Research hotspots and development trends of microRNA in ischemia-reperfusion: network analysis of academic journals oriented by bibliometric and visualization
Highlight box
Key findings
• MiRNA is a hot spot in the study of IR. It participates in the occurrence of IR.
What is known and what is new?
• MiRNA can regulate apoptosis, inflammation, oxidative stress, and autophagy during IR.
• MiRNA regulation of apoptosis, inflammation, oxidative stress, and autophagy induced by IR involves a variety of target genes and signal pathways. Recent research focuses on the regulatory network of miRNA and lncRNA.
What is the implication, and what should change now?
• Although miRNA participates in the occurrence of IR, further research is needed for its true clinical application. The future research focus of miRNA in IR field may be the regulatory network of miRNA and other non-coding genes.
Introduction
Ischemia-reperfusion (IR) injury refers to a syndrome in which the blood flow recovers after a period of organ or tissue ischemia, with the degree of tissue damage further aggravated and organ function further deteriorated than in ischemia. IR is common in clinical practice following ischemic stroke, hemorrhagic shock, organ transplantation, and cardiopulmonary resuscitation, and it increases mortality. Oxidative stress, energy metabolism, mitochondrial dysfunction, and inflammatory response are the factors leading to IR-related damage.
MicroRNA (miRNA) is a kind of single-stranded noncoding RNA with an average of 22 nucleotides. It induces messenger RNA (mRNA) degradation or inhibition by binding to the 3'-untranslated region (3'-UTR) of target mRNA, and participates in the post transcriptional regulation of gene expression. MiRNA has many functions, including regulating cell differentiation, proliferation, and apoptosis.
The number of studies on miRNA in IR has increased. It was also found that miRNA was involved in critical signaling pathways of apoptosis, oxidative stress, inflammatory by IR (1). In this study, CiteSpace visual analysis software (2,3) was used to visually analyze research on miRNA in IR in the last 10 years, and to explore the topic’s research hotspots, trends, and frontiers, to provide valuable ideas for further research.
Methods
Literature search
The Web of Science database was used as the retrieval library. The search query was TS = (MicroRNA OR Micro RNA OR miRNA OR mir) AND TS = (Reperfusion Injury OR Reperfusion Damage OR Ischemia-Reperfusion Injury OR Injury, Ischemia-Reperfusion OR Ischemia-Reperfusion). The language of the search was English, the document type was article, the index used was SCI-Expanded, and the time span was 2012–2022. The search was performed on 2 May 2020. A total of 2020 relevant literature items were retrieved.
The titles and abstracts of the retrieved articles were independently screened by two reviewers from the research group to exclude literature unrelated to IR and miRNA. A third reviewer screened the literature in the case of difference of opinion between the first two reviewers. Finally, 1,518 published items were included.
Study methods
A total of 1,518 document records were exported in the plain text file format of “Full Records and Cited References”. Each document record contained the title, author, organization, keywords, abstract, references, citations, and other materials.
We imported the plain text file of the literature collection into CiteSpace v5.8 R3 software for visual analysis. The software options were set as follows: timespan: 2012–2022 (slice length =1) and selection criteria: top 50 per slice. The remaining parameter settings remained at the default values.
Observation indicators
By analyzing the authors, institutions, countries, journals, co-citations, and keywords of 1,518 literature items, we were able to further analyze the trend changes, hot spots, and current status of research on miRNA and IR.
Results
Publication of articles
Number of documents published in 10 years
As shown in Figure 1, from 2012 to 2014, relatively few studies were published on miRNA in the field of IR, with no more than 50 papers released per year. However, since 2015, with the gradual deepening of miRNA research, the number of published papers has gradually increased. Rapid growth was seen after 2019, with high numbers of publications persisting in 2020 and 2021, indicating that research on miRNA in the field of IR has been receiving increasing and continuous attention.
Analysis of authors, research institutions, and countries
Table 1 shows the top 5 authors of research on miRNA and IR in the past 10 years, indicating that they are relatively active in this research field. There are many connections between Mingyu Liang, Jie Teng, and Xiaoqian Ding, indicating cooperative relationships among them (Figure 2).
Table 1
Frequency | Author | Institution | Country |
---|---|---|---|
10 | Mingyu Liang | Medical College of Wisconsin | USA |
8 | Dan Wang | China Med Univ | China |
6 | Yang Li | China Med Univ | China |
6 | Jie Teng | Fudan University | China |
6 | Xiaoqiang Ding | Fudan University | China |
MiRNA, microRNA; IR, ischemia-reperfusion.
