Atorvastatin alleviates oxidative damage by activating the nuclear factor erythroid 2-related factor 2 pathway after brain ischemia in mice
Introduction
Cerebral infarction, also known as ischemic stroke, is the block of blood supply to the brain, contributing to inadequate oxygen as well as glucose in brain tissues. As one of the greatest contributors to death in China behind malignant tumors and heart disease (1), stroke has been the highest cause of years of life lost (YLLs) in China (2). In recent years, research on ischemic cerebral apoplexy has mainly focused on various measures to improve blood circulation in the brain, such as thrombolytic, anti-platelet, and anti-coagulation therapies, among others (3-5). However, these treatment methods have limited therapeutic efficacy, more contraindications, and bleeding risks, and as such, only few patients can benefit from them (6). Accordingly, it is pivotal to continue to seek novel therapeutic targets.
Ischemic stroke is defined as ischemic hypoxic necrosis of brain tissues, and oxidative stress is an important mechanism of secondary brain injury after stroke (7). After stroke, abundant reactive oxygen species (ROS) are produced, which poses a challenge to the body and breaks the balance between the oxidation and antioxidant systems, causing damage to neurons (8). Studies have shown that ROS produced by oxidative stress can induce autophagy and thus play a regulatory role in cell damage and inflammation (9,10). Autophagy can eliminate damaged or aging cells, so that cells can maintain homeostasis and survival under various stress conditions (11).
Nuclear factor E2-related factor 2 (Nrf2) belongs to the family of Cap-n-collar (CNC) transcription factors. Nrf2, which acts as a critical player in oxidative stress, can be mediated by Keap1 in the cytoplasm. Activated Nrf2 dissociates from Keap1 and regulates the expression of antioxidant proteins and phase II enzymes by interacting with antioxidant response elements (AREs) after nuclear entry, thereby promoting the levels of various enzymatic and non-enzymatic antioxidant genes including HO-1, NADP(H):NQO1, and GST (12,13). Being a ubiquitin-binding protein, P62, which acts as an indispensable cargo receptor in autophagy, inhibits Keap1, activates Nrf2, and protects against oxidative stress (14).
Atorvastatin is a selective and competitive inhibitor of hydroxymethylglutaryl coenzyme A (HMG-CoA) reductase. It is demonstrated that atorvastatin can reduce the combination of cholesterol, rescue ischemia, resist inflammation, and ameliorate the function of vascular endothelial cells. Atorvastatin also represses inflammation and lipid deposition, but improves the balance of vulnerable atherosclerotic plaques via the regulation of autophagy (15). It has been demonstrated that atorvastatin activates autophagy and promotes the recovery of nerve function after spinal cord injury (16). In addition, our previous work has shown that atorvastatin exerts anti-inflammatory properties by alleviating microglia-mediated neuroinflammation and downregulating high mobility group box (HMGB1) and nuclear factor kappa-B (NF-κB) expression in ischemic stroke (17-19). Our previous work also reveals that oxidative damage plays an important role in cerebral ischemic pathogenesis (20). However, it is not clear whether the effect of atorvastatin against ischemic stroke is related to antioxidants. The genes that can scavenge free radicals, detoxify xenobiotics, and maintain redox potential can be modulated by Nrf2. Therefore, it is speculated that atorvastatin exerts a protective effect on neurons by targeting Nrf2 to regulate oxidative stress-dependent autophagy.
Objectives
Herein, a distal middle cerebral artery occlusion (dMCAO) model by electrocoagulation was established to perform focal ischemic cerebral infarction research and to achieve the following aims: (I) establish animal models that are reliable, simple, practicable, and close to the clinical reality; (II) highlight some novel and innovative mechanisms of the antioxidant effects of atorvastatin; (III) we used Nrf2−/− mice to explore the relationship between the protective effect of atorvastatin and the Nrf2 signaling pathway and related antioxidant systems. In order to provide a theoretical basis for further research on the mechanism of action of atorvastatin, in this experiment, the effects of atorvastatin on mice with focal permanent cerebral infarction and oxidative stress through Nrf2 signaling and autophagy were explored. We present the following article in accordance with the ARRIVE reporting checklist (available at https://atm.amegroups.com/article/view/10.21037/atm-22-4565/rc).
