Exercise training combined with alprostadil improves myocardial infarction and coronary microcirculation disorder in aged rats by inhibiting mitogen-activated protein kinase (MAPK) signaling pathway activation
Highlight box
Key findings
• Exercise training combined with ALPR improved MI in elderly rats by inhibiting MAPK signaling, promoting angiogenesis, and increasing metabolism.
What is known and what is new?
• Myocardial infarction (MI), one of the most severe ischemic heart diseases, is the leading cause of cardiovascular morbidity and mortality. Prostaglandins have shown promising effects in treating various cardiovascular diseases;
• Based on existing evidence, we hypothesized that a combination of exercise and drug therapy would improve the treatment outcomes of MI. Therefore, in this study, we aimed to explore the effects of exercise training and ALPR on MI treatment.
What is the implication, and what should change now?
• Our goal was to determine whether their combination could form a new treatment strategy and provide potential evidence for improving the treatment of elderly patients with MI.
Introduction
Myocardial infarction (MI), one of the most severe ischemic heart diseases, is the leading cause of cardiovascular morbidity and mortality (1) and has been reported to affect more than 50 million people worldwide. The incidence of MI is affected by various factors, such as gender, age, atherosclerosis, arterial hypertension, and smoking (2). Globally, MI is the most prevalent cause of morbidity and mortality in the elderly. As a special group of patients, elderly patients are at high risk of suffering from atherosclerotic cardiovascular disease due to the physiological changes induced by age. It has been reported that the incidence of MI increases after age 65 for men and after age 75 for women (3). MI and stroke, major diseases caused by atherosclerosis, may lead to loss of organ function and even death. Many patients with progressive MI succumb to the disease before reaching the hospital for treatment (4). Cardiomyocytes undergo various pathological changes after ischemia, including energy metabolism disturbance, calcium homeostasis disturbance, overload of reactive oxygen species, and cell death (5). The current clinical treatment for MI is focused on its early stage. Specifically, early treatments primarily include improving hypertension and adjusting living habits. The incidence of atherosclerosis-induced MI can be reduced with appropriate lifestyle changes and medical therapy (6). When MI occurs, early reperfusion is the only way to rescue ischemic cardiomyocytes. However, some reversible and irreversible damage to cardiomyocytes will remain. Myocardial injuries that occur at reperfusion include arrhythmias, transient mechanical dysfunction of the heart, microvascular damage, and oxidative stress (OS) response (7). MI is mainly treated with drugs and surgery, but the prognosis remains unsatisfactory (8). A study showed that the levels of YKL-40 and sTNFR1 were associated with poor cardiac function and prognosis (9). Given the large proportion of the elderly in the general population, the primary tasks of public health research are to prevent MI, improve treatment outcomes, and promote recovery after surgery, especially in the elderly.
Prostaglandins have shown promising effects in treating various cardiovascular diseases (10). Alprostadil (ALPR) is a synthetic compound of prostaglandins. Initially, ALPR was mainly adopted to treat cavernous smooth muscle-related disorders (11), but its role in other diseases has also been explored. Tu et al. reported that ALPR treated myocardial fibrosis in rats with diabetes mellitus (DM) by inhibiting the transforming growth factor-β1 (TGF-β1)/Smad2 signaling pathway (12). Zhang et al. reported that ALPR alleviated myocardial ischemia/reperfusion (MI/R) injury by promoting antioxidant activity and endothelial nitric oxide (eNOS) activation in rats (13). The above studies suggest that ALPR has an excellent protective effect on myocardial injury, and there is no obvious adverse reaction (14). Exercise is the preferred choice for most patients in the early treatment and postoperative recovery of MI. Further, since exercise training can reduce the risk of all-cause mortality (15), it has become a cornerstone of cardiac rehabilitation. In addition, abundant evidence has shown that psychosocial stress and emotional distress play an important role in the pathogenesis and progression of major cardiovascular diseases. It has also been reported that appropriate exercise can effectively mobilize positive emotions in patients, thereby reducing the risk of MI recurrence and concurrently inhibiting the development of depression (16). A study has also shown that the risk of early death in people with low levels of negative emotional distress has declined by 63% compared to people with high levels of emotional distress (17). However, there is currently no report on the role of exercise or ALPR in elderly patients with MI.
Based on existing evidence, we hypothesized that a combination of exercise and drug therapy would improve the treatment outcomes of MI. Therefore, in this study, we aimed to explore the effects of exercise training and ALPR on MI treatment. Our goal was to determine whether their combination could form a new treatment strategy and provide potential evidence for improving the treatment of elderly patients with MI. We present the following article in accordance with the ARRIVE reporting checklist (available at https://atm.amegroups.com/article/view/10.21037/atm-22-5763/rc).
