To explore the active constituents of Sedum aizoon L in the treatment of coronary heart disease based on network pharmacology and molecular docking methodology
Highlight box
Key findings
• SL may be a potential therapeutic agent for CHD.
What is known and what is new?
• SL have been variously used to treating several diseases.
• SL role of CHD did not have reseatched. In presents study,it act as on targets such as STAT3, TP53, and VEGFA through tumor necrosis factor (TNF) signaling pathway, thereby playing a role in preventing and treating CHD.
What is the implication, and what should change now?
• SL may contribute to the treatment and prevention of CHD.
Introduction
Cardiovascular disease (CVD) is currently one of the leading causes of human mortality, and the residual risk of CVD patients remains high through lifestyle interventions and pharmacological treatment (1). Although mortality from coronary heart disease (CHD) has declined in recent years, CHD remains the leading cause of death worldwide and carries a significant economic burden (2,3). The search for drugs that can prevent and treat CHD is important to further reduce the incidence of major adverse cardiovascular events in patients with CHD. Currently, most drugs used in clinical practice are chemically synthesized and are prone to drug resistance and certain adverse events. In contrast, Chinese herbal medicines have achieved promising clinical efficacy by treating the disease from a multi-pathway and multi-target perspective (4).
Studies have shown that herbal medicines can prevent CHD by regulating oxidative stress and promoting angiogenesis to protect against myocardial ischemic injury and other pathways (5,6). Sedum aizoon L (SL), an herb of the Sedum family, contains a large number of active substances that are considered to have medicinal and food-related effects. Numerous reports have indicated that SL mainly exerts antibacterial and blood pressure-lowering activity (7-9). Recent study has shown that components of SL can effectively improve glucolipid metabolism, organ coefficients and antioxidant stress enzymes, reduce liver tissue damage, and regulate redox imbalance in type 1 diabetes mellitus (T1DM) mice by modulating the Nrf2/Keap1/ARE signaling pathway (10). However, there is a paucity of examining the role of SL in the treatment of CHD and this warrants further investigation.
Cyberpharmacology is an interdisciplinary field based on bioinformatics, molecular biology, and major databases to study the relationship between “drug-gene target and disease” (11,12). Cyberpharmacology can elucidate the mechanisms of action of multi-component and multi-target herbal medicines, while screening the main active ingredients. There is currently a lack information regarding the potential effects and mechanisms of SL in the treatment of CHD. Therefore, this study conducted an in-depth analysis of the effective active ingredients of SL in the treatment of CHD, and the related action targets and signaling pathways through a network pharmacology approach. This information will provide novel insights into potential clinical treatments for CHD. We present the following article in accordance with the STREGA reporting checklist (available at https://atm.amegroups.com/article/view/10.21037/atm-22-5391/rc).
Methods
Acquisition of active ingredients and targets of SL
The active ingredients and corresponding targets of SL were identified by searching with the keyword SL in the Systematic Pharmacology Database and Analysis Platform for Traditional Chinese Medicine (https://old.tcmsp-e.com/tcmsp.php; TCMSP), as well as the Uniprot database (https://www.uniprot.org/). i.e., the targets on which the active ingredients of King’s panax ginseng act. We used cytoscape to visualize and analyze the active ingredients of SL and their corresponding targets.
Acquisition of CHD targets
Using “coronary heart disease” as a keyword, the DisGeNet (https://www.disgenet.org/) and GENECARDs (https://www.genecards.org/) databases were searched to obtain datasets of CHD targets. The CHD dataset was retrieved using the Gene Expression Omnibus data base (GEO) database to obtain the GSE20680 and GSE20681 datasets, which were normalized using the “limma” package in R software. In the above datasets, P<0.05 was set as the criteria for differential genes, which were considered key genes for CHD. The “VennDiagram” package in R software was used to obtain the intersection as a potential target for improving CHD. There were at least two datasets intersecting as potential targets for improving CHD. Finally, the Venn diagram of the therapeutic targets of SL and the disease targets of CHD was drawn as the potential targets of SL for improving CHD. The study was conducted in accordance with the Declaration of Helsinki (as revised in 2013).