Methods
Animals
Clean-grade healthy adult male ICR mice (8–12 weeks old, 25–30 g body weight) were provided by Beijing Viton Lever Laboratory Animal Technology Co., Ltd. Wild-type (Nrf2+/+) and Nrf2-deficient (Nrf2−/−) ICR mice (8 weeks old, male) were obtained from academician Chunyan Li (Department of Neurology, The Second Hospital of Hebei Medical University, Shijiazhuang, China) and were genotyped using primers as follows: 5'-TGGACGGGACTATTGAAGGCTG-3' (sense for Nrf2+/+ and Nrf2−/−), 5'-GCCGCCTTTTCAGTAGATGGAGG-3' (antisense for Nrf2−/−), and 5'-GCGGATTGACCGTAATGGGATAGG-3' (antisense for Nrf2−/−). Nrf2+/+ means mice with the Nrf2 gene, and Nrf2−/− means mice with the Nrf2 gene knock out. The identification of mouse genotypes was implemented by examining the bands of the polymerase chain reaction (PCR) products. All animals individually resided in separate cages with controlled temperature and a 12-h light/12-h dark cycle, and fed at libitum and drank water freely. Forty mice were randomly separated into 5 groups (n=8): sham (no induced dMCAO model), vehicle (dMCAO model), Atorv-H (dMCAO mice were given 40 mg/kg atorvastatin), Atorv-M (dMCAO mice were given 20 mg/kg atorvastatin), and Atorv-L (dMCAO mice were given 10 mg/kg atorvastatin) groups. The animal experiments were approved under a project license (No. 2020-AE009) granted by the Ethics Committee of Animal Experiments of the Second Hospital of Hebei Medical University and conducted in accordance with national guidelines for the care and use of animals.
Construction of the dMCAO model by electrocoagulation
The mouse dMCAO model was established as described in previous study (21). The anesthetization of mice was carried out through intraperitoneal injection of Avertin (2.4 mg/10 g) (Sigma-Aldrich, St. Louis, MO, USA). The unconscious mice were fixed in the supine position on the operating table and blunt separation of cervical glandular tissue, muscle, and fascia was performed. The exposure of the right cervical triangle was initially conducted, after which the right common carotid artery was separated. The right common carotid artery was permanently ligated and the subcutaneous tissues and skin of the neck were sutured. Then, the mouse was fixed on the operating table in the left lateral position, and a 0.5 cm incision was made between the right lateral canthus and the right external auditory canal to expose the temporalis muscle, which was cut off under a microscope to separate and expose the skull. Subcranial branches of the right medial cerebral artery cortex were microscopically visible. Subsequently, a small hole about 2 mm in diameter was drilled into the skull with a cranial drill to expose the cortical branch of the right middle cerebral artery. The right middle cerebral artery distal to the striatal branch was permanently coagulated with a coagulator (RWD Life Science, Shenzhen, China) under a microscope. The model was proven successful if there was no blood reflow in the cauterized arterial branches for a few seconds. For the sham operation group, no ligation was performed after the separation of the right common carotid artery, the skull was exposed and drilled, and the cauterization of appropriate arteries was not implemented. The remaining operation steps were the same as previously mentioned. Body temperature was kept at 37 ℃ until the mice woke from anesthesia. The animals were monitored every day, and the criteria used for humane endpoints of this experiment were as follows: a marked reduction in food or water intake, labored breathing, inability to stand, and no response to external stimuli. No abnormal signs that signified the humane endpoints of the experiment were observed from any of the mice during the experiment. Death was verified by the lack of heartbeat and a cold body.
Administration of atorvastatin
Atorvastatin calcium tablets (Pfizer Inc., New York, NY, USA) were dissolved in 0.9% saline solution to prepare the intragastric treatment for the mice. The dose was 10 mg/kg in the low-dose group, 20 mg/kg in the medium-dose group, and 40 mg/kg in the high-dose group. Atorvastatin was administered to mice in the treatment group by gavage 7 days before surgery, once a day, until sampling. An equal volume of 0.9% saline solution without the drug was given to the sham group and vehicle group.
Rotarod test
The rotarod test has been utilized to investigate the motor coordination ability and anti-fatigue ability of mice (22). In a quiet and relatively balanced environment, daily training and testing were conducted at a fixed period. Mice from each group received 5-day wheel training on the rotarod cylinder (Ugo Basile 47,600, Italy) with speed of 4 RPM before modeling, and the mice that remained for at least 60 consecutive seconds could be included in the group. Mice in each group were tested for rotarod behavior before and after surgery at 24, 48, and 72 h separately. The mice were seated on the rotating bar, and the rotation speed increased from 4 to 40 RPM in 4 min. The time from the beginning to falling from the wheel was recorded. The average time of 3 independent experiments for each mouse was calculated. The sample size was 12.
Modified neurological severity score (mNSS)
The mNSS was mainly applied to assess movement, sensation, balance, reflex of mice (23). The scores of the scale vary from 0 to 18.0, with 0 indicating complete functionality, while higher scores represent increased severity of the neurological injury. In a quiet and relatively balanced environment, the mNSS behavior test was conducted and recorded in each group at 24, 48, and 72 h following the operation. The sample size was 12.
Measurements of brain infarct volume
At 72 h following surgery, the mice were sacrificed for the isolation of brain tissues, after which they were immediately frozen at −20 ℃ for 20 min. Subsequently, sections of brain tissues were cut at 1 mm intervals. The slices were subjected to 2% 2,3,5-triphenyltetrazolium chloride (TTC) staining (Sigma-Aldrich, St. Louis, MO, USA). The areas of infarcted tissue in both hemispheres of each brain slice were determined by using image analysis software (Image-Pro Plus 6.0 software). The calculation of infarct volume was implemented by the following formula: the percentage of hemisphere lesion volume after edema correction = (total infarct volume − [right hemisphere volume − left hemisphere volume]/left hemisphere volume × 100%. The sample size was 6.