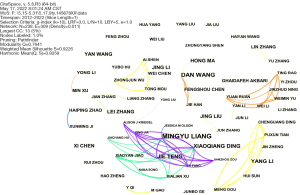
We chose institution as the node type for co-occurrence analysis. Figure 3 shows that the institutions involved in research on miRNA in IR were widely distributed. It can be seen from the connection in the figure that there are cooperative relationships between research institutions. The top 5 institutions are university research institutions from China, including Fudan University, Nanjing Medical University, Shanghai Jiaotong University, Zhengzhou University, and China Medical University. Their publication frequencies were 48, 46, 46, 44, and 39, respectively. The size of intermediary centrality reflected the importance of the node to the whole network. The top 3 intermediaries were Shanghai Jiaotong University (0.61), Nanjing Medical University (0.47), and Tongji University (0.44).
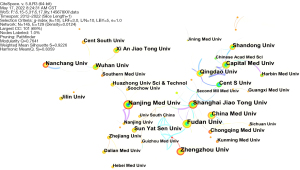
Figure 4 shows the top 5 countries (or regions) with the largest number of published articles on miRNA in the field of IR: China (1,249 publications), the United States (141 publications), Canada (38 publications), Taiwan (19 publications), and South Korea (16 publications). China was found to be a major role-player in the study of miRNA and IR.
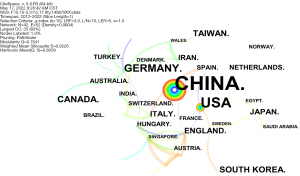
Analysis of journals and literature citation
Analysis of the citation of journals
As can be seen from Figure 5, in the past 10 years, the top 5 journals cited were PLoS One (766 times), Circulation (559 times), Biochemical and Biophysical Research Communications (547 times), Circulation Research (536 times), and Proceedings of the National Academy of Sciences (USA) (524 times). This suggests that these 5 journals have published important articles on miRNA and IR.
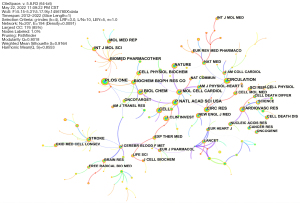
Analysis of literature citation
We summarized the references cited in the 1,518 articles. Table 2 shows the top 10 cited articles, of which 6 were reviews and 4 were research reports. The content included the detection of miRNA, miRNA and cerebral IR, cardiac IR, and renal IR.
Table 2
Ranking | Total frequency of citations | Author | Title | Citation | Main content |
---|---|---|---|---|---|
1 | 30 | Yu Di (4) | MicroRNAs expression and function in cerebral ischemia reperfusion injury (review) | J Mol Neurosci. 2014;53(2):242-250 | MiRNAs (miR-15a/b, miR-21, miR-29b/c, miR-124, miR-145, miR-181, miR-200 family, miR-338, miR-422a, miR-497, and miR let 7 family) in cerebral ischemia reperfusion injury |
2 | 25 | Zhi-Xing Fan (1) | The role of microRNAs in regulating myocardial ischemia reperfusion injury (review) | Saudi Med J. 2015;36(7):787-793 | MiRNAs in myocardial IR injury |
3 | 25 | Thomas X. Lu (5) | MicroRNA (review) | J Allergy Clin Immunol. 2018;141(4):1202-1207 | The major technological platforms for miRNA |
4 | 24 | Meng-Yu Wu (6) | Current mechanistic concepts in ischemia and reperfusion injury (review) | Cell Physiol Biochem. 2018;46(4):1650-1667 | Highlight the latest mechanistic insights into reperfusion-injury-induced cell death |
5 | 24 | Jue Wang (7) | Long non-coding RNA H19 induces cerebral ischemia reperfusion injury via activation of autophagy | Aging Dis. 2017;8(1):71-84 | The role of lncRNA H19 and H19 gene variation in cerebral IR injury |
6 | 24 | Xiao-Ping Ren (8) | MicroRNA-320 is involved in the regulation of cardiac ischemia/reperfusion injury by targeting heat-shock protein 20 | Circulation. 2009;119(17):2357-2366 | MiR-320 is involved in the regulation of IR-induced cardiac injury |
7 | 23 | Xiaohong Wang (9) | MicroRNA-494 targeting both proapoptotic and antiapoptotic proteins protects against ischemia/reperfusion-induced cardiac injury | Circulation. 2010;122(13):1308-1318 | MiR-494 is involved in the cardioprotective effects against IR-induced injury |
8 | 23 | Pouran Makhdoumi (10) | MicroRNAs regulate mitochondrial apoptotic pathway in myocardial ischemia-reperfusion-injury (review) | Biomed Pharmacother. 2016;84:1635-1644 | Molecular mechanisms of miRNA-regulated mitochondrial functions during ischemia/reperfusion injury |
9 | 22 | Jonathan G. Godwin (11) | Identification of a microRNA signature of renal ischemia reperfusion injury | Proc Natl Acad Sci U S A. 2010;107(32):14339-14344 | Identification of a miRNA signature of renal ischemia reperfusion injury |
10 | 21 | Yumei Ye (12) | The role of microRNA in modulating myocardial ischemia-reperfusion injury (review) | Physiol Genomics. 2011;43(10):534-542 | MiR-1, miR-21, miR-29, miR-92a, miR-133, miR-199a, and miR-320 in IR injury and/or remodeling after myocardial infarction |
MiRNA, microRNA; IR, ischemia-reperfusion; lncRNA, long noncoding RNA.