Functional enrichment analysis of potential action targets
Gene Ontology (GO) functional enrichment analysis and Kyoto Encyclopedia of Genes and Genomes (KEGG) pathway enrichment analysis were performed using the Cluster Profiler package in the R software to determine the potential targets of the Chinese medicine SL to improve CHD.
Protein-protein interaction (PPI) network construction
The intersection targets obtained above were uploaded to the STRING 11. 0 database (https://string-db.org/), the species was set as “Homo sapiens”, and the minimum interaction threshold was set to 0.4. The free nodes were removed to obtain the PPI network, and the cytohubba plug-in in Cytoscape software was used to obtain the top 10 key targets for improving CHD based on the MCC algorithm, from the highest to the lowest MCC score.
The main components of SL in the treatment of CHD
To determine the main active ingredients of SL in improving CHD, the active ingredients of SL and the potential targets of improving CHD were used to construct an intersection gene Venn diagram using the “VennDiagram” package in R software. The active ingredients with the potential to improve CHD were considered the main active ingredients of SL in improving CHD. Based on these intersecting genes, the Cluster Profiler package was used to construct the GO functional analysis and KEGG enrichment analysis to elucidate the potential mechanisms of action.
Molecular docking
The network pharmacology analysis identified 10 key genes that play important roles in the ability of SL to improve CHD. Using the RSCB PDB (http://www.rcsb.org/) database, we downloaded the optimal pdb format files of the above 10 key protein receptors, performed dehydration and hydrogenation operations with PyMOL. The Auto Dock software was used to merge non-polar hydrogen, determine the atom type and other characteristics, and the results were saved in pdbqt format. The coordinates and sizes of the binding sites of the key protein receptors were determined and saved in the relevant format as txt files. Finally, vina diagram was run separately for molecular docking to virtually screen the active ingredients of Chinese medicine SL for improving CHD. The docking results of each important protein receptor with the screened active ingredients of SL with lower binding energy and better conformation were selected and visualized using the PyMOL software.
Statistical analysis
Data were obtained from public database downloads. For the identification of differentially expressed genes (DEGs), |log2 (foldchange)| >1 and P value <0.05 were set as the statistically significant criteria. For GO and KEGG analyses, a q value <0.05 was considered statistically significant.
Results
Active components and targets of SL
According to the screening conditions, a total of 12 active ingredients and 134 therapeutic targets of SL were obtained from the TCMSP database, and the regulatory network of active ingredients and targets of SL was constructed using Cytoscape software. As shown in Figure 1A, the center circle is the active ingredient of SL, and the surrounding circles are the targets, the color shades and the size of the diagram are determined by the connectivity between the active ingredient and the targets. The results identified the top three active ingredients as ursolic acid, myricetin, and beta-sitosterol (Table 1).
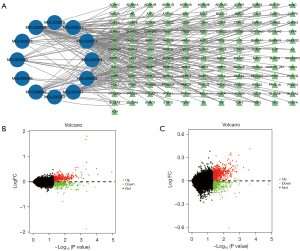
Table 1
MOL ID | Active ingredients |
---|---|
MOL002008 | Myricetin |
MOL002075 | 1-[(2S)-1-methyl-2-piperidyl]acetone |
MOL000263 | Oleanolic acid |
MOL002930 | Tyrosol |
MOL000357 | Sitogluside |
MOL000358 | Beta-sitosterol |
MOL000359 | Sitosterol |
MOL000511 | Ursolic acid |
MOL000513 | 3,4,5-trihydroxybenzoic acid |
MOL000579 | Hydroquinone |
MOL006086 | Isomyricitrin |
MOL006089 | (1R)-2-[(2S)-1-methyl-2-piperidyl]-1-phenyl-ethanol |
MOL, molecular.
CHD target screening
A total of 221 DEGs were identified in the GSE20680 dataset, of which, 150 were upregulated and 71 were downregulated. In the GSE2681 dataset, a total of 297 DEGs were identified, including, 212 upregulated genes and 85 downregulated genes (Figure 1B,1C).