Brain water content test
At 72 h following surgery, the mice were sacrificed to obtain their brain tissues. The brain tissues in the infarcted area were isolated and weighed immediately, then wrapped in tin foil and placed in an electric drying box (Shanghai Zhicheng Analysis Instrument Manufacturing Co., Ltd., Shanghai, China) at 95 ℃ for 24 h. The weight was monitored and the dry weight was recorded when the reading stabilized. The value was calculated by the following formula: brain water content (%) = (wet weight − dry weight)/wet weight × 100%. The sample size was 6.
Cerebral blood flow (CBF) monitoring
Laser Speckle Contrast Imaging (PeriCam PSI System, Perimed, Sweden) was used to monitor real-time 2D CBF. Following the successful anesthetization of mice, the head was fixed with a stereoscopic localizer. The periosteum was separated following scalp opening and the skull was exposed. The skull surface was kept moist with normal saline, and the impurities on the skull surface were removed. The mice were then scanned with a stereo locator under a laser speckle imaging system to measure the CBF in both hemispheres and obtain images at a working distance of 10 cm from the skull surface. To assess changes in CBF, a computer program (PimSoft 1.3, Perimed AB, Sweden) was used to calculate the average perfusion level of the region of interest (ROI) in the right cortical infarcted area. The following formula was used to calculate the change in CBF: (CBF at each time point)/(preischemic CBF) × 100%. The sample size was 6.
Enzyme-linked immunosorbent assay (ELISA)
The activity of superoxide dismutase (SOD) and Catalase (CAT) and the expression of glutathione (GSH) and malondialdehyde (MDA) were quantified using commercially available ELISA kits. The SOD assay kit (cat no. A001-3-2), CAT assay kit (cat no. A007-1-1), GSH assay kit (cat no. A006-2-1), and MDA assay kit (cat no. A003-1-2) were provided by Nanjing Jiancheng Bioengineering Institute Co., Ltd. ELISA assays were conducted using a standard protocol. The sample size was 4.
Western blot
Total protein, cytoplasm proteins, and cytonuclear proteins were extracted from the cortical brain tissues of the ischemic side according to the instructions of the protein extraction kit (Beijing Pleilai Company, Beijing) and cytoplasm and cytonuclear protein extraction kits (Kangwei Century Biological Technology Co., Ltd., China). Subsequently, the contents of total proteins, cytoplasm proteins, and cytonuclear proteins were detected using the BCA kit (Novagen, USA). Protein samples of the same quality (50 µg) were obtained and SDS-PAGE gel electrophoresis was conducted. Proteins were transferred to PVDF membranes, which were then blocked by 5% milk sealant. The following primary antibodies were applied to the membranes and incubated overnight at 4 ℃: Nrf2 (ab62352; 1:1,000; Abcam), Beclin1 (ab207612; 1:2,000; Abcam), HO-1 (ab52947; 1:1,000; Abcam), NQO1 (ab80588; 1:800; Abcam), β-tubulin (ab179513; 1:2,000; Abcam), LC3B (ab48394; 1:2,000; Abcam), P62 (arg57285; 1:1,000; Arigo), GAPDH (ab9485; 1:5,000; Abcam), and H3 (ab1971; 1:2,000; Abcam). Subsequently, the TBST-rinsed membranes were exposed to a secondary antibody (611-103-122; 1:10,000; Rockland) and then oscillated for 1 h at room temperature. Finally, the signals were detected using an enhanced chemiluminescence reagent (GE Healthcare, Chicago, IL, USA), and Image J software (version 146; National Institutes of Health, Bethesda, MD, USA) was employed for the analysis of the fold changes of protein levels. The sample size was 4.
Immunofluorescence (IF) staining
Mice were deeply anesthetized by intraperitoneal injection of Avertin and then fixed by transcardial perfusion with cold saline quickly for 2 min as well as 4% paraformaldehyde for 10 min. A cryotome (Thermo Fisher Scientific, USA) was applied to obtain frozen coronal brain sections (15 µm thick). Brain slices were washed with PBS 3 times every 10 min. After 15 min of permeabilization with 0.5% Triton X-100, the slices were blocked by 10% donkey serum at 37 ℃ for 1 h and subsequently probed with Nrf2 antibody (ab62352; 1:100; Abcam) overnight at 4 ℃. On the second day, the PBS-rinsed sections were subjected to Alexa Fluor 488-AffiniPure Donkey Anti-Rabbit IgG antibody (AB_2313584; 1:700; Jackson ImmunoResearch La) at 37 ℃ for 1 h. DAPI (Southern Biotech, USA) was used to stain the nuclei. An imaging system for immunofluorescence was used (Zeiss LSM880, Germany) to obtain immunofluorescence images. ImageJ was used to analyze the volume density of Nrf2 expression.
