Dosimetry for photobiomodulation therapy: response to Sommers et al.
In a recent article by Sommer et al. in Annals of Translational Medicine, the authors make several key comments on our recent article in Scientific Reports entitled “Molecular pathway of near infrared laser phototoxicity involves ATF-4 orchestrated ER stress” (1). Firstly, we would like to thank the authors for their laudatory, constructive comments, specifically appreciating the rigorous effort and potential impact of our work. However, this editorial also raised a few key issues regarding methodology and interpretations in our study. We feel these points could be further clarified for the increasingly enthusiastic audience following nuances of photobiomodulation (PBM) therapy.
Motivation for this study in reference to prior studies
Given our understanding of the biphasic effects of the Arndt-Schulz law evident in PBM, the two prior studies cited in the editorial are clearly relevant to this study but appear to straddle two distinct dose realms of biophotonics treatments (Figure 1). The Demidova-Rice et al. paper noted mice wound promoting effects at lower laser (810 nm) doses but a negative impact on healing at higher laser fluences at 50 J/cm2 (2). The study by Joensen et al. was performed in human volunteers with an 810 nm laser and assessed discomfort due to thermal damage in a laser dose escalation study (3). It is interesting to note that their treatment parameters (laser irradiance ~5.2 W/cm2) leading to a significant increase in temperature approximates the phototoxic doses (~5.2–5.4 W/cm2) noted in our study as well (Table 1). The authors distinctly state that subjects with darker skin tones were more susceptible to thermal effect but there was little effort to objectively quantify skin photoabsorption. We made a concerted effort to distinctly define melanin scores and further noted that despite a syngeneic mouse strain used in this study, there is significant variation in melanin scores that had potent implications on laser dosing (Supplementary Figure 2a-c in Khan et al.). Nonetheless, both these studies are clearly relevant to our current study and we thank the authors for pointing them out.
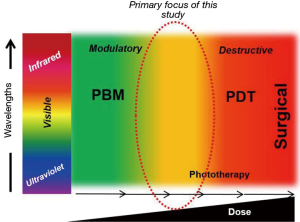
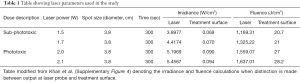
Full table
Therapeutic and damage photobiological mechanisms
As indicated in the editorial, there has been a growing understanding of molecular mechanisms of PBM, largely focused on intracellular targets especially the mitochondria. We recently demonstrated an extracellular pathway involving activation of latent TGF-β1 via a redox-dependent conformational change (4). In our attempts for clinical translation to human therapy, the current study was focused on outlining the transition threshold where the increasing photonic dose demonstrates a transition from beneficial to detrimental (Figure 1). We demonstrate in this study that simply monitoring surface temperature is a very potent real-time clinical biomarker to ensure treatment safety. We do believe future efforts to assess real-time tissue ROS levels offer another attractive avenue to further develop safer therapies. This study demonstrates the precise molecular pathway involved in near-infrared laser phototoxicity (Figure 2). Given the ubiquitous nature of cell stress damage response, the specificity of ATF-4 in mediating phototoxicity is striking indeed (5). One of the major implication of this observation is that low amounts of PBM induced-cell stress resulting in protein turnover (misfolding and/or degradation via autophagy) coordinated by ATF-4 can be clearly beneficial, helping reinvigorate the overall healing responses in a process we term Resynchronization (6). ATF-4 has discrete effects in various cell types and its role in PBM pathophysiological contexts needs further investigation (7). Nonetheless, a major implication of our study is the utility of assessing ATF-4 levels as a molecular biomarker to develop safe and effective clinical PBM regimens.
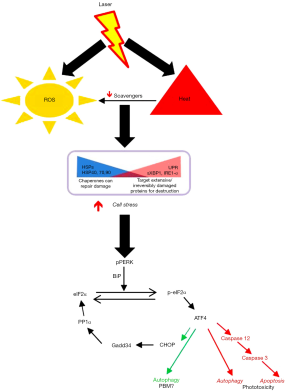
Clinical photobiomodulation (PBM) treatment protocols
Since its early inception in the 1960s with the invention of lasers, the field of low level light/laser therapy (LLLT) or PBM therapy has been plagued with inconsistencies in clinical outcomes. This appears to be due to two major variables namely, first—the complexity of the biophotonics interactions with biological systems and second—a lack of understanding of precise molecular mechanisms mediating its therapeutic responses (6). There are significant issues with current practices of reporting PBM dosing and delivery. As pointed out in the editorial, a majority of the PBM literature reports irradiance (laser irradiance) as laser power over area at laser probe tip (beam diameter at aperture) (Figure 3). This is an inaccurate and unfortunate practice as the actual delivery of photonic energy is on a treatment surface (treatment surface irradiance) wherein a rapid loss of power occurs (inverse square law). In our study we assessed the power density as treatment surface irradiance to perform in vitro assays that were carefully standardized for rigorous reproducibility (as reported in Table 1 and Supplementary Table 4 in Khan et al.). We thank the authors for raising this critical point of laser dosimetry as the listed phototoxic dose in our publication could be misleading if inaccurately interpreted. In retrospect, a new table has been generated in this response that attempts to discretely define differences of conventional dosimetry (Table 1). As noted in this analysis, there is about 100-fold difference in the laser power calculations when considering laser irradiance versus the actual treatment surface irradiances. This is clearly a massive difference in dosing calculations that is likely contributing to irregularities and non-reproducibility in PBM clinical dosing.