Potential therapeutic targets of SL
Based on the DEGs screened in the GEO dataset and the CHD-related genes identified in the DisGeNet and GENECARDs databases, the targets that intersected at least 2 or 3 times were identified and defined as CHD-related therapeutic targets. Finally, a total of 52 potential targets were identified for SL in the treatment of CHD (Figure 2A,2B).
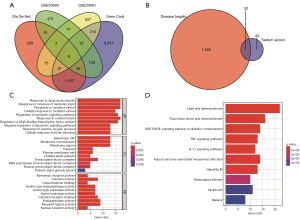
GO and KEGG analyses, and the PPI network
To further explore the mechanisms of action of SL in the treatment of CHD, we performed enrichment analysis and PPI network construction on the above screened targets. The genes that intersected in the two databases were examined. The targets were mainly enriched in the biological processes (BP) including response to lipopolysaccharide, response to molecule of bacterial origin, and response to oxidative stress; cell components (CCs) including membrane raft, membrane microdomain, and membrane region; and molecular functions (MFs) including endopeptidase activity, RNA polymerase II transcription factor binding, and activating transcription factor binding. KEGG enrichment analysis showed that the targets were mainly enriched in lipid and atherosclerosis, AGE-RAGE signaling pathway in diabetic complications, TNF signaling pathway, and IL-17 signaling pathway (Figure 2C,2D). The PPI network was then constructed for potential therapeutic targets, and the results showed that STAT3, TP53, VEGFA, intercellular adhesion molecule 1 (ICAM1), interleukin 1B (IL1B), interleukin 6 (IL-6), matrix metallopeptidase 2 (MMP2), matrix metallopeptidase 9 (MMP9), TNF, and prostaglandin-endoperoxide synthase 2 (PTGS2) were the top 10 core genes (Figure 3).
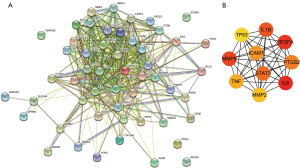
Identification of core active ingredients and their mechanisms of action
The identified active ingredients of SL were intersected with the disease targets of CHD. A total of 28 target genes were identified, and ursolic acid was determined to be the main core ingredient of SL. Further enrichment analysis showed a high degree of overlap with GO and KEGG of SL target genes (Figure 4).
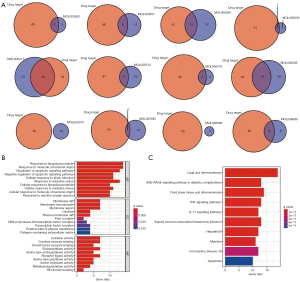
Molecular docking
The top 10 identified targets, including TP53, TNF, and IL-6, were identified by PPI network analysis, and the corresponding active ingredients were identified by molecular docking (Figure 5).
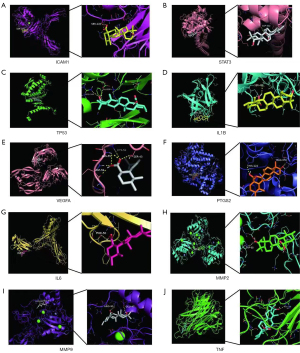
Discussion
Medications are one of the main treatment modalities for CHD, but the need for patients to take multiple medications at the same time due to underlying comorbidities is a great burden for both patients and society. Despite the multiple drugs available for the treatment of CHD, they have limited mechanisms of action and long-term use can lead to complications. Network pharmacology is of great significance and high research value in revealing the treatment mechanism and pharmacology laws of complex traditional Chinese medicine. The practical application of network pharmacology also proves that the combination of traditional Chinese medicine and modern medical technology is a powerful way and can play an important role in the field of traditional Chinese medicine. This current study used network pharmacology to identify the potential targets and active ingredients of SL in the treatment of CHD, and preliminarily elucidated the possible mechanisms of action.