Research hotspots and evolution trends of miRNA in the field of IR
Analysis of keyword co-occurrence
We selected “keyword” as the node type. Based on a time slice of 1 year, the information of the top 50 for each time slice and the map of keyword co-occurrence were obtained (Figure 6). The top 15 keywords were expression (451 times), IR injury (438 times), apoptosis (356 times), miRNA (284 times), protect (273 times), activation (214 times), cell (188 times), mechanism (176 times), reperfusion injury (166 times), heart (155 times), injury (147 times), oxidative stress (147 times), infection (137 times), disease (124 times), and promotion (122 times).
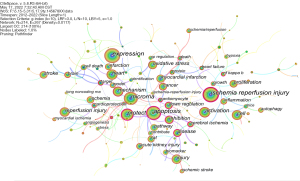
Analysis of keyword citation bursts
It can be seen from Figure 7 that the early keyword citation bursts were mainly in the aspect of cardiac IR. With the deepening of research, research hotspots were further diversified, with recent hotspot focused on long noncoding RNA (lncRNA), exosomes, kidney, and cerebral IR injury. Through the evolution of keyword hotspots, it can be seen that many studies have been carried out on lncRNA and exosomes in gene research in recent years. It is worth noting that among the highlighted keyword references in Figure 7, miRNA-21 ranks 14th and is the only miRNA that appears on this ranking.
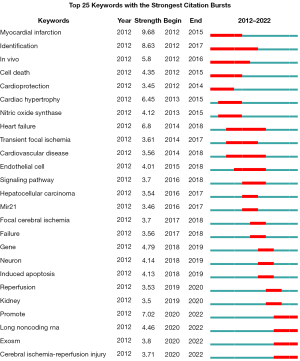
Keyword clustering graph
By using a log-likelihood ratio algorithm to cluster and classify closely related keywords, a keyword clustering graph was obtained (Figure 8). The clustering map of keywords summarized the research concerns in this field. As shown in Figure 8, the keywords were aggregated into 9 clusters: #0 myocardial information, #1 oxidative stress, #2 cerebral ischemia/reperfusion input, #3 reperfusion input, #4 ischemic stroke, #5 informatory response, #6 stroke, #7 acute childhood input, and #8 ischemia/reperfusion. The larger the number of the cluster number, the less keywords were included in the cluster. On the contrary, the smaller the number, the more keywords were included in the cluster. The lines between the internal nodes of the tag title cluster represent the co-occurrence relationship. The more lines, the higher the co-occurrence degree between the keywords in this field. There were more internal connections in clusters #1, #2, #3, and #6, suggesting a higher degree of co-occurrence between keywords in the modified cluster.
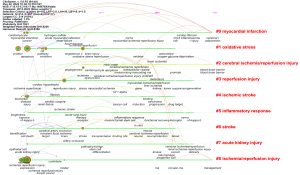
Discussion
In 1993, Lin-4 RNA was found in Caenorhabditis elegans. After further to elucidate the expression and function of the miRNA in the early 21st century, there was a boom in miRNA research, including research on miRNA in the field of IR (1).