Statistical analysis
All data that were displayed in the form of mean ± SD were analyzed with SPSS 22.0 (IBM Corp., New York, NY, USA) and GraphPad Prism 5.0 (GraphPad Software, Inc., San Diego, CA, USA). Data were analyzed using either analysis of variance (ANOVA) followed by a Tukey’s post-hoc test for the comparison of multiple groups or an independent Student’s t-test for the comparison of 2 groups. P<0.05 indicated a statistical difference.
Results
Atorvastatin plays a protective role in the brains of mice with cerebral infarction
Through assessing the rotarod neurological function scores of mice in each group, it was discovered that relative to the sham group, the vehicle group had severe postoperative neurological impairment. Relative to the vehicle group, atorvastatin effectively rescued the damaged neurological function, of which the Atorv-M group had the most significant therapeutic effect (Figure 1A). The mNSS results showed that there was no obvious difference between the vehicle group and Atorv-M and Atorv-H group at 24 and 48 h after surgery. At 72 h after surgery, the scores of nerve defects in the Atorv-M group were markedly diminished compared with the vehicle group (Figure 1B). The body weight of the mice in each group after surgery generally declined during this period (Figure 1C). In this way, 20 mg/kg atorvastatin was adopted for follow-up experiments.
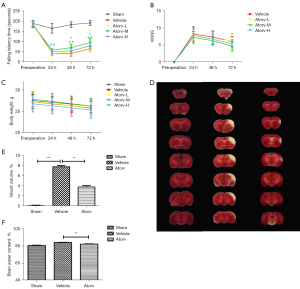
TCC staining results demonstrated that the volume of cerebral infarction in the Atorv group significantly reduced relative to the vehicle group (Figure 1D,1E). The brain water content of the Atorv group was also significantly reduced relative to the vehicle group, which indicated that atorvastatin relieved brain infarct volume and brain edema after stroke (Figure 1F).
In addition, the cerebral perfusion in the left cerebral hemisphere decreased to about 95%, but in the right cerebral hemisphere it decreased to about 55% immediately. The cerebral perfusion of the right cortical cerebral artery gradually recovered over time, and the recovery was faster in the Atorv group than in the vehicle group. However, no statistically significant relationship was observed (Figure S1A-S1C). This might be related to the small ischemia range of this model, and CBF is a macro index with a small amount of change in a short time period.
Atorvastatin promotes Nrf2 nuclear translocation and activates autophagy in mice with cerebral infarction
With the aim of investigating the impact of atorvastatin on oxidative stress as well as autophagy, we detected the related indexes. The levels of SOD (Figure 2A), CAT (Figure 2B), GSH (Figure 2C), and MDA (Figure 2D) were detected at 72 h after the operation. Relative to the sham group, the activities of SOD, CAT, and GSH all reduced. Relative to the vehicle group, the levels of SOD, CAT, and GSH increased while the level of MDA reduced in the Atorv group, indicating that atorvastatin could inhibit oxidative stress in ischemic stroke mice. Furthermore, the contents of Nrf2 protein in the cytoplasm and nucleus of brain cells were detected and it was discovered that compared with the sham group, the Nrf2 content in the nucleus of the Atorv group increased (Figure 3A,3B), but was decreased in the cytoplasm (Figure 3C,3D). IF staining results also indicated that atorvastatin treatment significantly translocated Nrf2 to the nucleus (Figure 3E,3F). Relative to the vehicle group, the contents of autophagy-related proteins LC3II/I and Beclin1 were significantly increased in the Atorv group (Figure 3G), indicating that autophagy was activated by the application of atorvastatin.
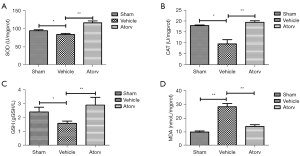
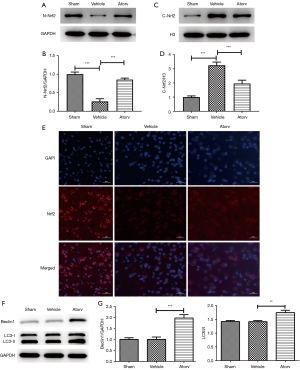
Atorvastatin plays a protective role in the brains of mice with cerebral infarction by activating the Nrf2 signaling pathway
To identify how atorvastatin regulated the Nrf2 gene, we generated Nrf2−/− mice for the experiments. Firstly, RT-qPCR was used to identify the genes of Nrf2+/+ and Nrf2−/− mice. The results showed that the Nrf2 gene was successfully knocked out (Figure S2). The mice were grouped into sham, Nrf2+/+ vehicle, Nrf2+/+ Atorv, Nrf2−/− vehicle, and Nrf2−/− Atorv groups. The rotarod neurological function score showed that the neurological impairment of Nrf2+/+ mice treated with atorvastatin was significantly lower than that of other groups. The neurological impairment of Nrf2−/− mice treated with atorvastatin was only different from that of the Nrf2−/− vehicle group at 72 h, and the recovery was slower (Figure 4A). The trend of the mNSS neural defect score was consistent with that of the rotarod results (Figure 4B). TTC staining results showed that the cerebral infarction volume of the Nrf2+/+ Atorv group was significantly smaller than that of the other groups, and the cerebral infarction volume of the Nrf2−/− vehicle group was significantly larger than that of the Nrf2+/+ vehicle group (Figure 4C,4D). The results showed that atorvastatin protected the brains of mice against cerebral infarction through the Nrf2 signaling pathway.