The PPI core network and molecular docking analysis revealed that the major components of SL may be involved in the regulation of CHD through genes such as STAT3, TP53, VEGFA, IL-6, MMP2, MMP9, and PTGS2. CHD is a chronic, progressive, and inflammatory lesion of the coronary arteries. STAT3 and TP53, as transcription factors of the classical cellular pathway, may play a key role in the inflammatory and immune response (13,14). Study has shown that the IL-6/JAK/STAT3 signaling pathway plays an vital role in endothelial cell dysfunction and immune cell differentiation in atherosclerosis (15). VEGFA has been demonstrated to be associated with pathological angiogenesis, and its genetic polymorphisms may have a significant impact on susceptibility to CHD in the Han Chinese population (16). The VEGF family has shown extraordinary potential in the treatment of CHD by regulating angiogenesis, inflammation, and apoptosis (17). IL-6 is independently associated with the risk of major adverse cardiovascular events, as well as cardiovascular and all-cause mortality in patients with stable CHD, possibly reflecting the pathophysiological involvement of IL-6 in the development of these events (18). MMP2 and MMP9 play important roles in extracellular matrix and collagen metabolism, and studies have shown that atheromatous plaques in patients with acute coronary syndrome have a large number of macrophages and smooth muscle cells with a significant increase in MMP2/MMP9 expression, suggesting a significant role of MMP2/MMP9 in the pathogenesis of atherosclerosis (19-21). PTGS2 is a key enzyme in the synthesis of prostaglandins and has a critical role in the inflammatory response, and previous reports have suggested that PTGS2 may be a potential therapeutic target in CHD (22,23).
KEGG enrichment analysis revealed that SL treatment of CHD was mainly enriched in signaling pathways such as AGE-RAGE signaling pathway in diabetic complications, TNF signaling pathway, and IL-17 signaling pathway. AGE/RAGE signaling pathway in diabetic complications can regulate the production and expression of TNF-α in type 2 diabetes and promote endothelial dysfunction, which leads to cardiovascular disease (24). VEGF enhances vascular endothelial cell proliferation, migration, and survival, and is an important mediator of angiogenesis (25). TNF-α promotes VEGF expression and angiogenesis, mediates endothelial chemerin expression under hypoxic conditions, and promotes early angiogenesis (26,27). IL-17 acts mainly on vascular and cardiac myocytes and exacerbates inflammation, coagulation, and thrombosis, in addition to activating downstream pathways such as NF-KB and promoting the secretion of related cytokines (28,29). In addition, it was found that hyperglycemia may exacerbate the complexity of coronary atherosclerosis through IL-17/IL-10 signaling (30). It is thus hypothesized that the active ingredients of SL may act on the core targets of the above-mentioned signaling pathways to treat CHD.
The results of this study showed that ursolic acid, myricetin, and beta-sitosterol are the main active ingredients of SL for the treatment of CHD. Ursolic acid belongs to a group of pentacyclic triterpenoids with C-3 hydroxyl and C-28 carboxylic acid groups and is widely found in the wax layer of coffee, lavender, and fruits (31,32). Studies have shown that ursolic acid can be used as an alternative drug in the treatment of diseases such as swelling, diabetes, and liver disease (33). In addition, it has been reported that the use of ursolic acid reduces oxidative effects in rats with diabetic cardiomyopathy, decreases the expression of TNF-α, MCP-1, and TGF-β1 in the heart, and lowers blood glucose levels, in addition to improving the function of the heart (34,35). Myricetin is a natural flavonoid that inhibits the proliferation and migration of vascular smooth muscle cells through inhibition of the TGFBR1 signaling pathway, thereby inhibiting neoplastic endothelial proliferation (36). Myricetin can reduce myocardial injury and heat stroke mortality through upregulation of heat shock protein (HSP)-72, which is expected to be a novel therapeutic agent for heat stroke prevention (37). In addition, myricetin has a protective effect on myocardial injury caused by acute ischemia-reperfusion in isolated rat hearts (38). Taken together, these findings are consistent with our findings that myricetin has cardioprotective effects. Beta-sitosterol is a polyphenolic hydroxamic acid compound widely found in plants and also one of the main components of eukaryotic cell membranes. It has a high concentration in plant oils, seeds, and fruits and vegetables, with antioxidant, cholesterol-lowering, anti-inflammatory, and anti-diabetic effects (39). It has been shown that beta-sitosterol-mediated silver nanoparticles appear cytotoxic in human colon cancer and promote apoptosis in colon cancer cells (40). The advantage of network pharmacology is that it can effectively predict the target of drug and disease interaction, but the difficulty is that molecular docking requires corresponding computer simulation technology. The lack of basic experimental verification is our limitation.