We used CiteSpace to visually analyze 1,518 studies on miRNA and IR published between from 2012 and 2022. Over the past 10 years, the volume of this research has gradually increased, especially since 2019, with the number of relevant articles published reaching 300 per year. Although the institutions involved in the research are mainly from medical universities in China, relevant studies have also been published in the United Kingdom, Australia, Japan, South Korea, India, Germany, Switzerland, and other countries. The titles of the top 3 cited articles are “MicroRNA expression and function in cerebral ischemia reperfusion injury” (4), “The role of microRNAs in regulating myocardial ischemia reperfusion injury” (1), and “MicroRNA” (5). This demonstrates that organ function damage caused by IR has attracted the attention of many academics. This is similarly suggested by the keyword hotspot and keyword citation results shown in Figures 6-8.
In recent years, lncRNA has been a research hotspot in the field of noncoding RNA. Studies have found that lncRNA TTTY15 has a role in inflammation, oxidative stress, and apoptosis of myocardial IR by targeting miR-98 (13), miR-186 (14), miR-374 (15), and miR-455 (16). LncRNA Ttc3-209 promotes apoptosis of retinal ganglion cells in retinal IR injury by targeting miR-484 (17). There are similar studies on hepatic IR, renal IR, and cerebral IR. LncRNA HOTAIR regulates autophagy in liver IR injury through miR-20b (18). LncRNA TUG can promote PTEN expression, autophagy, and apoptosis by competitively combining miR-29, thereby aggravating acute renal injury in rats treated with ischemia-perfusion (19). LncRNA C2dat2 promotes autophagy and apoptosis in cerebral IR injury through the miR-30d-5p/DDIT4/mTOR axis (20).
In the last 3 years, the relationship between miRNA in exosomes and IR has attracted increasing attention from researchers (Figure 7). Their results have found that the miRNA of the exosomes of various cells, such as tubular epithelial cells (21), myocardial fibroblasts (22), bone marrow mesenchymal stem cells (23), human urine-derived stem cells (24), macrophages (25), cochlear spiral ganglion progenitor cells (26), and human umbilical cord mesenchymal stem cells (hUCMSCs) (27) are involved in the occurrence and development of IR.
IR occurs in many organs. The research on miRNA and IR involves not only the heart, liver, kidney, and brain, but also the spinal cord (28), lung (29), intestine (30), and other organs. However, the keyword clustering network map in Figure 8 shows only miRNA and myocardial infarction IR, miRNA and brain IR, and miRNA and kidney IR in the clustering range, suggesting that the heart, brain, and kidney are the most common organs for IR, generating more interest from researchers.
Oxidative stress (31), inflammatory response (32), microvascular embolism, calcium overload, autophagy, and apoptosis are involved in the pathogenesis of IR injury (6), and miRNA research that involves IR also involves the above mechanisms. However, from the keyword cluster, we found that the research focused more on oxidative stress and inflammatory response. In renal IR, miR-27a-3p simulates the oxidative stress induced by hypoxia/reoxygenation (H/R) in vitro by increasing reactive oxygen species (ROS) levels, reducing Grb2 expression, and down-regulating PI3K-Akt signals (33). In the intestinal IR model, when miR-23a-5p was inhibited, the cell damage caused by H/R was reduced and the ROS level was reduced (30). As demonstrated in in vivo and in vitro experiments of myocardial ischemia, inhibition of miR-218-5p can reduce the activity of lactate dehydrogenase (LDH) and the levels of ROS, malondialdehyde (MDA), and superoxide dismutase, as well as tumor necrosis factor-α (TNF-α), interleukin-1β (IL-1β), and IL-6 expression (34). Similarly, in another myocardial IR model, down-regulation of miR-15b-5p expression improved infarct size and apoptosis after myocardial IR injury in mice, and reduced MDA in myocardial tissue and IL-6 and TNF-α content in the blood (35). TNF-α, IL-1β, and IL-6 are inflammatory factors. More studies have found that miRNA affects the inflammatory response induced by IR. MiR-455-5p reduces the level of C-C chemokine receptor type 5 mRNA and protein, inhibits microglia activation, and reduces TNF-α and IL-1β (36). In renal IR injury model, overexpression of miR-194 targeting Ras homology enriched in brain (Rheb) inhibits the secretion of proinflammatory cytokines, including IL-6, IL-1β, and TNF-α (37). By targeting CREB binding protein (CBP), miR-22 regulates the CBP/AP-1 pathway to reduce TNF-α and IL-6 during myocardial IR injury (38).
