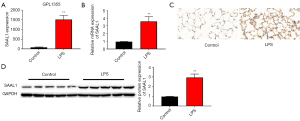
The silencing of SAAL1 ameliorated LPS-induced lung injury and inflammation in the mouse model
We next examined whether the silencing of SAAL1 affected LPS-induced pneumonia. Treatment with sh-SAAL1 significantly suppressed the LPS-induced elevation of SAAL1 expression (see Figure 2A). LPS stimulation caused injury (see Figure 2B) and edema (see Figure 2C) in the mice lung tissues, while SAAL1 depletion alleviated this phenomenon. LPS also stimulated the neutrophil accumulation and MPO activation, compared to the control group, which were suppressed by sh-SAAL1 (see Figure 2D,2E). Additionally, the LPS-induced production of TNF-α, IL-6, and IL-1β was abolished by SAAL1 knockdown (see Figure 2F-2I).
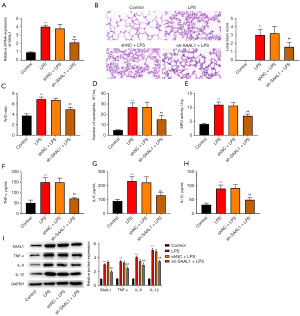
Silencing of SAAL1 suppressed NLR signaling
We next performed a Gene Set Enrichment Analysis (GSEA) of the lung tissue data from the obtained GEO data set. The samples were divided into the SAAL1-high and SAAL1-low groups, and the differentially expressed genes were then analyzed. The results indicated that the differently expressed genes between the 2 groups were mainly enriched in the NLR pathway (see Figure 3A). Western blotting assays were then conducted, and we observed that LPS increased the expression of NLRP3, NOD1, and MAPK1 (see Figure 3B), which are the critical regulators of the NLR pathway, while the depletion of SAAL1 inactivated this signal transduction. These data suggest that SAAL1 might promote the inflammatory response of LPS-induced pneumonia by modulating the NLR pathway.
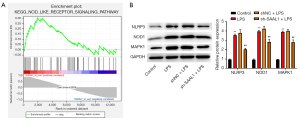
The silencing of SAAL1 alleviated LPS-induced cell apoptosis and inflammation in vitro
Subsequently, we checked the function of SAAL1 depletion in vitro. As Figure 4A shows, stimulation with LPS increased the level of SAAL1 in the A549 lung cells in a dose-dependent manner. Transfection with si-SAAL1 effectively suppressed SAAL1 expression in the lung cells (see Figure 4B). LPS treatment suppressed lung cell viability and enhanced cell apoptosis, while SAAL1 knockdown reversed these effects (see Figure 4C,4D). Additionally, LPS suppressed the protein levels of the proliferative biomarker Ki67 and anti-apoptotic Bcl-2 and elevated the expression of pro-apoptotic caspase-1 and Bax (see Figure 4E), while the depletion of SAAL1 abolished the altered protein levels.
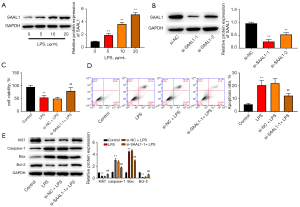
Further, the depletion of SAAL1 suppressed the LPS-induced production of inflammatory factors TNF-α (see Figure 5A), IL-6 (see Figure 5B), and IL-1β (see Figure 5C) in the A549 cells. Consistent with the results from the mouse model, LPS also induced the elevation of the NLR signaling pathway, including the protein levels of NLRP3, NOD1, and MAPK1 (see Figure 5D), and SAAL1 depletion suppressed the activation of this pathway.
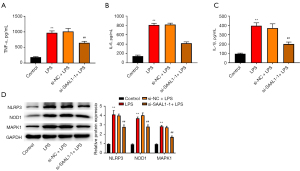
The silencing of SAAL1 alleviated LPS-induced cell apoptosis by modulating the NLR signaling pathway
To determine the involvement of the NLR signaling pathway in SAAL1-modulated pneumonia, we performed the overexpression of NLRP3 in the LPS-stimulated cells. Transfection with NLRP3 overexpressing vectors effectively elevated the level of NLRP3 in lung cells (see Figure 6A). As Figure 6B,6C show, the improved cell viability and decreased cell apoptosis caused by si-SAAL1-1 were abolished by the overexpression of NLRP3 in the LPS-treated A549 cells. Additionally, the deceased production of TNF-α, IL-6, and IL-1β by si-SAAL1 in the LPS-stimulated cells was significantly upregulated by NLRP3 overexpression (see Figure 6D-6F).