Conclusions
In summary, SL may act on the above targets and pathways to achieve hypoglycemic and anti-inflammatory effects to prevent and treat CHD. However, since the information in the online database does not completely cover all potential targets, this may have limited the study somewhat. Further cellular and animal experiments are warranted to verify the results herein, and to provide further support and theoretical basis for the clinical use of SL in the treatment of CHD.
Acknowledgments
Funding: This research was supported by Baise Scientific Research and Technology Development Program “City-School Science and Technology Cooperation” Project (Baise Science and Technology No. 20170813).
Footnote
Reporting Checklist: The authors have completed the STREGA reporting checklist. Available at https://atm.amegroups.com/article/view/10.21037/atm-22-5391/rc
Conflicts of Interest: All authors have completed the ICMJE uniform disclosure form (available at https://atm.amegroups.com/article/view/10.21037/atm-22-5391/coif). The authors have no conflicts of interest to declare.
Ethical Statement: The authors are accountable for all aspects of the work in ensuring that questions related to the accuracy or integrity of any part of the work are appropriately investigated and resolved. The study was conducted in accordance with the Declaration of Helsinki (as revised in 2013).
Open Access Statement: This is an Open Access article distributed in accordance with the Creative Commons Attribution-NonCommercial-NoDerivs 4.0 International License (CC BY-NC-ND 4.0), which permits the non-commercial replication and distribution of the article with the strict proviso that no changes or edits are made and the original work is properly cited (including links to both the formal publication through the relevant DOI and the license). See: https://creativecommons.org/licenses/by-nc-nd/4.0/.
References
- GBD 2016 Causes of Death Collaborators. Global, regional, and national age-sex specific mortality for 264 causes of death, 1980-2016: a systematic analysis for the Global Burden of Disease Study 2016. Lancet 2017;390:1151-210. [Crossref] [PubMed]
- Kuznetsova T. Sex Differences in Epidemiology of Cardiac and Vascular Disease. Adv Exp Med Biol 2018;1065:61-70. [Crossref] [PubMed]
- Duggan JP, Peters AS, Trachiotis GD, et al. Epidemiology of Coronary Artery Disease. Surg Clin North Am 2022;102:499-516. [Crossref] [PubMed]
- Vavlukis M, Kedev S. Effects of High Intensity Statin Therapy in the Treatment of Diabetic Dyslipidemia in Patients with Coronary Artery Disease. Curr Pharm Des 2018;24:427-41. [Crossref] [PubMed]
- Yang X, He T, Han S, et al. The Role of Traditional Chinese Medicine in the Regulation of Oxidative Stress in Treating Coronary Heart Disease. Oxid Med Cell Longev 2019;2019:3231424. [Crossref] [PubMed]
- Zhai S, Zhang XF, Lu F, et al. Chinese medicine GeGen-DanShen extract protects from myocardial ischemic injury through promoting angiogenesis via up-regulation of VEGF/VEGFR2 signaling pathway. J Ethnopharmacol 2021;267:113475. [Crossref] [PubMed]
- Li M, Qi Z, Hao Y, et al. New Adducts of Iriflophene and Flavonoids Isolated from Sedum aizoon L. with Potential Antitumor Activity. Molecules 2017;22:1859. [Crossref] [PubMed]