Methods
Experimental animals
A protocol was prepared before the study without registration. Twenty-five male Wistar rats aged 20 months were purchased from Beijing Vital River Laboratory Animal Technology Co. Ltd. (Beijing, China). All rats were housed in a 12/12 h dark/light environment at a temperature of 22 ℃ and relative humidity of 50%. Follow-up experiments were conducted after 7 days of adaptive feeding. Our relevant experiments were completed with the assistance of the staff of Guangdong Experimental Animal Center. Animal experiments were performed under a project license (No. C202204-06) granted by Ethics Committee of Guangdong Experimental Animal Center, in compliance with Ethics Committee of Guangdong Experimental Animal Center guidelines for the care and use of animals.
Establishment and treatment of the rat model
The experimental MI model was induced by ligating the left anterior descending (LAD) coronary artery as previously described (18). After the rats were anesthetized with 3% pentobarbital sodium (30 mg/kg), an ALC-V8S small animal ventilator (Shanghai Alcott Biotechnology Co. Ltd., China) was connected for controlled mechanical-assisted ventilation. The rats’ extremities were connected to the BL-420E biological and functional experimental system (Chengdu Instrument Factory, China) with pinpoint electrodes for the electrocardiogram (ECG) monitoring and recording. After opening the chest, the pericardium was opened, and the LAD coronary artery was ligated with a 6/0 wire. The MI model was considered successfully constructed when there was a significant increase in the ST segment of the ECG (data not shown). The thorax was subsequently closed.
Twenty-five rats were randomly divided into the following five groups: Control group (n=5), MI group (n=5), exercise training group (Trained, n=5), ALPR treatment group (n=5), and exercise training combined with ALPR treatment group (Trained + ALPR, n=5). In the Control group, the LAD coronary artery of the rats was exposed, and the suture was threaded but not ligated. The exercise training was adapted from the study by Shen et al. (19). Before inducing the MI model, the rats were made to perform intermittent running on a motorized rodent treadmill five times a week, lasting 6 weeks. The 5-day incremental running treadmill test was performed with a graded running speed. The exercise intensity began at 10 m/min and gradually increased to 22 m/min. After the adaptive training period, the incremental running treadmill test was conducted with an initial speed setting of 10 m/min. Then, the speed was increased by 22 m/min every 3 min. The MI model was induced 2 days after completing the last exercise and supplementation. In the ALPR group, before inducing the MI model, the rats were injected with 8 µg/kg of ALPR (14) via the tail vein every day for 6 consecutive weeks before MI induction. In the Trained + ALPR group, before inducing the MI model, the rats were made to perform weekly aerobic training on a treadmill, and 8 µg/kg of ALPR was injected via the tail vein every day for 6 consecutive weeks. Then, 48 h after completing the last exercise and supplementation, the MI model was induced. One day after MI induction, the blood tissue samples of the rats in each group were collected; one week after the induction, echocardiography was performed. The rats were then sacrificed, and the rat heart tissues were collected and weighed.
Echocardiography
The occurrence of MI was determined by echocardiography 1 week after MI induction using a GE Vivid 7 Dimension ultrasound diagnostic instrument (General Electric Company, Boston, USA) with a 10S MHZ transducer at 3-cm depth. The rats were weighed and injected intraperitoneally with 3% pentobarbital sodium (30 mg/kg) according to the physical fitness standard. After anesthesia, the rats’ limbs were fixed on the operating table. The rats were laid on their left side, and their chest hair was shaved. Subsequently, a small amount of coupling agent was applied to measure the left ventricular end-diastolic diameter (LVEDD), left ventricular end-systolic diameter (LVESD), left ventricular ejection fraction (LVEF), and left ventricular fraction shortening (LVFS) rate of rats in each group.
Measurement of rat heart weight (HW)
The body weight (BW) and HW of the rats were first weighed, and then the tibia length (TL) was measured. After that, their heart mass index (HMI) was calculated as (HW/BW) ×100%, and their HW-to-TL ratio was calculated as (HW/TL) ×100%.