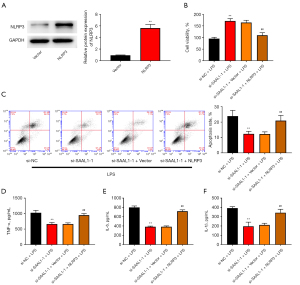
Discussion
Pneumonia is a complicated disorder of the respiratory system that affects the health of a certain number of people worldwide and has become an economic burden (28). Thus, understanding the mechanisms of pneumonia and looking for a safe and effective therapy for pneumonia is urgent. In this study, we examined the effects of SAAL1 in pathogenesis of pneumonia using LPS-stimulated in vitro and in vivo models.
One of the most common consequences of pneumonia is lung injury, which includes inflammatory damage to the alveolar capillary and the accumulation of inflammatory cytokines (29,30). Thus, the abnormal inflammatory response plays a critical role in pneumonia development (31,32). The LPS stimulation is a well-accepted and reliable experimental approach for clinical studies of pneumonia, and is thus widely applied to the exploration of pathological mechanisms (19,21). LPS stimulation is capable of activating the inflammatory cascades, such as TNF-α and IL-6 (33,34). TNF-α and IL-6 are representative inflammatory cytokines that participate in the progression of various diseases, such as diabetes, sepsis, cardiovascular diseases, fibrosis, and cancers (35-38). The abnormal serum levels of IL-10, IL-6, IL-1β, and TNF-α are proposed as potential diagnostic biomarkers for community-acquired pneumonia and coronavirus disease infection (39-41). The administration of LPS elevated the expression of SAAL1 in lung cells, and decreased cell proliferation, enhanced cell apoptosis, and activated inflammation. Conversely, the silencing of SAAL1 abolished the adverse effects of LPS in lung cells. Additionally, the lung injury caused by pneumonia led to neutrophil accumulation and pulmonary edema, which were also alleviated by the knockdown of SAAL1.
SAAL1 is a newly spotted acute phase responsive protein that is correlated with abnormal activation of synovial fibroblasts and synovitis development (25). The knockdown of SAAL1 could suppress synovial fibroblasts proliferation to alleviate rheumatoid arthritis (25). As an acute-phase protein, SAAL1 is upregulated in the liver and blood when bacterial infection and inflammation occurs (24). This may explain the elevated expression of SAAL1 in the lung tissues and cells upon LPS stimulation.
The results of our bioinformatics analysis showed that the knockdown of SAAL1 in LPS-stimulated lung tissues induced the alteration of the NLR signaling pathway. NLRs are a family of pattern recognition receptors that play a critical role in innate immunity by recognizing diverse microbial motifs (42). NLRs trigger the innate immune signaling by stimulating the downstream cascades including cytokines, chemokines, interferons, and antimicrobial peptides (42,43). Studies have revealed that LPS could activate the expression of NOD1 and NOD2 (44,45). NOD1 is a member of intracellular pattern-recognition receptors and initiates the inflammatory response to cell wall molecules, such as diaminopimelic acid from bacteria during innate immune responses (46). The detection of gram-negative bacterial peptidoglycan stimulated the NOD1 signaling to activate autophagy and inflammation of host cells (47). The knockdown of NOD1 leads to the impaired recruitment of neutrophils to alveolar tissue (48,49). In the NLR family, NLRP3 inflammasome is a well-studied and critical inflammasome, which causes the release of proinflammatory cytokines IL-1β and IL-6, upon activation by pathogen-associated molecular patterns and damage-associated molecular patterns, such as viral and pathogenic bacterial infections (50). Targeting NLRP3 is a potential and promising treatment for inflammation-related lung and gut diseases (51,52). We found that LPS stimulation induced the expression of NOD1 and NLRP3, and SAAL1 knockdown downregulated their expression. Mechanistically, the overexpression of NLRP3 abolished the protective effects of SAAL1 depletion against injury and inflammation stimulated by LPS. Taken together, our study might provide new understanding for pneumonia mechanism and provide potential therapeutic strategies for pneumonia.
However, there are some limitations in our study. First, we performed rescue experiments (co-transfection of si-SAAL1-1 and pCMV-NLRP3) to investigate the relationship between SAAL1 and NLR signaling. Increasing the inhibitor or agonist of signaling pathway may be more conducive to support the conclusions of this study. Second, we used CCK-8 assay and flow cytometry to detect cell viability and apoptosis. Using multiple methods to detect cell viability and apoptosis will be led to more reliable results. Third, we only detected the change of IL-1β and IL-6, and TNF-α. Detecting multiple inflammatory factors may make the results more credible. Finally, increasing the experimental study on the regulation of SAAL1 by lncRNA or miRNA will make the mechanism of SAAL1 in pneumonia more complete. In future studies, we will conduct more assays to further improve our study.