- Wang J, Chi Z, Zhao K, et al. A transcriptome analysis of the antibacterial mechanism of flavonoids from Sedum aizoon L. against Shewanella putrefaciens. World J Microbiol Biotechnol 2020;36:94. [Crossref] [PubMed]
- Xu T, Wang Z, Lei T, et al. New flavonoid glycosides from Sedum aizoon L. Fitoterapia 2015;101:125-32. [Crossref] [PubMed]
- Qi X, Lu XT, Sun XH, et al. The regulatory effect of total flavonoids of Sedum aizoon L. on oxidative stress in type 1 diabetic mice. Curr Res Food Sci 2022;5:1140-7. [Crossref] [PubMed]
- Wu Z, Li W, Liu G, et al. Network-Based Methods for Prediction of Drug-Target Interactions. Front Pharmacol 2018;9:1134. [Crossref] [PubMed]
- Zhou S, Ai Z, Li W, et al. Deciphering the Pharmacological Mechanisms of Taohe-Chengqi Decoction Extract Against Renal Fibrosis Through Integrating Network Pharmacology and Experimental Validation In Vitro and In Vivo. Front Pharmacol 2020;11:425. [Crossref] [PubMed]
- Qin H, Yeh WI, De Sarno P, et al. Signal transducer and activator of transcription-3/suppressor of cytokine signaling-3 (STAT3/SOCS3) axis in myeloid cells regulates neuroinflammation. Proc Natl Acad Sci U S A 2012;109:5004-9. [Crossref] [PubMed]
- Li D, Liu L, Yang S, et al. Exploring the Therapeutic Mechanisms of Huzhang-Shanzha Herb Pair against Coronary Heart Disease by Network Pharmacology and Molecular Docking. Evid Based Complement Alternat Med 2021;2021:5569666. [Crossref] [PubMed]
- Wiejak J, Dunlop J, Mackay SP, et al. Flavanoids induce expression of the suppressor of cytokine signalling 3 (SOCS3) gene and suppress IL-6-activated signal transducer and activator of transcription 3 (STAT3) activation in vascular endothelial cells. Biochem J 2013;454:283-93. [Crossref] [PubMed]
- Liu D, Song J, Ji X, et al. Association of Genetic Polymorphisms on VEGFA and VEGFR2 With Risk of Coronary Heart Disease. Medicine (Baltimore) 2016;95:e3413. [Crossref] [PubMed]
- Zhou Y, Zhu X, Cui H, et al. The Role of the VEGF Family in Coronary Heart Disease. Front Cardiovasc Med 2021;8:738325. [Crossref] [PubMed]
- Held C, White HD, Stewart RAH, et al. Inflammatory Biomarkers Interleukin-6 and C-Reactive Protein and Outcomes in Stable Coronary Heart Disease: Experiences From the STABILITY (Stabilization of Atherosclerotic Plaque by Initiation of Darapladib Therapy) Trial. J Am Heart Assoc 2017;6:005077. [Crossref] [PubMed]
- Manginas A, Bei E, Chaidaroglou A, et al. Peripheral levels of matrix metalloproteinase-9, interleukin-6, and C-reactive protein are elevated in patients with acute coronary syndromes: correlations with serum troponin I. Clin Cardiol 2005;28:182-6. [Crossref] [PubMed]
- Newby AC. Matrix metalloproteinase inhibition therapy for vascular diseases. Vascul Pharmacol 2012;56:232-44. [Crossref] [PubMed]
- Zaldivia MTK, McFadyen JD, Lim B, et al. Platelet-Derived Microvesicles in Cardiovascular Diseases. Front Cardiovasc Med 2017;4:74. [Crossref] [PubMed]
- Huo M, Wang Z, Wu D, et al. Using Coexpression Protein Interaction Network Analysis to Identify Mechanisms of Danshensu Affecting Patients with Coronary Heart Disease. Int J Mol Sci 2017;18:1298. [Crossref] [PubMed]