Triphenyl tetrazolium chloride (TTC) staining
The heart was removed, then the left and right atria were removed and frozen at −20 ℃ for 20 min. Five myocardial slices approximately 2-mm thick were transected vertically in their long axis and sequentially placed in 2% TTC buffer (Sigma-Aldrich, USA) and incubated at 37 ℃ for 30 min in the dark. After staining, 10% formaldehyde was used instead of TTC buffer. Sections were fixed for 4–6 h, and the infarct size was determined using ImageJ software. Normal myocardial tissue was red, while infarcted areas were grayish-white.
Masson’s trichrome stain
The heart tissues were fixed in 4% paraformaldehyde for 48 h, followed by dehydration and paraffin embedding. Then, the embedded samples were cut into 5-µm-thick sections. Masson staining was used to observe the distribution and degree of myocardial interstitial fibrosis and evaluate the degree of MI.
Hematoxylin and eosin (H&E) staining
The heart tissues of the rats in each group were fixed in neutral formaldehyde for 20 min. Then, a 15% ethylenediaminetetraacetic acid (EDTA) solution was applied for decalcification, and ethanol was used for dehydration in a stepwise manner. After routine paraffin embedding, the heart tissues were prepared in sagittal plane sections of approximately 4 µm thickness. Subsequently, the sections were stained with hematoxylin for 5 min at 25 ℃, differentiated with hydrochloric acid alcohol, and turned blue with 1% ammonia water. After rinsing with tap water, the sections were stained with eosin for 30 s, dehydrated with alcohol, and then treated with xylene. Afterward, the slides were mounted, and the heart tissues were observed under a biological microscope.
Immunohistochemistry
First, 5-µm-thick paraffin sections of heart tissue were deparaffinized, placed in 10 mM sodium citrate solution (pH 6.0), and heated at 100 ℃ for 10 min. Then, the sections were incubated with 3% hydrogen peroxide to block endogenous peroxidase activity. They were subsequently treated with 1 mM EDTA (pH 9.0) for 15 min and then with 5% bovine serum albumin for 1 h at room temperature. On completion of the incubation with primary antibody CD34 overnight at 4 ℃, 30 min of incubation with the secondary antibody was performed at room temperature. Next, treatment with diaminobenzidine or 3-amino-9-ethylcarbazole for 28 min was allowed for color development, followed by hematoxylin counterstaining and mounting using neutral resin. Lastly, the samples were observed and photographed under a microscope.
Detection of biochemical reagents
Whole blood was collected from the rat through the retro-orbital vein and placed in a 1.5 mL centrifuge tube. Then, the blood was centrifuged at 2,000 r/min for 20 min at 4 ℃, and the supernatant was collected in a new centrifuge tube to obtain the serum. A fully automatic biochemistry analyzer was used to detect the cardiac troponin I (cTnI), cardiac troponin T (cTnT), and creatine kinase MB isoenzyme (CK-MB) serum levels in the rats.
Detection of energy metabolism indicators
A total of 50 mg of the collected rat heart tissues in each group were placed in a homogenization tube, and 1 mL of pre-cooled PBS buffer was added. The tissues were placed in a low-temperature homogenizer for homogenization and breaking. Afterward, the homogenization tube was placed at a temperature of 4 ℃ and centrifuged at 12,000 g for 10 min to remove cell debris. Subsequently, the supernatant was transferred to a new centrifuge tube. Specifically, some supernatant was stored at −80 ℃, and the others were detected according to the instructions of the adenosine triphosphate (ATP), adenosine diphosphate (ADP), and adenosine monophosphate (AMP) test kits (Beyotime Biotechnology, China).
Western blot
Total proteins of the heart tissue were extracted using RIPA lysate (50 mM Tris, 150 mM NaCl, 1% NP-4, 0.5% sodium deoxycholate, 0.1% SDS, PH7.4) buffer, and the concentration of the extracted proteins was determined using a bicinchoninic acid (BCA) kit. A total of 20 µg of the protein with supplementary 1× loading buffer was boiled for denaturation. The proteins were then separated by sodium dodecyl sulfate-polyacrylamide gel electrophoresis (SDS-PAGE) and transferred to polyvinylidene fluoride (PVDF) membranes. The membranes were blocked with 5% non-fat dry milk for 1 h. Primary antibodies, including vascular endothelial growth factor (VEGF) (ab32152, Abcam, UK), fibroblast growth factor (FGF) (9740S, CST, USA), thrombospondin-1 (TSP-1) (37879, CST), phosphorylated-P38 (p-P38) (4970S, CST), extracellular signal-regulated kinase 1/2 (ERK1/2) (4695S, CST), p-ERK1/2 (4370S, CST), c-Jun-N-terminal kinase (JNK) (9252S, CST), p-JNK (9255S, CST), and glyceraldehyde 3-phosphate dehydrogenase (GAPDH) (5174S, CST) were added for incubation overnight at 4 ℃ on a shaker. Subsequently, the membranes were rinsed three times, and a secondary antibody was added for 1 h of incubation at 25 ℃. After washing the membranes three times, a chemiluminescence reagent was added to develop the proteins, and image collection was conducted with the gel imaging system. Image J software was used to analyze the gray level of the protein bands, and GAPDH was used as an internal control to calculate the relative protein expression.