Conclusions
To summarize, the analysis of data from the GEO database and the LPS-stimulated mouse model revealed that SAAL1 was upregulated in the pneumonia model. The knockdown of SAAL1 alleviated the injury, edema, and inflammation in the lung tissues of the LPS-treated mice, and the knockdown of SAAL1 increased cell proliferation and decreased cell apoptosis and inflammation in the LPS-treated cells. A study of the regulatory mechanisms revealed the inactivation of NLR signaling on SAAL1 knockdown.
Acknowledgments
Funding: This study received funding from the Ministry of Education’s Industry-University Cooperative Education Project (project No: 202102242016, to Wei Chen).
Footnote
Reporting Checklist: The authors have completed the ARRIVE reporting checklist. Available at https://atm.amegroups.com/article/view/10.21037/atm-22-4013/rc
Data Sharing Statement: Available at https://atm.amegroups.com/article/view/10.21037/atm-22-4013/dss
Conflicts of Interest: All authors have completed the ICMJE uniform disclosure form (available at https://atm.amegroups.com/article/view/10.21037/atm-22-4013/coif). WC reports that this study received funding from the Ministry of Education’s Industry-University Cooperative Education Project (project No: 202102242016). The other authors have no conflicts of interest to declare.
Ethical Statement:
Open Access Statement: This is an Open Access article distributed in accordance with the Creative Commons Attribution-NonCommercial-NoDerivs 4.0 International License (CC BY-NC-ND 4.0), which permits the non-commercial replication and distribution of the article with the strict proviso that no changes or edits are made and the original work is properly cited (including links to both the formal publication through the relevant DOI and the license). See: https://creativecommons.org/licenses/by-nc-nd/4.0/.
References
- de Benedictis FM, Kerem E, Chang AB, et al. Complicated pneumonia in children. Lancet 2020;396:786-98. [Crossref] [PubMed]
- Eshwara VK, Mukhopadhyay C, Rello J. Community-acquired bacterial pneumonia in adults: An update. Indian J Med Res 2020;151:287-302. [Crossref] [PubMed]
- Nair GB, Niederman MS. Updates on community acquired pneumonia management in the ICU. Pharmacol Ther 2021;217:107663. [Crossref] [PubMed]
- Pneumonia. Nat Rev Dis Primers 2021;7:26. [Crossref] [PubMed]
- Torres A, Cilloniz C, Niederman MS, et al. Pneumonia. Nat Rev Dis Primers 2021;7:25. [Crossref] [PubMed]
- Quinton LJ, Walkey AJ, Mizgerd JP. Integrative Physiology of Pneumonia. Physiol Rev 2018;98:1417-64. [Crossref] [PubMed]
- Hespanhol V, Bárbara C. Pneumonia mortality, comorbidities matter? Pulmonology 2020;26:123-9. [Crossref] [PubMed]
- Zhang Z, Wang P, Ma C, et al. Host inflammatory response is the major factor in the progression of Chlamydia psittaci pneumonia. Front Immunol 2022;13:929213. [Crossref] [PubMed]
- Liu X, Lin Z, Yin X. Pellino2 accelerate inflammation and pyroptosis via the ubiquitination and activation of NLRP3 inflammation in model of pediatric pneumonia. Int Immunopharmacol 2022;110:108993. [Crossref] [PubMed]
- Li S, Cui W, Song Q, et al. miRNA-302e attenuates inflammation in infantile pneumonia though the RelA/BRD4/NF-κB signaling pathway. Int J Mol Med 2019;44:47-56. [Crossref] [PubMed]
- Muhammad W, Zhai Z, Wang S, et al. Inflammation-modulating nanoparticles for pneumonia therapy. Wiley Interdiscip Rev Nanomed Nanobiotechnol 2022;14:e1763. [Crossref] [PubMed]
- Mejías A, Chávez-Bueno S, Ramilo O. Respiratory syncytial virus pneumonia: mechanisms of inflammation and prolonged airway hyperresponsiveness. Curr Opin Infect Dis 2005;18:199-204. [Crossref] [PubMed]