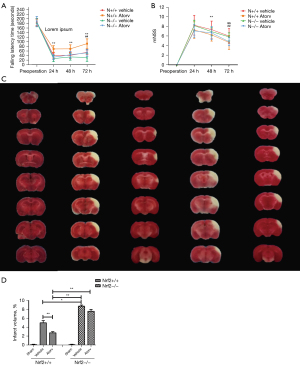
Atorvastatin inhibits oxidative stress and activates autophagy by activating the P62/Nrf2/ARE signaling pathway
To further investigate the mechanism of the role of atorvastatin on the cerebral infarction, the expression levels of SOD and the downstream genes of the Nrf2 pathway such as HO-1 and NQO1 and other antioxidant proteins were detected. The results showed that SOD activity in the brain tissues of the Nrf2+/+ Atorv group was higher than that of the other groups (Figure 5A). There was no significant difference in the expression of HO-1 and NQO1 in Nrf2−/− mice after atorvastatin administration compared with the vehicle group. However, the expression of HO-1 and NQO1 were increased after atorvastatin was administered without Nrf2 knockout (Figure 5B,5C), which further demonstrated that atorvastatin played a protective role in the brains of mice with cerebral infarction by activating the Nrf2/HO-1 signaling pathway. In addition, P62 expression in the Nrf2+/+ Atorv group was markedly elevated compared to the Nrf2+/+ vehicle group, while the P62 level in the Nrf2−/− Atorv group was lower than that of the Nrf2−/− vehicle group (Figure 5D,5E). These results indicated that atorvastatin activated the Nrf2 gene and upregulated the expression of P62. Elevated expression of P62 might be promoted by positive feedback of Nrf2 activation.
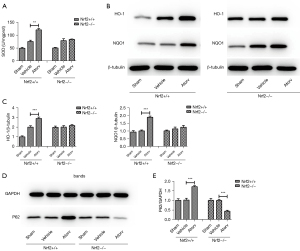
Discussion
Atorvastatin has important application value in clinic. Atorvastatin reduces blood lipids, thereby reducing the risk of cardiovascular events and stroke, as well as platelet accumulation in the blood vessels of patients with cardiovascular disease, diabetes, and ischemic stroke (24). In addition, atorvastatin has the ability to fight atherosclerosis and lower blood pressure levels (25). Moreover, the combination of atorvastatin with other drugs can increase the efficacy and reduce the adverse reactions of drugs (26). In this paper, 10, 20, 40 mg/kg atorvastatin were used for the experiment and finally the concentration of 20 mg/kg atorvastatin was determined for the experiment (27). The clinical dose corresponding to this concentration is also safe. And the study found that atorvastatin significantly alleviated neurological deficits following cerebral infarction, reduced the volume of cerebral infarction, and exerted an antioxidative effect through Nrf2 signaling. Our results were consistent with those of Kho et al. who demonstrated that atorvastatin could reduce global cerebral ischemia-induced neuronal death (28).
The mechanism of secondary brain injury in ischemic stroke includes a series of pathological processes, including abnormal energy metabolism, calcium overload, inflammation, and oxidative stress, all of which can directly or indirectly lead to neuron death (29). Oxidative stress is a pathological process in which excessive ROS are produced and endogenous antioxidant reactions occur when the body is subjected to various harmful stimuli. In the process of ischemic stroke, numerous ROS are generated, which can aggravate ischemia-reperfusion injury in a variety of ways, including blood-brain barrier destruction, inflammation, and apoptosis (7,30,31). Excess ROS can be removed by various antioxidants such as SOD, GSH, and CAT (30). Additionally, MDA can indirectly reflect the degree of free radical lipid oxidation (32). Our results showed that the levels of SOD, GSH, and CAT were markedly reduced while the MDA level was elevated following the occurrence of cerebral infarction in mice, indicating that the oxidative stress level of the ischemic stroke mice increased. The oxidative stress level was inhibited after atorvastatin administration.
In the state of stress, Nrf2 dissociates from Keap1 in the cytoplasm and is transferred into the nucleus. Nrf2 combines with AREs to activate the expression of downstream genes, thus generating antioxidant enzymes (33). Atorvastatin was shown to reduce the oxidative stress caused by hyperglycemia through activating the expression of Nrf2/HO-1, thus improving the inflammation and apoptosis of diabetic rats (34). In addition, atorvastatin regulated antioxidant enzymes through the Nrf2 signaling pathway to protect renal tissues (35). Our results showed that the amount of Nrf2 protein in the atorvastatin treatment group was increased in the nucleus and decreased in the cytoplasm 72 h after surgery, suggesting that the antioxidant effect of atorvastatin might be mediated by nuclear translocation of Nrf2.