In the keyword citation bursts (Figure 7), miRNA-21 not only ranks 14th, but is also the only miRNA that appears on this ranking. It involves the intestines, heart, kidney, liver, spinal cord, lung, and other organs, especially in cardiac IR. The mediated signal pathways between miRNA-21 and IR are Akt/Bcl-2/Bax (39), PTEN/AKT (40,41), SMAD2 (42), CPEG3 (43), AKT/mTOR (44), PTEN/AKT/mTOR (45), and TLR4/NF-κB (46). Among these, most research was focused on the PTEN/AKT signaling pathway. This suggests that miRNA-21 and the PTEN/AKT signaling pathway are the latest and future research targets in the field of miRNA and IR.
In the study of miRNA in IR, different miRNAs have different target genes. MiR-429 protects neurons from oxygen-glucose deprivation and reoxygenation (OGD/R)-induced damage through target GATA-binding protein 4 (GATA4) (47). MiR-24-3p targets Kelch-like ECH-associated protein 1 (Keap1) gene and protects myocardial cells from IR injury by activating Nrf2-Keap1 pathway (48). MiR-34c targets Toll-like receptor 7 (TLR7) to down regulate NF-κB/MAPK axis and alleviated nerve injury caused by I/R (49). The up regulation of miR-30e can alleviate myocardial IR injury through targeted inhibition of SRY-related high mobility group-box gene 9 (SOX9) (50). MiR-374a-5p negatively regulates the expression of mitogen-activated protein kinase 6 (MAPK6), which can improve myocardial cell damage in ischemic models in vivo and in vitro (51). It can be seen that even for the same organ of IR, there are different miRNAs, different miRNA target genes and different pathway mechanisms, which indicates that the regulation and interaction of miRNAs are complex. Their operation may not only affect targeted genes, but also affect other unrelated mRNA. In the IR field, in addition to the role of a single miRNA, more detailed and comprehensive studies are needed to clarify the complexity of the regulatory network of miRNA, protein coding genes and other non-coding genes (52). For example, miRNA-miRNA, circular RNA (circRNA)-miRNA-mRNA, circRNA-lncRNA-miRNA-mRNA network may be the research hotspot of miRNA in IR field in the future.
MiRNA plays a role in IR. However, few of them enter clinical transformation. In the future clinical aspect, we hope to further study whether circulating miRNA can be used as a potential marker for IR diagnosis and prognosis. The treatment of miRNA is promising. But many challenges related to miRNA delivery limit its efficacy (53). With the deepening of research, we believe that treatment methods based on miRNA or using miRNA mimics or miRNA inhibitors can be applied to clinical treatment, including improving medical materials, such as miRNA mimics or miRNA inhibitors microspheres can be considered in the coating of vascular stents implanted during acute myocardial infarction or acute ischemic stroke to reduce the occurrence of IR.
Conclusions
Through our visual analysis, it was shown that miRNA in IR has attracted considerable research attention. Many miRNAs, target genes and signal transduction pathways are involved in the process of IR. This poses a challenge for the prospect of miRNA as a clinical intervention target for IR, and further research is needed.
Acknowledgments
Funding: This work was supported by a grant from the Hainan Clinical Research Center Project (No. LCYX202207).
Footnote
Conflicts of Interest: All authors have completed the ICMJE uniform disclosure form (available at https://atm.amegroups.com/article/view/10.21037/atm-22-5677/coif). The authors have no conflicts of interest to declare.
Ethical Statement: The authors are accountable for all aspects of the work in ensuring that questions related to the accuracy or integrity of any part of the work are appropriately investigated and resolved.
Open Access Statement: This is an Open Access article distributed in accordance with the Creative Commons Attribution-NonCommercial-NoDerivs 4.0 International License (CC BY-NC-ND 4.0), which permits the non-commercial replication and distribution of the article with the strict proviso that no changes or edits are made and the original work is properly cited (including links to both the formal publication through the relevant DOI and the license). See: https://creativecommons.org/licenses/by-nc-nd/4.0/.
References
- Fan ZX, Yang J. The role of microRNAs in regulating myocardial ischemia reperfusion injury. Saudi Med J 2015;36:787-93. [Crossref] [PubMed]
- Chen C. Searching for intellectual turning points: progressive knowledge domain visualization. Proc Natl Acad Sci U S A 2004;101:5303-10. [Crossref] [PubMed]
- Chen C. CiteSpace II: Detecting and visualizing emerging trends and transient patterns in scientific literature. J Am Soc Inf Sci Technol 2006;57:359-77.