Statistical analysis
All data were measured three times independently. The results were expressed as the mean ± standard deviation (SD). All statistical analyses were performed using SPSS 26.0 software (IBM Corp., Armonk, NY, USA). One-way analysis of variance (ANOVA) followed by Tukey’s method was used for multiple group comparisons. The differences between groups were analyzed by independent sample t-test analysis. P<0.05 was used as the criterion for statistical significance.
Results
Exercise training combined with ALPR improved cardiac function in rats with MI
We assessed the effects of exercise training combined with ALPR treatment on cardiac function in MI rats via serum biochemical indexes and echocardiography. The results showed that compared with the Control group, LVEDD and LVESD in the MI group were significantly increased, while LVEF and LVFS were significantly decreased, indicating the successful construction of the MI rat model. Additionally, compared with the MI, Trained, and ALPR groups, the Trained + ALPR group had significantly lower LVEDD and LVESD but significantly higher LVEF and LVFS. The cardiac function indicators in the Trained + ALPR group were also better than those in the Trained or ALPR groups (P<0.01; Figure 1A-1D). In addition, the Trained, ALPR, and Trained + ALPR groups exhibited higher HW/BW and HW/TL ratios than the MI group (P<0.05; Figure 1E,1F). Further, the detection of cardiac biochemical indicators showed that cTnI, cTnT, and CK-MB serum levels in the MI group were significantly higher than in the Control group. The cTnI, cTnT, and CK-MB serum levels in the Trained, ALPR, and Trained + ALPR groups were notably lower than that in the MI group (P<0.01; Figure 1G-1I). Moreover, compared with the Trained and ALPR groups, the Trained + ALPR group displayed significantly decreased cTnI, cTnT, and CK-MB levels. The above results indicated that exercise training combined with ALPR treatment significantly improved the cardiac function of MI rats, and the efficacy was better than exercise training or ALPR alone.
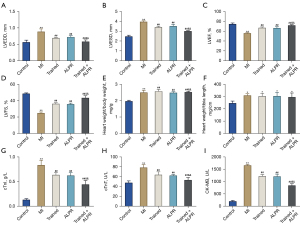
Exercise training combined with ALPR treatment reduced the degree of MI and myocardial injury in rats
We further explored the effects of exercise training combined with ALPR treatment on MI size and myocardial injury in MI rats. According to the results of TTC staining (P<0.01; Figure 2), no infarction was observed in the Control group, while different degrees of infarction were observed in the other four groups. Infarct size was significantly reduced in the Trained, ALPR, and Trained + ALPR groups compared to the MI group, with the smallest observed in the Trained + ALPR group. Similarly, Masson’s trichrome stain results showed no MI in the Control group, while the other four groups presented different degrees of MI. Compared with the MI group, the MI size was significantly reduced in the Trained, ALPR, and Trained + ALPR groups, with the smallest observed in the Trained + ALPR group (Figure 3A). H&E staining results showed that the Control group did not have inflammatory cell infiltration, and their cardiomyocytes were arranged in an orderly manner. In contrast, the MI group demonstrated increased inflammatory cell infiltration, and their myocardial fibers were disorderly arranged. Additionally, cardiomyocytes in the MI group were few and accompanied by necrosis. In the Trained and ALPR groups, inflammatory cell infiltration was decreased, and cardiomyocytes were more ordered than in the MI group, but slight edema appeared. In the Trained + ALPR group, cardiomyocyte inflammation was subtle, and the cardiomyocytes were arranged neatly. Compared with the Trained and ALPR groups, improvement in the Trained + ALPR group was most pronounced (Figure 3B). Thus, these findings suggested that exercise training combined with ALPR demonstrated promising potential in reducing MI size and myocardial injury in rats.