- Barriga M, Benitez R, Ferraz-de-Paula V, et al. Protective role of cortistatin in pulmonary inflammation and fibrosis. Br J Pharmacol 2021;178:4368-88. [Crossref] [PubMed]
- Mizgerd JP. Inflammation and Pneumonia: Why Are Some More Susceptible than Others? Clin Chest Med 2018;39:669-76. [Crossref] [PubMed]
- Yende S, Tuomanen EI, Wunderink R, et al. Preinfection systemic inflammatory markers and risk of hospitalization due to pneumonia. Am J Respir Crit Care Med 2005;172:1440-6. [Crossref] [PubMed]
- Jobs A, Simon R, de Waha S, et al. Pneumonia and inflammation in acute decompensated heart failure: a registry-based analysis of 1939 patients. Eur Heart J Acute Cardiovasc Care 2018;7:362-70. [Crossref] [PubMed]
- Niederman MS, Ahmed QA. Inflammation in severe pneumonia: act locally, not globally. Crit Care Med 1999;27:2030-2. [Crossref] [PubMed]
- Beisswenger C, Honecker A, Kamyschnikow A, et al. Moxifloxacin modulates inflammation during murine pneumonia. Respir Res 2014;15:82. [Crossref] [PubMed]
- Liu M, Li W, Song F, et al. Silencing of lncRNA MIAT alleviates LPS-induced pneumonia via regulating miR-147a/NKAP/NF-κB axis. Aging (Albany NY) 2020;13:2506-18. [Crossref] [PubMed]
- Zhou Z, Zhu Y, Gao G, et al. Long noncoding RNA SNHG16 targets miR-146a-5p/CCL5 to regulate LPS-induced WI-38 cell apoptosis and inflammation in acute pneumonia. Life Sci 2019;228:189-97. [Crossref] [PubMed]
- Zeng M, Sang W, Chen S, et al. 4-PBA inhibits LPS-induced inflammation through regulating ER stress and autophagy in acute lung injury models. Toxicol Lett 2017;271:26-37. [Crossref] [PubMed]
- Fan Y, Wang J, Feng Z, et al. Pinitol attenuates LPS-induced pneumonia in experimental animals: Possible role via inhibition of the TLR-4 and NF-κB/IκBα signaling cascade pathway. J Biochem Mol Toxicol 2021;35:e22622. [Crossref] [PubMed]
- Yang W, Han B, Chen Y, et al. SAAL1, a novel oncogene, is associated with prognosis and immunotherapy in multiple types of cancer. Aging (Albany NY) 2022;14:6316-37. [Crossref] [PubMed]
- Saranya Revathy K, Umasuthan N, Whang I, et al. A novel acute phase reactant, serum amyloid A-like 1, from Oplegnathus fasciatus: genomic and molecular characterization and transcriptional expression analysis. Dev Comp Immunol 2012;37:294-305. [Crossref] [PubMed]
- Sato T, Fujii R, Konomi K, et al. Overexpression of SPACIA1/SAAL1, a newly identified gene that is involved in synoviocyte proliferation, accelerates the progression of synovitis in mice and humans. Arthritis Rheum 2011;63:3833-42. [Crossref] [PubMed]
- Fujii R, Komatsu R, Sato T, et al. SPACIA1/SAAL1 Deletion Results in a Moderate Delay in Collagen-Induced Arthritis Activity, along with mRNA Decay of Cyclin-dependent Kinase 6 Gene. Int J Mol Sci 2018;19:3828. [Crossref] [PubMed]
- Chu PY, Tung SL, Tsai KW, et al. Identification of the Novel Oncogenic Role of SAAL1 and Its Therapeutic Potential in Hepatocellular Carcinoma. Cancers (Basel) 2020;12:1843. [Crossref] [PubMed]
- Marangu D, Zar HJ. Childhood pneumonia in low-and-middle-income countries: An update. Paediatr Respir Rev 2019;32:3-9. [Crossref] [PubMed]
- Li Y, Li H, Liu S, et al. Pirfenidone ameliorates lipopolysaccharide-induced pulmonary inflammation and fibrosis by blocking NLRP3 inflammasome activation. Mol Immunol 2018;99:134-44. [Crossref] [PubMed]
- Bai C, Liu T, Xu J, et al. Effect of High Calorie Diet on Intestinal Flora in LPS-Induced Pneumonia Rats. Sci Rep 2020;10:1701. [Crossref] [PubMed]
- Pandher U, Kirychuk S, Schneberger D, et al. Lung inflammation from repeated exposure to LPS and glyphosate. Cell Tissue Res 2021;386:637-48. [Crossref] [PubMed]
- Gui Y, Sun L, Liu R, et al. Pachymic acid inhibits inflammation and cell apoptosis in lipopolysaccharide (LPS)-induced rat model with pneumonia by regulating NF-κB and MAPK pathways. Allergol Immunopathol (Madr) 2021;49:87-93. [Crossref] [PubMed]