- Di Y, Lei Y, Yu F, et al. MicroRNAs expression and function in cerebral ischemia reperfusion injury. J Mol Neurosci 2014;53:242-50. [Crossref] [PubMed]
- Lu TX, Rothenberg ME. MicroRNA. J Allergy Clin Immunol 2018;141:1202-7. [Crossref] [PubMed]
- Wu MY, Yiang GT, Liao WT, et al. Current Mechanistic Concepts in Ischemia and Reperfusion Injury. Cell Physiol Biochem 2018;46:1650-67. [Crossref] [PubMed]
- Wang J, Cao B, Han D, et al. Long Non-coding RNA H19 Induces Cerebral Ischemia Reperfusion Injury via Activation of Autophagy. Aging Dis 2017;8:71-84. [Crossref] [PubMed]
- Ren XP, Wu J, Wang X, et al. MicroRNA-320 is involved in the regulation of cardiac ischemia/reperfusion injury by targeting heat-shock protein 20. Circulation 2009;119:2357-66. [Crossref] [PubMed]
- Wang X, Zhang X, Ren XP, et al. MicroRNA-494 targeting both proapoptotic and antiapoptotic proteins protects against ischemia/reperfusion-induced cardiac injury. Circulation 2010;122:1308-18. [Crossref] [PubMed]
- Makhdoumi P, Roohbakhsh A, Karimi G. MicroRNAs regulate mitochondrial apoptotic pathway in myocardial ischemia-reperfusion-injury. Biomed Pharmacother 2016;84:1635-44. [Crossref] [PubMed]
- Godwin JG, Ge X, Stephan K, et al. Identification of a microRNA signature of renal ischemia reperfusion injury. Proc Natl Acad Sci U S A 2010;107:14339-44. [Crossref] [PubMed]
- Ye Y, Perez-Polo JR, Qian J, et al. The role of microRNA in modulating myocardial ischemia-reperfusion injury. Physiol Genomics 2011;43:534-42. [Crossref] [PubMed]
- Ma R, Gao L, Liu Y, et al. LncRNA TTTY15 knockdown alleviates H(2)O(2)-stimulated myocardial cell injury by regulating the miR-98-5p/CRP pathway. Mol Cell Biochem 2021;476:81-92. [Crossref] [PubMed]
- Zheng J, Zhuo YY, Zhang C, et al. LncRNA TTTY15 regulates hypoxia-induced vascular endothelial cell injury via targeting miR-186-5p in cardiovascular disease. Eur Rev Med Pharmacol Sci 2020;24:3293-301. [Crossref] [PubMed]
- Chen YQ, Yang X, Xu W, et al. Knockdown of lncRNA TTTY15 alleviates myocardial ischemia-reperfusion injury through the miR-374a-5p/FOXO1 axis. IUBMB Life 2021;73:273-85. [Crossref] [PubMed]
- Huang S, Tao W, Guo Z, et al. Suppression of long noncoding RNA TTTY15 attenuates hypoxia-induced cardiomyocytes injury by targeting miR-455-5p. Gene 2019;701:1-8. [Crossref] [PubMed]
- Zhang R, Feng Y, Lu J, et al. lncRNA Ttc3-209 Promotes the Apoptosis of Retinal Ganglion Cells in Retinal Ischemia Reperfusion Injury by Targeting the miR-484/Wnt8a Axis. Invest Ophthalmol Vis Sci 2021;62:13. [Crossref] [PubMed]
- Tang B, Bao N, He G, et al. Long noncoding RNA HOTAIR regulates autophagy via the miR-20b-5p/ATG7 axis in hepatic ischemia/reperfusion injury. Gene 2019;686:56-62. [Crossref] [PubMed]
- Xu Z, Huang X, Lin Q, et al. Long non-coding RNA TUG1 knockdown promotes autophagy and improves acute renal injury in ischemia-reperfusion-treated rats by binding to microRNA-29 to silence PTEN. BMC Nephrol 2021;22:288. [Crossref] [PubMed]
- Xu Q, Guohui M, Li D, et al. lncRNA C2dat2 facilitates autophagy and apoptosis via the miR-30d-5p/DDIT4/mTOR axis in cerebral ischemia-reperfusion injury. Aging (Albany NY) 2021;13:11315-35. [Crossref] [PubMed]
- Ding C, Zheng J, Wang B, et al. Exosomal MicroRNA-374b-5p From Tubular Epithelial Cells Promoted M1 Macrophages Activation and Worsened Renal Ischemia/Reperfusion Injury. Front Cell Dev Biol 2020;8:587693. [Crossref] [PubMed]