ROS produced by oxidative stress can induce autophagy, which can reduce the cell damage caused by oxidative stress in turn (36). The role that autophagy plays in ischemic disease is still controversial. A previous study has shown that activation of autophagy is beneficial to injury repair (37), and other study has shown that reduction of autophagy has a protective effect on neuron death (38). The experimental data here further demonstrated that the expression of autophagy proteins was elevated after atorvastatin administration, indicating that autophagy played a protective role in the brain. It has been reported that P62, an autophagy substrate, is downregulated when autophagy is activated (39). However, in this paper, when autophagy strengthened, the P62 level was enhanced. Additionally, the expression of P62 was decreased after atorvastatin was administered to Nrf2−/− mice, indicating that the expression of P62 was correlated with Nrf2. On the one hand, P62 triggers Nrf2 by binding to Keap1 as well as mediating autophagy degradation. On the other hand, the P62 level is mediated by Nrf2, which can directly bind to the ARE promoter region to induce the expression of P62. P62 enhances its own expression through this promoter, thus forming positive feedback regulation (40).
In clinical and preclinical studies, the overall therapeutic benefit of multidrug combinations, as compared with monotherapy, is greater than the sum of the individual drug effects. The main mechanism of synergistic effect of combined drugs may act on the same or different signaling pathways, which can obtain equal or better therapeutic benefits at lower doses (41). The pathogenesis of ischemic cerebral infarction is complicated. Previous study has shown that probucol can enhance the anti-atherosclerosis function of statins, which can be applied to patients with oxidative stress, and probucol-mediated lipid-lowering effect can more effectively prevent the formation of early atherosclerotic lesions (20). However, the specific mechanism of effects of atorvastatin combined with probucol in ischemic diseases is unclear, and we will further explore it in future experiments.
In this study, it was found that both Nrf2+/+ mice and Nrf2−/− mice had improved neurological deficit symptoms after atorvastatin administration. However, the improvement in Nrf2−/− mice was not as good as that in Nrf2+/+ mice, suggesting that atorvastatin could indeed play a protective role in the brain though Nrf2 signaling pathways. Atorvastatin may also work in other ways, which is in agreement with our previous findings (18,19). There was no effect on SOD, HO-1, and NQO1 expression in Nrf2−/− mice, while the opposite was found in Nrf2+/+ mice after atorvastatin administration, indicating the antioxidant function of atorvastatin through the activation of downstream antioxidant genes and various enzymes of the Nrf2 signaling pathway.
Limitations
Our study has some shortcomings. We focused on whole brain tissues, but did not perform experiments on tissue sections related to the ischemic core/penumbra and/or in tissues with different sensitivity to ischemia (such as cortex vs. striatum). Experiments on these tissues will be conducted in our future studies. However, we applied Nrf2−/− mice and multiple experimental approaches to demonstrate that atorvastatin had protective properties against oxidative stress. Therefore, our results still provide solid evidence for the importance of atorvastatin.
Conclusions
The antioxidant effect of atorvastatin had a protective effect on the brains of mice with ischemic stroke. The underlying mechanism might be through the activation of autophagy and upregulation of P62 expression while activating the Nrf2 gene. Nonetheless, the relationship between Nrf2 and P62 still needs further study.
Acknowledgments
Funding: This work was generously supported by grants from the National Natural Science Foundation of China (No. 81974184), the Health Commission of Hebei Province (No. 20190550), and the Health Commission of Hebei Province (No. 20190061).
Footnote
Reporting Checklist: The authors have completed the ARRIVE reporting checklist. Available at https://atm.amegroups.com/article/view/10.21037/atm-22-4565/rc
Data Sharing Statement: Available at https://atm.amegroups.com/article/view/10.21037/atm-22-4565/dss
Conflicts of Interest: All authors have completed the ICMJE uniform disclosure form (available at https://atm.amegroups.com/article/view/10.21037/atm-22-4565/coif). The authors have no conflicts of interest to declare.
Ethical Statement: The authors are accountable for all aspects of the work in ensuring that questions related to the accuracy or integrity of any part of the work are appropriately investigated and resolved. The animal experiments were approved under a project license (No. 2020-AE009) granted by the Ethics Committee of Animal Experiments of the Second Hospital of Hebei Medical University and conducted in accordance with national guidelines for the care and use of animals.
Open Access Statement: This is an Open Access article distributed in accordance with the Creative Commons Attribution-NonCommercial-NoDerivs 4.0 International License (CC BY-NC-ND 4.0), which permits the non-commercial replication and distribution of the article with the strict proviso that no changes or edits are made and the original work is properly cited (including links to both the formal publication through the relevant DOI and the license). See: https://creativecommons.org/licenses/by-nc-nd/4.0/.
