Analysis of gut microbiota in patients with acute myocardial infarction by 16S rRNA sequencing
Highlight box
Key findings
• The results showed that a distinct difference of gut microbiota was observed in AMI patients compared to healthy controls.
• PCI treatment and routine drugs have no effect on gut microbiota in patients with AMI.
What is known and what is new?
• The disorder of gut microbiota structure was shown in cardiovascular patients.
• The expression of TMAO, a derivative of microbial metabolite, increased in peripheral blood of patients with AMI.
What is the implication, and what should change now?
• Attention should be paid to the treatment of gut microbiota in patients with AMI.
Introduction
Acute myocardial infarction (AMI) is caused by myocardial necrosis due to an acute or persistent reduction in the coronary artery blood supply and an insufficient oxygen supply (1). AMI is often accompanied by malignant arrhythmia, cardiogenic shock, and even sudden death (2). With the aging population, stresses of modern life, changes in eating habits, and influence of social and psychological factors, cardiovascular disease-related deaths, such as coronary heart disease and AMI, have become the primary cause of human mortality and pose a serious threat to human health. Percutaneous coronary intervention (PCI) can help to quickly open the responsible blood vessels, mitigate ischemia, and save lives; therefore, early PCI is invaluable for improving the overall survival rate of AMI patients.
In recent years, the close relationships between the microbiome and human diseases have been demonstrated, and studies have confirmed that gut microbiota are associated with extraintestinal diseases, such as liver-, brain-, and immunity-related conditions (3-5). The digestive tract is directly connected to the outside environment and contains up to 100 trillion microorganisms, mainly in the colon (6). The number of genes associated with gut microbes is thought to exceed that of the host by approximately 150-fold (7-9). Trimethylamine (TMA) and short-chain fatty acids (SCFA) are both produced by gut microbiota and can be absorbed into the blood through the intestinal mucosa. TMA is then converted into trimethylamine N-oxide (TMAO) in the liver (10,11). SCFA, including acetate, propionate, and butyrate, are absorbed by intestinal epithelial cells, providing energy for the intestinal epithelium and entering the blood stream to participate in distal metabolic functions. Kimura et al. found that SCFAs and ketosomes directly regulate sympathetic nervous system (SNS) activity at the sympathetic ganglion level via the G protein-coupled receptor 41 (GPR41) (12). These derived products may have profound roles in host metabolism, immunity, and nutrition and can produce either beneficial or harmful metabolites in the human body as a result of changes in the environment and diet (13). The gut microbiota and gut metabolites have associated with hypertension, dyslipidemia, systemic inflammation, which are risk factors of AMI. Human study showed that hypertensive patients have lower alpha diversity of gut microbiota and higher LPS-producing microbiota (14). Animal study point a direct role for SCFA in blood pressure regulation (15).
Recently, the emerging study of gut microbiota with acute myocardial infarction have shown that gut microbiota has alteration in patients (16,17), but there are few studies about the changes of gut microbiota post-treatment. In this study, we have collected three group fecal sample from health group, AMI patients’ group and post-treatment group. And we analyzed the community of microbiota of AMI patients and compared the effect of post-PCI treatment on gut microbiota for AMI patients. We present the following article in accordance with the MDAR reporting checklist (available at https://atm.amegroups.com/article/view/10.21037/atm-22-5671/rc).
Methods
Patients and healthy controls
A total of 20 AMI patients and 20 healthy people aged 45–85 years were consecutively recruited from the Department of Cardiology, Yijishan Hospital of Wannan Medical College between December 2018 and May 2019. The AMI patients in this study were diagnosed with ST-segment elevation myocardial infarction according to electrocardiographic (ECG) examination and laboratory examination of myocardial enzymes. The eligible volunteers were selected according to the following conditions: (I) age 45–85 years old; (II) no digestive system-related diseases in the past 3 years; (III) no history of surgery; (IV) no history of smoking, alcoholism, and various metabolic diseases; (V) all participants were volunteers and signed their informed consent voluntarily. Hypertension as an independent factor should be controlled below 135/90 mmHg. All of the healthy controls were from the patients’ families or close relatives with similar age and no other diseases.