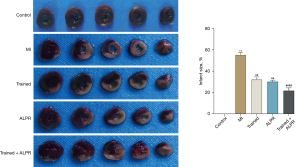
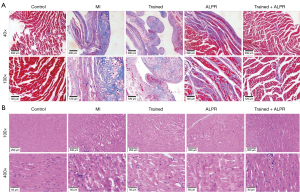
Exercise training combined with ALPR treatment promoted microangiogenesis in the myocardial tissue of rats with MI
In this section, we explored the effects of exercise training combined with ALPR treatment on microangiogenesis in the myocardial tissue of rat models. Immunohistochemistry results showed that, compared with the Control group, the expression of CD34 in the myocardial tissue of the MI group was significantly reduced. Compared with the MI group, the expression of CD34 was increased in the Trained, ALPR, and Trained + ALPR groups. Moreover, the expression of CD34 in the Trained + ALPR group was higher than in the Trained or ALPR groups. These findings showed that exercise training combined with ALPR treatment significantly increased the microvessel density of MI rats (Figure 4A). The expression of angiogenesis factors and FGFs was further determined. The results showed that VEGF and FGF expression in the myocardial tissue of the MI group was significantly lower than in the Control group, while TSP-1 expression was significantly increased. The above results indicated that angiogenesis in the myocardial tissue of the MI rats was inhibited. Compared with the MI group, VEGF and FGF expressions in the Trained, ALPR, and Trained + ALPR groups were increased, while TSP-1 expression was decreased. Furthermore, the Trained + ALPR group also showed an increase in VEGF and FGF expression and a decrease in TSP-1 expression compared with the Trained and ALPR groups (P<0.01; Figure 4B). These results suggested that exercise training combined with ALPR promoted microangiogenesis in the myocardial tissue of rats with MI.
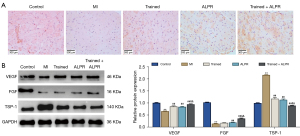
Exercise training combined with ALPR treatment restored the energy metabolism of cardiomyocytes in rats with MI
The effect of exercise training combined with ALPR treatment on the energy metabolism of cardiomyocytes was further investigated. The results showed that, compared with the Control group, the myocardial tissue of the rats in the MI group exhibited remarkably decreased ATP levels, notably increased ADP and AMP levels, and significantly reduced ATP/ADP and ATP/AMP ratios. In addition, compared with the MI group, the ATP level in the Trained, ALPR, and Trained + ALPR groups was significantly increased, while the ADP and AMP levels were significantly decreased. Compared with the Trained or ALPR groups, the Trained + ALPR group displayed a marked increase in ATP level, ratios of ATP/ADP and ATP/AMP, and a remarkable decrease in ADP and AMP levels (P<0.05; Figure 5A-5E). All in all, these observations suggested that exercise training combined with ALPR treatment effectively restored the energy metabolism of cardiomyocytes in MI rats.
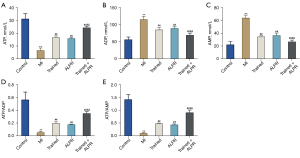
Exercise training combined with ALPR treatment inhibited the activation of the mitogen-activated protein kinase (MAPK) signaling pathway in the myocardial tissues of rats with MI
To explore the mechanism of exercise training combined with ALPR in treating MI rats, we detected the MAPK signaling pathway-related proteins using Western blotting. The results showed that compared with the Control group, the ratios of p-P38/P38, p-ERK1/2/ERK1/2, and p-JNK/JNK in the myocardial tissue of the MI group were notably increased. The Trained, ALPR, and Trained + ALPR groups presented significantly decreased p-P38/P38, p-ERK1/2/ERK1/2, and p-JNK/JNK ratios in the myocardial tissue compared with the MI group. Moreover, the ratios of p-P38/P38, p-ERK1/2/ERK1/2, and p-JNK/JNK in the Trained + ALPR group were significantly lower than those in the Trained and ALPR groups (P<0.05; Figure 6). Based on these findings, we can deduce that exercise training combined with ALPR treatment exerted its effects by regulating the MAPK/ERK signaling pathway.
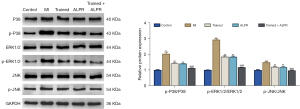
Discussion
In this study, we observed significant effects on MI rats by combining exercise training with ALPR treatment. Specifically, exercise training combined with ALPR treatment improved cardiac function in MI rats, relieved myocardial cell damage, and promoted microangiogenesis and energy metabolism. In addition, exercise training combined with ALPR treatment inhibited MAPK signaling pathway activation and alleviated MI and coronary microcirculation disorders in elderly rats. Moreover, the effects of exercise training combined with ALPR were better than exercise training or ALPR alone. The outcomes of this study suggested that exercise training combined with ALPR effectively treated MI.