References
- Wang YJ, Li ZX, Gu HQ, et al. China Stroke Statistics: an update on the 2019 report from the National Center for Healthcare Quality Management in Neurological Diseases, China National Clinical Research Center for Neurological Diseases, the Chinese Stroke Association, National Center for Chronic and Non-communicable Disease Control and Prevention, Chinese Center for Disease Control and Prevention and Institute for Global Neuroscience and Stroke Collaborations. Stroke Vasc Neurol 2022; Epub ahead of print. [Crossref] [PubMed]
- Zhou M, Wang H, Zeng X, et al. Mortality, morbidity, and risk factors in China and its provinces, 1990-2017: a systematic analysis for the Global Burden of Disease Study 2017. Lancet 2019;394:1145-58. [Crossref] [PubMed]
- Zheng H, Bu S. Trial of Intravenous Alteplase before Endovascular Treatment for Stroke. N Engl J Med 2022;386:496. [Crossref] [PubMed]
- Yaşar E, Akalın Y, Aktaş İ, et al. The CHA2DS2-VASc risk score predicts successful endovascular treatment in patients with acute ischemic stroke. Acta Neurol Scand 2022;145:407-13. [Crossref] [PubMed]
- van der Steen W, van de Graaf RA, Chalos V, et al. Safety and efficacy of aspirin, unfractionated heparin, both, or neither during endovascular stroke treatment (MR CLEAN-MED): an open-label, multicentre, randomised controlled trial. Lancet 2022;399:1059-69. [Crossref] [PubMed]
- Nagahama H, Suzuki J, Sasaki M, et al. 8. Evaluation of the Therapeutic Mechanisms in Regeneration Therapy for Cerebral Infarction Using Pre-clinical Magnetic Resonance Imaging. Nihon Hoshasen Gijutsu Gakkai Zasshi 2018;74:722-5. [Crossref] [PubMed]
- Rodrigo R, Fernández-Gajardo R, Gutiérrez R, et al. Oxidative stress and pathophysiology of ischemic stroke: novel therapeutic opportunities. CNS Neurol Disord Drug Targets 2013;12:698-714. [Crossref] [PubMed]
- Cadenas S. ROS and redox signaling in myocardial ischemia-reperfusion injury and cardioprotection. Free Radic Biol Med 2018;117:76-89. [Crossref] [PubMed]
- Vikram A, Anish R, Kumar A, et al. Oxidative Stress and Autophagy in Metabolism and Longevity. Oxid Med Cell Longev 2017;2017:3451528. [Crossref] [PubMed]
- O'Grady SM. Oxidative stress, autophagy and airway ion transport. Am J Physiol Cell Physiol 2019;316:C16-32. [Crossref] [PubMed]
- Ravanan P, Srikumar IF, Talwar P. Autophagy: The spotlight for cellular stress responses. Life Sci 2017;188:53-67. [Crossref] [PubMed]
- Zhang DD, Lo SC, Cross JV, et al. Keap1 is a redox-regulated substrate adaptor protein for a Cul3-dependent ubiquitin ligase complex. Mol Cell Biol 2004;24:10941-53. [Crossref] [PubMed]
- Itoh K, Wakabayashi N, Katoh Y, et al. Keap1 represses nuclear activation of antioxidant responsive elements by Nrf2 through binding to the amino-terminal Neh2 domain. Genes Dev 1999;13:76-86. [Crossref] [PubMed]
- Pan JA, Sun Y, Jiang YP, et al. TRIM21 Ubiquitylates SQSTM1/p62 and Suppresses Protein Sequestration to Regulate Redox Homeostasis. Mol Cell 2016;62:149-51. [Crossref] [PubMed]
- Peng S, Xu LW, Che XY, et al. Atorvastatin Inhibits Inflammatory Response, Attenuates Lipid Deposition, and Improves the Stability of Vulnerable Atherosclerotic Plaques by Modulating Autophagy. Front Pharmacol 2018;9:438. [Crossref] [PubMed]
- Gao S, Zhang ZM, Shen ZL, et al. Atorvastatin activates autophagy and promotes neurological function recovery after spinal cord injury. Neural Regen Res 2016;11:977-82. [Crossref] [PubMed]
- Zhang P, Zhang X, Huang Y, et al. Atorvastatin alleviates microglia-mediated neuroinflammation via modulating the microbial composition and the intestinal barrier function in ischemic stroke mice. Free Radic Biol Med 2021;162:104-17. [Crossref] [PubMed]
- Wang L, Zhang X, Liu L, et al. Atorvastatin protects rat brains against permanent focal ischemia and downregulates HMGB1, HMGB1 receptors (RAGE and TLR4), NF-kappaB expression. Neurosci Lett 2010;471:152-6. [Crossref] [PubMed]
- Cui L, Zhang X, Yang R, et al. Neuroprotection of early and short-time applying atorvastatin in the acute phase of cerebral ischemia: down-regulated 12/15-LOX, p38MAPK and cPLA2 expression, ameliorated BBB permeability. Brain Res 2010;1325:164-73. [Crossref] [PubMed]
- Du Y, Zhang X, Ji H, et al. Probucol and atorvastatin in combination protect rat brains in MCAO model: upregulating Peroxiredoxin2, Foxo3a and Nrf2 expression. Neurosci Lett 2012;509:110-5. [Crossref] [PubMed]