- Ruan Z, Wang S, Yu W, et al. LncRNA MALAT1 aggravates inflammation response through regulating PTGS2 by targeting miR-26b in myocardial ischemia-reperfusion injury. Int J Cardiol 2019;288:122. [Crossref] [PubMed]
- Gao X, Zhang H, Schmidt AM, et al. AGE/RAGE produces endothelial dysfunction in coronary arterioles in type 2 diabetic mice. Am J Physiol Heart Circ Physiol 2008;295:H491-8. [Crossref] [PubMed]
- Apte RS, Chen DS, Ferrara N. VEGF in Signaling and Disease: Beyond Discovery and Development. Cell 2019;176:1248-64. [Crossref] [PubMed]
- An L, Shen S, Wang L, et al. TNF-alpha increases angiogenic potential in a co-culture system of dental pulp cells and endothelial cells. Braz Oral Res 2019;33:e059. [Crossref] [PubMed]
- Chigusa S, Moroi T, Shoji Y. State-of-the-Art Calculation of the Decay Rate of Electroweak Vacuum in the Standard Model. Phys Rev Lett 2017;119:211801. [Crossref] [PubMed]
- Iwakura Y, Ishigame H, Saijo S, et al. Functional specialization of interleukin-17 family members. Immunity 2011;34:149-62. [Crossref] [PubMed]
- Robert M, Miossec P. Effects of Interleukin 17 on the cardiovascular system. Autoimmun Rev 2017;16:984-91. [Crossref] [PubMed]
- Li Q, Liu Y, Xia X, et al. Activation of macrophage TBK1-HIF-1α-mediated IL-17/IL-10 signaling by hyperglycemia aggravates the complexity of coronary atherosclerosis: An in vivo and in vitro study. FASEB J 2021;35:e21609. [Crossref] [PubMed]
- Kashyap D, Tuli HS, Sharma AK. Ursolic acid (UA): A metabolite with promising therapeutic potential. Life Sci 2016;146:201-13. [Crossref] [PubMed]
- Son J, Lee SY. Therapeutic Potential of Ursonic Acid: Comparison with Ursolic Acid. Biomolecules 2020;10:1505. [Crossref] [PubMed]
- Seo DY, Lee SR, Heo JW, et al. Ursolic acid in health and disease. Korean J Physiol Pharmacol 2018;22:235-48. [Crossref] [PubMed]
- Ullevig SL, Zhao Q, Zamora D, et al. Ursolic acid protects diabetic mice against monocyte dysfunction and accelerated atherosclerosis. Atherosclerosis 2011;219:409-16. [Crossref] [PubMed]
- Wang XT, Gong Y, Zhou B, et al. Ursolic acid ameliorates oxidative stress, inflammation and fibrosis in diabetic cardiomyopathy rats. Biomed Pharmacother 2018;97:1461-7. [Crossref] [PubMed]
- Chen G, Xu H, Wu Y, et al. Myricetin suppresses the proliferation and migration of vascular smooth muscle cells and inhibits neointimal hyperplasia via suppressing TGFBR1 signaling pathways. Phytomedicine 2021;92:153719. [Crossref] [PubMed]
- Lin X, Lin CH, Liu R, et al. Myricetin against myocardial injury in rat heat stroke model. Biomed Pharmacother 2020;127:110194. [Crossref] [PubMed]
- Qiu Y, Cong N, Liang M, et al. Systems Pharmacology Dissection of the Protective Effect of Myricetin Against Acute Ischemia/Reperfusion-Induced Myocardial Injury in Isolated Rat Heart. Cardiovasc Toxicol 2017;17:277-86. [Crossref] [PubMed]
- Babu S, Jayaraman S. An update on β-sitosterol: A potential herbal nutraceutical for diabetic management. Biomed Pharmacother 2020;131:110702. [Crossref] [PubMed]
- Shathviha PC, Ezhilarasan D, Rajeshkumar S, et al. β-sitosterol Mediated Silver Nanoparticles Induce Cytotoxicity in Human Colon Cancer HT-29 Cells. Avicenna J Med Biotechnol 2021;13:42-6. [Crossref] [PubMed]