Cardiac remodeling is a process of compensatory changes caused by MI, hypertension, and other heart diseases (20). Left ventricular (LV) remodeling is mainly manifested as changes in ventricular size and function (21). This study also revealed that the LVEDD and LVESD in the MI group were significantly higher than those of the Control group, and the LVEF and LVFS were significantly decreased. Moreover, the Trained + ALPR group demonstrated the most significant reduction in LVEDD and LVESD and increased LVEF and LVFS, suggesting that combination therapy could improve LV function after MI. In addition to the LVEDD and LVESD parameters, LV mass index is also an important indicator of cardiac function (22). This study also revealed that combination therapy significantly decreased LV mass index despite increased LV mass index after MI. The above results suggest that combination therapy improved MI injury by reducing LV hypertrophy.
According to previous studies, cardiomyocyte apoptosis is a major mechanism in the pathogenesis of several primary heart diseases (23,24). In addition, cardiomyocyte apoptosis after MI also plays a role in MI recurrence (25,26). In this study, we observed the MI area and found that the infarct area in the MI group was much larger than that in the control group. However, the infarct area in the Trained + ALPR group remained much lower than in the MI, Trained, or ALPR groups. As the most specific markers of cardiac injury, cTnI, cTnT, and CK-MB are often applied to diagnose subacute MI (27). The above markers are released from dead cells within 2–4 and 3–4 h after the onset of MI symptoms (28). In this study, the MI group showed a significant increase in cTnI, cTnT, and CK-MB levels; in contrast, these factors were significantly lower in the Trained + ALPR group. H&E staining also indicated that combination therapy significantly reduced cardiomyocyte injury in MI rats.
ATP is essential for normal cardiac function, including myofibril contraction, ion transport, and cardiomyocyte viability (29,30). Creatine phosphokinase is an enzyme found in many tissues, including cardiac muscle tissue, which helps regulate the level of ATP within the cells. A study reported that the concentrations of creatine phosphocreatine and ATP are significantly decreased in the heart of MI patients compared with healthy controls (31). Specifically, myocyte loss and/or reduced mitochondrial production of high-energy phosphoryl can cause a reduction in the content of CK that transfers into myofibrils in viable myocytes through CK flux, resulting in a decrease in ATP levels (32). In this study, the myocardial tissue of the rats in the MI group displayed a significant decrease in ATP levels compared with the Control group. Moreover, exercise training or ALPR not only significantly increased ATP levels in the myocardial tissue of the MI rats but also had the best efficacy. The exercise training combined with ALPR group showed the most significant efficacy. In addition, a study shows that aerobic rehabilitation exercise with appropriate intensity can improve the physiological and psychological state of cardiac function in patients with MI (33). Based on the above findings, it can be concluded that combination therapy effectively improved the energy metabolism of cardiomyocytes in MI rats.
According to an extensive body of previous research on the mechanisms associated with MI, activation of the MAPK signaling pathway can cause pathological myocardial hypertrophy and cardiac remodeling, leading to heart failure (34). MAPKs have three subfamilies: ERK1/2, JNK, and P38. MAPK signaling promotes nuclear factor-kappa B (NF-κB) to trigger additional inflammatory cytokines, thereby inducing further damage within myocardial tissues (35). The MAPK/ERK signaling pathway has been well studied and is known to be involved in regulating multiple biological processes, such as the survival, growth, and death of various cells and the immune response associated with inflammation (36). In this study, compared with the Control group, the ratios of p-P38/P38, p-ERK1/2/ERK1/2, and p-JNK/JNK in the myocardial tissue of the MI group were markedly increased; in contrast, the ratios of p-P38/P38, p-ERK1/2/ERK1/2, and p-JNK/JNK in the myocardial tissue of the Trained + ALPR group were notably reduced. Based on the above outcomes, exercise training combined with ALPR treatment exerted its effect by regulating the MAPK/ERK signaling pathway.
Conclusions
In summary, exercise training combined with ALPR treatment demonstrated significant positive effects on aged rats with MI. Additionally, exercise training combined with ALPR treatment showed that it could protect the heart by regulating the MAPK signaling pathway and demonstrated better efficacy than exercise training or ALPR treatment alone. Therefore, exercise training combined with ALPR may have great potential in the early treatment of MI, and the mechanism deserves further study.
Acknowledgments
Funding: This work was supported by the Cardiovascular Health Alliance Fund (No. 2017-CCA-VG-032) and the Guangzhou Science and Technology Planning Project (No. 202002020036).