- Piao CS, Gonzalez-Toledo ME, Xue YQ, et al. The role of stem cell factor and granulocyte-colony stimulating factor in brain repair during chronic stroke. J Cereb Blood Flow Metab 2009;29:759-70. [Crossref] [PubMed]
- Ishrat T, Pillai B, Soliman S, et al. Low-dose candesartan enhances molecular mediators of neuroplasticity and subsequent functional recovery after ischemic stroke in rats. Mol Neurobiol 2015;51:1542-53. [Crossref] [PubMed]
- Wang J, Yu L, Jiang C, et al. Bone marrow mononuclear cells exert long-term neuroprotection in a rat model of ischemic stroke by promoting arteriogenesis and angiogenesis. Brain Behav Immun 2013;34:56-66. [Crossref] [PubMed]
- Szarek M, Amarenco P, Callahan A, et al. Atorvastatin Reduces First and Subsequent Vascular Events Across Vascular Territories: The SPARCL Trial. J Am Coll Cardiol 2020;75:2110-8. [Crossref] [PubMed]
- Han Z, Wang Y, Li J. Effects of Atorvastatin Combined with Nano-Selenium on Blood Lipids and Oxidative Stress in Atherosclerotic Rats. J Nanosci Nanotechnol 2021;21:1331-7. [Crossref] [PubMed]
- Ai C, Zhang S, He Q, et al. Comparing the combination therapy of ezetimibe and atorvastatin with atorvastatin monotherapy for regulating blood lipids: a systematic review and meta-analyse. Lipids Health Dis 2018;17:239. [Crossref] [PubMed]
- Liu P, Gao Q, Guan L, et al. Atorvastatin Attenuates Isoflurane-Induced Activation of ROS-p38MAPK/ATF2 Pathway, Neuronal Degeneration, and Cognitive Impairment of the Aged Mice. Front Aging Neurosci 2020;12:620946. [Crossref] [PubMed]
- Kho AR, Hong DK, Kang BS, et al. The Effects of Atorvastatin on Global Cerebral Ischemia-Induced Neuronal Death. Int J Mol Sci 2021;22:4385. [Crossref] [PubMed]
- Chen XR, Liao SJ, Ye LX, et al. Neuroprotective effect of chondroitinase ABC on primary and secondary brain injury after stroke in hypertensive rats. Brain Res 2014;1543:324-33. [Crossref] [PubMed]
- Kahles T, Brandes RP. NADPH oxidases as therapeutic targets in ischemic stroke. Cell Mol Life Sci 2012;69:2345-63. [Crossref] [PubMed]
- Olmez I, Ozyurt H. Reactive oxygen species and ischemic cerebrovascular disease. Neurochem Int 2012;60:208-12. [Crossref] [PubMed]
- Koutina G, Jongberg S, Skibsted LH. Protein and lipid oxidation in Parma ham during production. J Agric Food Chem 2012;60:9737-45. [Crossref] [PubMed]
- Wakabayashi N, Dinkova-Kostova AT, Holtzclaw WD, et al. Protection against electrophile and oxidant stress by induction of the phase 2 response: fate of cysteines of the Keap1 sensor modified by inducers. Proc Natl Acad Sci U S A 2004;101:2040-5. [Crossref] [PubMed]
- Thongnak L, Pongchaidecha A, Jaikumkao K, et al. The additive effects of atorvastatin and insulin on renal function and renal organic anion transporter 3 function in diabetic rats. Sci Rep 2017;7:13532. [Crossref] [PubMed]
- Jaikumkao K, Pongchaidecha A, Chattipakorn N, et al. Atorvastatin improves renal organic anion transporter 3 and renal function in gentamicin-induced nephrotoxicity in rats. Exp Physiol 2016;101:743-53. [Crossref] [PubMed]
- Xu J, Wise JTF, Wang L, et al. Dual Roles of Oxidative Stress in Metal Carcinogenesis. J Environ Pathol Toxicol Oncol 2017;36:345-76. [Crossref] [PubMed]
- Zhu T, Yao Q, Wang W, et al. iNOS Induces Vascular Endothelial Cell Migration and Apoptosis Via Autophagy in Ischemia/Reperfusion Injury. Cell Physiol Biochem 2016;38:1575-88. [Crossref] [PubMed]
- Papadakis M, Hadley G, Xilouri M, et al. Tsc1 (hamartin) confers neuroprotection against ischemia by inducing autophagy. Nat Med 2013;19:351-7. [Crossref] [PubMed]
- Zhou H, Wang J, Jiang J, et al. N-acetyl-serotonin offers neuroprotection through inhibiting mitochondrial death pathways and autophagic activation in experimental models of ischemic injury. J Neurosci 2014;34:2967-78. [Crossref] [PubMed]
- Jain A, Lamark T, Sjøttem E, et al. p62/SQSTM1 is a target gene for transcription factor NRF2 and creates a positive feedback loop by inducing antioxidant response element-driven gene transcription. J Biol Chem 2010;285:22576-91. [Crossref] [PubMed]
- Jaaks P, Coker EA, Vis DJ, et al. Effective drug combinations in breast, colon and pancreatic cancer cells. Nature 2022;603:166-73. [Crossref] [PubMed]
(English Language Editor: C. Betlazar-Maseh)