- Zhang D, Lee H, Wang X, et al. A potential role of microvesicle-containing miR-223/142 in lung inflammation. Thorax 2019;74:865-74. [Crossref] [PubMed]
- Zhang L, Dong L, Tang Y, et al. MiR-146b protects against the inflammation injury in pediatric pneumonia through MyD88/NF-κB signaling pathway. Infect Dis (Lond) 2020;52:23-32. [Crossref] [PubMed]
- Zhang J, Chi H, Xiao H, et al. Interleukin 6 (IL-6) and Tumor Necrosis Factor α (TNF-α) Single Nucleotide Polymorphisms (SNPs), Inflammation and Metabolism in Gestational Diabetes Mellitus in Inner Mongolia. Med Sci Monit 2017;23:4149-57. [Crossref] [PubMed]
- Pedersen BK. Anti-inflammatory effects of exercise: role in diabetes and cardiovascular disease. Eur J Clin Invest 2017;47:600-11. [Crossref] [PubMed]
- Zhuang YT, Xu DY, Wang GY, et al. IL-6 induced lncRNA MALAT1 enhances TNF-α expression in LPS-induced septic cardiomyocytes via activation of SAA3. Eur Rev Med Pharmacol Sci 2017;21:302-9. [PubMed]
- Nigro E, Polito R, Elce A, et al. Physical Activity Regulates TNFα and IL-6 Expression to Counteract Inflammation in Cystic Fibrosis Patients. Int J Environ Res Public Health 2021;18:4691. [Crossref] [PubMed]
- Lu Q, Zhu Z, Tan C, et al. Changes of serum IL-10, IL-1β, IL-6, MCP-1, TNF-α, IP-10 and IL-4 in COVID-19 patients. Int J Clin Pract 2021;75:e14462. [Crossref] [PubMed]
- Bacci MR, Leme RC, Zing NP, et al. IL-6 and TNF-α serum levels are associated with early death in community-acquired pneumonia patients. Braz J Med Biol Res 2015;48:427-32. [Crossref] [PubMed]
- Tian F, Chen LP, Yuan G, et al. Differences of TNF-α, IL-6 and Gal-3 in lobar pneumonia and bronchial pneumonia caused by mycoplasma pneumoniae. Technol Health Care 2020;28:711-9. [Crossref] [PubMed]
- Kim YK, Shin JS, Nahm MH. NOD-Like Receptors in Infection, Immunity, and Diseases. Yonsei Med J 2016;57:5-14. [Crossref] [PubMed]
- Chow JC, Young DW, Golenbock DT, et al. Toll-like receptor-4 mediates lipopolysaccharide-induced signal transduction. J Biol Chem 1999;274:10689-92. [Crossref] [PubMed]
- Bi D, Wang Y, Gao Y, et al. Recognition of Lipopolysaccharide and Activation of NF-kappaB by Cytosolic Sensor NOD1 in Teleost Fish. Front Immunol 2018;9:1413. [Crossref] [PubMed]
- Swain B, Basu M, Samanta M. NOD1 and NOD2 receptors in mrigal (Cirrhinus mrigala): inductive expression and downstream signalling in ligand stimulation and bacterial infections. J Biosci 2013;38:533-48. [Crossref] [PubMed]
- Hysi P, Kabesch M, Moffatt MF, et al. NOD1 variation, immunoglobulin E and asthma. Hum Mol Genet 2005;14:935-41. [Crossref] [PubMed]
- Irving AT, Mimuro H, Kufer TA, et al. The immune receptor NOD1 and kinase RIP2 interact with bacterial peptidoglycan on early endosomes to promote autophagy and inflammatory signaling. Cell Host Microbe 2014;15:623-35. [Crossref] [PubMed]
- Berrington WR, Iyer R, Wells RD, et al. NOD1 and NOD2 regulation of pulmonary innate immunity to Legionella pneumophila. Eur J Immunol 2010;40:3519-27. [Crossref] [PubMed]
- Wang H, Lin X, Pu X. NOD-like receptors mediate inflammatory lung injury during plateau hypoxia exposure. J Physiol Anthropol 2020;39:32. [Crossref] [PubMed]
- Zhao N, Di B, Xu LL. The NLRP3 inflammasome and COVID-19: Activation, pathogenesis and therapeutic strategies. Cytokine Growth Factor Rev 2021;61:2-15. [Crossref] [PubMed]
- Freeman TL, Swartz TH. Targeting the NLRP3 Inflammasome in Severe COVID-19. Front Immunol 2020;11:1518. [Crossref] [PubMed]
- Donovan C, Liu G, Shen S, et al. The role of the microbiome and the NLRP3 inflammasome in the gut and lung. J Leukoc Biol 2020;108:925-35. [Crossref] [PubMed]