Footnote
Reporting Checklist: The authors have completed the ARRIVE reporting checklist. Available at https://atm.amegroups.com/article/view/10.21037/atm-22-5763/rc
Data Sharing Statement: Available at https://atm.amegroups.com/article/view/10.21037/atm-22-5763/dss
Conflicts of Interest: All authors have completed the ICMJE uniform disclosure form (available at https://atm.amegroups.com/article/view/10.21037/atm-22-5763/coif). The authors have no conflicts of interest to declare.
Ethical Statement: The authors are accountable for all aspects of the work in ensuring that questions related to the accuracy or integrity of any part of the work are appropriately investigated and resolved. Animal experiments were performed under a project license (No. C202204-06) granted by Ethics Committee of Guangdong Experimental Animal Center, in compliance with Ethics Committee of Guangdong Experimental Animal Center guidelines for the care and use of animals.
Open Access Statement: This is an Open Access article distributed in accordance with the Creative Commons Attribution-NonCommercial-NoDerivs 4.0 International License (CC BY-NC-ND 4.0), which permits the non-commercial replication and distribution of the article with the strict proviso that no changes or edits are made and the original work is properly cited (including links to both the formal publication through the relevant DOI and the license). See: https://creativecommons.org/licenses/by-nc-nd/4.0/.
References
- Chen YQ, Quan S, Du FX, et al. Progress in chemiluminescence and electrochemiluminescence analysis of markers of acute myocardial infarction. Chinese Journal of Analytical Chemistry 2021;49:849-57.
- Lanza GA. The universal definition of myocardial infarction: some issues and concerns. Eur Heart J 2008;29:1209-author reply 1209-10. [Crossref] [PubMed]
- Lattuca B, Kerneis M, Zeitouni M, et al. Elderly Patients with ST-Segment Elevation Myocardial Infarction: A Patient-Centered Approach. Drugs Aging 2019;36:531-9. [Crossref] [PubMed]
- Sandoval Y, Jaffe AS. Type 2 Myocardial Infarction: JACC Review Topic of the Week. J Am Coll Cardiol 2019;73:1846-60. [Crossref] [PubMed]
- Sheifer SE, Manolio TA, Gersh BJ. Unrecognized myocardial infarction. Ann Intern Med 2001;135:801-11. [Crossref] [PubMed]
- Han F, Chen Q, Su J, et al. MicroRNA-124 regulates cardiomyocyte apoptosis and myocardial infarction through targeting Dhcr24. J Mol Cell Cardiol 2019;132:178-88. [Crossref] [PubMed]
- Saleh M, Ambrose JA. Understanding myocardial infarction. F1000Res 2018;7:eF1000 Faculty Rev-1378.
- Takata J, Nishinaga M, Doi Y. Reperfusion therapy for acute myocardial infarction in elderly patients. Nihon Ronen Igakkai Zasshi 2006;43:693-6. [Crossref] [PubMed]
- Zhan ZS, Qin SQ, Wang YL, et al. Correlation between serum levels of human cartilage glycoprotein 39 and soluble tumor necrosis factor receptor 1 and cardiac function and prognosis in patients with acute myocardial infarction. Chinese Journal of Arteriosclerosis 2022;3:243-7.
- Hanchanale V, Eardley I. Alprostadil for the treatment of impotence. Expert Opin Pharmacother 2014;15:421-8. [Crossref] [PubMed]
- Dean RC, Lue TF. Physiology of penile erection and pathophysiology of erectile dysfunction. Urol Clin North Am 2005;32:379-95. v. [Crossref] [PubMed]
- Tu JH, Xu Y, Dai Y, et al. Effect of alprostadil on myocardial fibrosis in rats with diabetes mellitus via TGF-β1/Smad signaling pathway. Eur Rev Med Pharmacol Sci 2019;23:9633-41. [Crossref] [PubMed]
- Zhang L, Zhang Y, Yu X, et al. Alprostadil attenuates myocardial ischemia/reperfusion injury by promoting antioxidant activity and eNOS activation in rats. Acta Cir Bras 2018;33:1067-77. [Crossref] [PubMed]
- Guo JD, Bian Y. Alprostadil injection in the treatment of 60 patients with acute cerebral infarction. Shaanxi Medical Journal 2012;41:563-4.