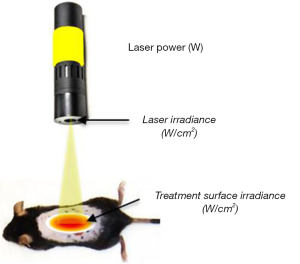
This situation is even more complicated for PBM in vivo mice studies as highlighted in our mice study. While we standardized laser treatment surface irradiance of 1 W/cm2 and maintained surface temperature at 45 °C as a dynamic readout to switch power on or off as this varied greatly with the melanin scores. For higher temperatures, this was still not adequate to prevent instant thermal damage and the laser probe had to be moved in a continuous, circular motion (Supplementary Video 1 in Khan et al.). This clinical motion is another major variable introduced into PBM clinical delivery regimens that is currently poorly documented. These probe movements during treatment is often employed by PBM practitioners to both cover larger areas of treatment (especially with a small laser probe spot size) and prevent inadvertent thermal damage. We strongly advocate reporting treatment surface irradiance and surface temperature, when available, in future PBM studies to aid in robust future reproducibility of specific treatment protocols.
Safety of lasers wavelengths used for photobiomodulation (PBM) therapy
A major discrepancy highlighted in the editorial is the demonstration of DNA damage following laser treatments in another study (8). Using a comprehensive battery of tests including Ames test, Plasmid cleavage, Abasic sites, γ-H2AX expression and gene expression arrays for DNA damage, we have unequivocally noted the non-mutagenic and non-genotoxic nature of photonic devices used for PBM therapy. The study by Sergio et al. demonstrating DNA fragmentation with a modified comet assay does not denote mutagenesis but is an indicator of routine cell death. This is similar to cell death TUNEL assays observed at phototoxic doses in our study (Supplementary Figure 3g in Khan et al.). While data on maximal permissible laser dosing for the eye and skin have been clearly established (ANSI Z136), we believe our study is the first description of the precise molecular mechanism mediating phototoxic laser dose responses (9).
Future directions
PBM therapy has over six decades of research from clinical and laboratory studies demonstrating positive, but often subtle, beneficial effects. Besides the two technical issues (clinical delivery and biological mechanisms) that need to be addressed rigorously, a major deterrent is the sheer breadth of PBM clinical applications. These need to be carefully explored with respect to anatomical and pathophysiological (disease-specific) scenarios. As the editorial eloquently point out, there is significant ongoing progress and exciting advances in the field but dialogues, like these, on standardization and rigorous analyses are invaluable to increased clarity and driving critical progress in the field, moving PBM therapy towards mainstream legitimacy (10).
Acknowledgements
None.
Footnote
Conflicts of Interest: The authors have no conflicts of interest to declare.
*Current address: National Cancer Institute, NIH, Bethesda, Maryland, USA.
References
- Khan I, Tang E, Arany P. Molecular pathway of near-infrared laser phototoxicity involves ATF-4 orchestrated ER stress. Sci Rep 2015;5:10581. [Crossref] [PubMed]
- Demidova-Rice TN, Salomatina EV, Yaroslavsky AN, et al. Low-level light stimulates excisional wound healing in mice. Lasers Surg Med 2007;39:706-15. [Crossref] [PubMed]
- Joensen J, Demmink JH, Johnson MI, et al. The thermal effects of therapeutic lasers with 810 and 904 nm wavelengths on human skin. Photomed Laser Surg 2011;29:145-53. [Crossref] [PubMed]
- Arany PR, Cho A, Hunt TD, et al. Photoactivation of endogenous latent transforming growth factor-β1 directs dental stem cell differentiation for regeneration. Sci Transl Med 2014;6:238ra69. [Crossref] [PubMed]
- Rutkowski DT, Kaufman RJ. All roads lead to ATF4. Dev Cell 2003;4:442-4. [Crossref] [PubMed]
- Arany PR. Craniofacial Wound Healing with Photobiomodulation Therapy: New Insights and Current Challenges. J Dent Res 2016. [Epub ahead of print]. [Crossref] [PubMed]
- Harding HP, Zhang Y, Zeng H, et al. An integrated stress response regulates amino acid metabolism and resistance to oxidative stress. Mol Cell 2003;11:619-33. [Crossref] [PubMed]
- Sergio LP, Silva AP, Amorim PF, et al. DNA damage in blood cells exposed to low-level lasers. Lasers Surg Med 2015;47:361-8. [Crossref] [PubMed]
- American National Standards Institute. (04/20/2016); Available online: www.ansi.org
- Tunér J, Jenkins PA. Parameter Reproducibility in Photobiomodulation. Photomed Laser Surg 2016;34:91-2. [Crossref] [PubMed]