- Liu N, Xie L, Xiao P, et al. Cardiac fibroblasts secrete exosome microRNA to suppress cardiomyocyte pyroptosis in myocardial ischemia/reperfusion injury. Mol Cell Biochem 2022;477:1249-60. [Crossref] [PubMed]
- Chen Q, Liu Y, Ding X, et al. Bone marrow mesenchymal stem cell-secreted exosomes carrying microRNA-125b protect against myocardial ischemia reperfusion injury via targeting SIRT7. Mol Cell Biochem 2020;465:103-14. [Crossref] [PubMed]
- Zhang Y, Wang J, Yang B, et al. Transfer of MicroRNA-216a-5p From Exosomes Secreted by Human Urine-Derived Stem Cells Reduces Renal Ischemia/Reperfusion Injury. Front Cell Dev Biol 2020;8:610587. [Crossref] [PubMed]
- Dai Y, Wang S, Chang S, et al. M2 macrophage-derived exosomes carry microRNA-148a to alleviate myocardial ischemia/reperfusion injury via inhibiting TXNIP and the TLR4/NF-κB/NLRP3 inflammasome signaling pathway. J Mol Cell Cardiol 2020;142:65-79. [Crossref] [PubMed]
- Yang T, Cai C, Peng A, et al. Exosomes derived from cochlear spiral ganglion progenitor cells prevent cochlea damage from ischemia-reperfusion injury via inhibiting the inflammatory process. Cell Tissue Res 2021;386:239-47. [Crossref] [PubMed]
- Diao L, Zhang Q. Transfer of lncRNA UCA1 by hUCMSCs-derived exosomes protects against hypoxia/reoxygenation injury through impairing miR-143-targeted degradation of Bcl-2. Aging (Albany NY) 2021;13:5967-85. [Crossref] [PubMed]
- Li JA, Zan CF, Xia P, et al. Key genes expressed in different stages of spinal cord ischemia/reperfusion injury. Neural Regen Res 2016;11:1824-9. [Crossref] [PubMed]
- Li W, Ma K, Zhang S, et al. Pulmonary microRNA expression profiling in an immature piglet model of cardiopulmonary bypass-induced acute lung injury. Artif Organs 2015;39:327-35. [Crossref] [PubMed]
- Li LX, Yin LH, Gao M, et al. MiR-23a-5p exacerbates intestinal ischemia-reperfusion injury by promoting oxidative stress via targeting PPAR alpha. Biochem Pharmacol 2020;180:114194. [Crossref] [PubMed]
- Chazelas P, Steichen C, Favreau F, et al. Oxidative Stress Evaluation in Ischemia Reperfusion Models: Characteristics, Limits and Perspectives. Int J Mol Sci 2021;22:2366. [Crossref] [PubMed]
- Schofield ZV, Woodruff TM, Halai R, et al. Neutrophils--a key component of ischemia-reperfusion injury. Shock 2013;40:463-70. [Crossref] [PubMed]
- Zhao XR, Zhang Z, Gao M, et al. MicroRNA-27a-3p aggravates renal ischemia/reperfusion injury by promoting oxidative stress via targeting growth factor receptor-bound protein 2. Pharmacol Res 2020;155:104718. [Crossref] [PubMed]
- Yang Y, Zhao F, Yuan Z, et al. Inhibition of miR-218-5p reduces myocardial ischemia-reperfusion injury in a Sprague-Dawley rat model by reducing oxidative stress and inflammation through MEF2C/NF-κB pathway. Int Immunopharmacol 2021;101:108299. [Crossref] [PubMed]
- Niu S, Xu L, Yuan Y, et al. Effect of down-regulated miR-15b-5p expression on arrhythmia and myocardial apoptosis after myocardial ischemia reperfusion injury in mice. Biochem Biophys Res Commun 2020;530:54-9. [Crossref] [PubMed]
- Zhang JS, Hou PP, Shao S, et al. microRNA-455-5p alleviates neuroinflammation in cerebral ischemia/reperfusion injury. Neural Regen Res 2022;17:1769-75. [Crossref] [PubMed]