- Brehm M, Darrelmann E, Strauer BE. Stem cell therapy in acute myocardial infarction. Internist (Berl) 2008;49:1068-78. [Crossref] [PubMed]
- Lavie CJ, Sui X, Milani RV. Emotional distress after myocardial infarction: Importance of cardiorespiratory fitness. Eur J Prev Cardiol 2018;25:906-9. [Crossref] [PubMed]
- Bulluck H, Yellon DM, Hausenloy DJ. Reducing myocardial infarct size: challenges and future opportunities. Heart 2016;102:341-8. [Crossref] [PubMed]
- Wang D, Tian L, Lv H, et al. Chlorogenic acid prevents acute myocardial infarction in rats by reducing inflammatory damage and oxidative stress. Biomed Pharmacother 2020;132:110773. [Crossref] [PubMed]
- Shen M, Yu M, Li J, et al. Effects of exercise training on kinin receptors expression in rats with myocardial infarction. Arch Physiol Biochem 2017;123:206-11. [Crossref] [PubMed]
- Verbrugge FH, Dupont M, Rivero-Ayerza M, et al. Comorbidity significantly affects clinical outcome after cardiac resynchronization therapy regardless of ventricular remodeling. J Card Fail 2012;18:845-53. [Crossref] [PubMed]
- Ponce NE, Cano RC, Carrera-Silva EA, et al. Toll-like receptor-2 and interleukin-6 mediate cardiomyocyte protection from apoptosis during Trypanosoma cruzi murine infection. Med Microbiol Immunol 2012;201:145-55. [Crossref] [PubMed]
- Boyle AJ, Shih H, Hwang J, et al. Cardiomyopathy of aging in the mammalian heart is characterized by myocardial hypertrophy, fibrosis and a predisposition towards cardiomyocyte apoptosis and autophagy. Exp Gerontol 2011;46:549-59. [Crossref] [PubMed]
- Burke AP, Virmani R. Pathophysiology of acute myocardial infarction. Med Clin North Am 2007;91:553-72. ix. [Crossref] [PubMed]
- Frangogiannis NG. Pathophysiology of Myocardial Infarction. Compr Physiol 2015;5:1841-75. [Crossref] [PubMed]
- Kain V, Halade GV. Big eater macrophages dominate inflammation resolution following myocardial infarction. J Mol Cell Cardiol 2015;87:225-7. [Crossref] [PubMed]
- Rodríguez M, Lucchesi BR, Schaper J. Apoptosis in myocardial infarction. Ann Med 2002;34:470-9. [Crossref] [PubMed]
- Jaffe AS, Ordonez-Llanos J. High sensitivity troponin in chest pain and acute coronary syndromes. A step forward? Rev Esp Cardiol 2010;63:763-9. [Crossref] [PubMed]
- Burcu Bahadır E, Kemal Sezgintürk M. Applications of electrochemical immunosensors for early clinical diagnostics. Talanta 2015;132:162-74. [Crossref] [PubMed]
- Jennings RB, Reimer KA. Lethal myocardial ischemic injury. Am J Pathol 1981;102:241-55.
- Wallimann T. Bioenergetics. Dissecting the role of creatine kinase. Curr Biol 1994;4:42-6. [Crossref] [PubMed]
- Yabe T, Mitsunami K, Inubushi T, et al. Quantitative measurements of cardiac phosphorus metabolites in coronary artery disease by 31P magnetic resonance spectroscopy. Circulation 1995;92:15-23. [Crossref] [PubMed]
- Conway MA, Allis J, Ouwerkerk R, et al. Detection of low phosphocreatine to ATP ratio in failing hypertrophied human myocardium by 31P magnetic resonance spectroscopy. Lancet 1991;338:973-6. [Crossref] [PubMed]
- Cao MY, Yao ZH, Zhou X, et al. Effect of different intensity aerobic rehabilitation exercise on cardiac function and psychological state of patients with myocardial infarction. Chinese Journal of Gerontology 2015;1:95-7.
- Rossello X, Yellon DM. The RISK pathway and beyond. Basic Res Cardiol 2018;113:2. [Crossref] [PubMed]
- Verma VK, Malik S, Narayanan SP, et al. Role of MAPK/NF-κB pathway in cardioprotective effect of Morin in isoproterenol induced myocardial injury in rats. Mol Biol Rep 2019;46:1139-48. [Crossref] [PubMed]
- Ren GD. Research on cardioprotective effect of irbesartan in rats with myocardial ischemia-reperfusion injury through MAPK-ERK signaling pathway. Eur Rev Med Pharmacol Sci 2019;23:5487-94. [Crossref] [PubMed]
(English Language Editor: D. Fitzgerald)