- Shen Y, Zhao Y, Wang L, et al. MicroRNA-194 overexpression protects against hypoxia/reperfusion-induced HK-2 cell injury through direct targeting Rheb. J Cell Biochem 2018; Epub ahead of print. [Crossref]
- Yang J, Chen L, Ding J, et al. Cardioprotective effect of miRNA-22 on hypoxia/reoxygenation induced cardiomyocyte injury in neonatal rats. Gene 2016;579:17-22. [Crossref] [PubMed]
- Ma N, Bai J, Zhang W, et al. Trimetazidine protects against cardiac ischemia/reperfusion injury via effects on cardiac miRNA-21 expression, Akt and the Bcl-2/Bax pathway. Mol Med Rep 2016;14:4216-22. [Crossref] [PubMed]
- Yang Q, Yang K, Li A. microRNA-21 protects against ischemia-reperfusion and hypoxia-reperfusion-induced cardiocyte apoptosis via the phosphatase and tensin homolog/Akt-dependent mechanism. Mol Med Rep 2014;9:2213-20. [Crossref] [PubMed]
- Tu Y, Wan L, Fan Y, et al. Ischemic postconditioning-mediated miRNA-21 protects against cardiac ischemia/reperfusion injury via PTEN/Akt pathway. PLoS One 2013;8:e75872. [Crossref] [PubMed]
- Tian F, Liu G, Fan L, et al. miR-21-3p alleviates neuronal apoptosis during cerebral ischemia-reperfusion injury by targeting SMAD2. Biocell 2021;45:49-56.
- Wang B, Yu P, Lin W, et al. MicroRNA-21-5p Reduces Hypoxia/Reoxygenation-Induced Neuronal Cell Damage through Negative Regulation of CPEB3. Anal Cell Pathol (Amst) 2021;2021:5543212. [Crossref] [PubMed]
- Huang Z, Wu S, Kong F, et al. MicroRNA-21 protects against cardiac hypoxia/reoxygenation injury by inhibiting excessive autophagy in H9c2 cells via the Akt/mTOR pathway. J Cell Mol Med 2017;21:467-74. [Crossref] [PubMed]
- Song N, Zhang T, Xu X, et al. miR-21 Protects Against Ischemia/Reperfusion-Induced Acute Kidney Injury by Preventing Epithelial Cell Apoptosis and Inhibiting Dendritic Cell Maturation. Front Physiol 2018;9:790. [Crossref] [PubMed]
- Zou HB, Sun XF. The mechanism of curcumin post-treatment relieving lung injuries by regulating miR-21/TLR4/NF-κB signalling pathway. J Int Med Res 2020;48:300060520965809. [Crossref] [PubMed]
- Xiao J, Kong R, Hu J. Inhibition of microRNA-429 attenuates oxygen-glucose deprivation/reoxygenation-induced neuronal injury by promoting expression of GATA-binding protein 4. Neuroreport 2018;29:723-30. [Crossref] [PubMed]
- Xiao X, Lu Z, Lin V, et al. MicroRNA miR-24-3p Reduces Apoptosis and Regulates Keap1-Nrf2 Pathway in Mouse Cardiomyocytes Responding to Ischemia/Reperfusion Injury. Oxid Med Cell Longev 2018;2018:7042105. [Crossref] [PubMed]
- Wu W, Liu J, Yang C, et al. Astrocyte-derived exosome-transported microRNA-34c is neuroprotective against cerebral ischemia/reperfusion injury via TLR7 and the NF-κB/MAPK pathways. Brain Res Bull 2020;163:84-94. [Crossref] [PubMed]
- Cheng N, Li L, Wu Y, et al. microRNA-30e up-regulation alleviates myocardial ischemia-reperfusion injury and promotes ventricular remodeling via SOX9 repression. Mol Immunol 2021;130:96-103. [Crossref] [PubMed]
- Huang ZQ, Xu W, Wu JL, et al. MicroRNA-374a protects against myocardial ischemia-reperfusion injury in mice by targeting the MAPK6 pathway. Life Sci 2019;232:116619. [Crossref] [PubMed]
- Mirzadeh Azad F, Arabian M, Maleki M, et al. Small Molecules with Big Impacts on Cardiovascular Diseases. Biochem Genet 2020;58:359-83. [Crossref] [PubMed]
- Ho PTB, Clark IM, Le LTT. MicroRNA-Based Diagnosis and Therapy. Int J Mol Sci 2022;23:7167. [Crossref] [PubMed]
(English Language Editor: J. Jones)