Collection and detection of fecal samples
We collected a total of 40 stool samples from the healthy control group and AMI patient group. The study was conducted in accordance with the Declaration of Helsinki (as revised in 2013). The study was approved by ethics board of Wannan Medical College (No. 2020371) and informed consent was taken from all the patients. In AMI patients, a week after PCI, stool was collected again as the post-treatment group (AMI-T). All fresh fecal samples from all participants were collected in container with liquid protectant from Hangzhou Guhe Information Technology Co. Ltd. (Hangzhou, China) and stored at 4 ℃ within 2 h of collection. Subsequently, samples were returned to the company for testing. The primers for polymerase chain reaction (PCR) amplification of the V4 region of the bacterial 16S ribosomal RNA (16SrRNA) gene were the forward primer 515F (5'-GTGCCAGCMGCCGCGGTAA-3') and the reverse primer 806R (5'-GGACTACHVGGTWTCTAAT-3'). Sequencing was performed on the Illumina HiSeq4000 pair-end 2×150 bp platform (Illumina, San Diego, CA, USA) after quantification.
TMAO and SCFA measurement
Blood samples were collected from all participants, including the control group, within 12 hours after admission to the hospital before clinical treatment or after a week of clinical treatment. Within 60 min of collection, the plasma from the blood supernatant were separated after centrifugation at 3,000 rpm at 4 ℃ and stored at −80 ℃. TMAO and SCFA were detected by liquid chromatography/stable-isotope dilution/multiple-reaction monitoring/mass spectrometry (LC/MS/MS) (18) by Laboratory of School of Pharmacy, Wannan Medical College.
Date analysis
The data for each sample were separated from the original data, according to the barcode and primer sequences. After removing the barcode and primer sequences, the reads of each sample were spliced with a data analysis software (Vsearch v2.4.4) to get the original Tags data (Raw Tags). Concurrently, the quality of the sequence was controlled and filtered. The criteria for screening low quality sequences were as follows: the sequence was less than 150 bp, the average mass value was less than 20, the sequence contained unclear bases, and the single nucleotide repeat sequence contained >8 bp. The chimera sequence was removed and the final effective data were obtained. Operational taxonomic units (OTUs) analysis was performed using Vsearch v2.4.4, including de-repeating sequences, clustering, and de-chimerism (Rognes 2016). The differences in the UniFrac distance between the drawing box graph were compared using the t-test and the Monte Carlo test.
Statistical analysis
Results were expressed as mean ± SEM and differences between groups were determined by Student’s t-test for continuous variables or χ2 test for categorical variables using Graphpad Prism version 8.0 (Graphpad Software, La, Jolla, CA). A value of P<0.05 was considered statistically significant.
Results
Participants’ baseline characteristics
The baseline characteristics of patients with AMI and healthy controls were investigated in detail within 24 h of admission and are shown in Table 1. To select a suitable healthy control group, patients’ family members or healthy people living close to patients were recruited to the trial. The ranges and proportions of age, body mass index (BMI), sex, and diabetes mellitus in the AMI group were not significantly different from those in the control group. However, the rate of hypertension was significantly higher in the patient group than the control group.
Table 1
Variables | Healthy control (n=20) | AMI patients (n=20) | P value |
---|---|---|---|
Ages (years) | 63.6±16.2 | 65.8±14.1 | 0.65 |
Sex (female) | 11 | 8 | – |
BMI (kg/m2) | 21.67±2.4 | 22.81±2.5 | 0.15 |
Hypertension** | 2 | 12 | <0.01 |
History of smoking | 0 | 2 | – |
Dyslipidemia | 3.8±0.59 | 4.0±0.85 | 0.27 |
AST (U/L)** | 21.1±7.26 | 143.5±135.34 | <0.01 |
ALT (U/L)** | 18.55±10.84 | 30.4±17.85 | <0.01 |
BUN (mg/dL)** | 3.88±1.79 | 6.6±2.81 | <0.01 |
HDL-C (mg/dL) | 1.5±0.93 | 1.3±0.32 | 0.29 |
LDL-C (mg/dL) | 1.9±0.80 | 2.3±0.84 | 0.16 |
TG (mg/dL) | 1.48±0.31 | 1.4±0.51 | 0.59 |
Data are presented as mean ± SEM or number (n=20). **P<0.01, AMI vs. healthy control group. AMI, acute myocardial infarction; BMI, body mass index; AST, aspartate aminotransaminase; ALT, alanine transaminase; BUN, blood urea nitrogen; HDL-C, high-density lipoprotein cholesterol; LDL-C, low-density lipoprotein cholesterol; TG, triglycerides; SEM, standard error of the mean.
Characteristics of fecal microbiota in AMI patients
To analyze the changes in gut microbiota in patients with AMI, we collected a total of 123,906.2±9,989.6 tags from control, 123,915.3±16,551.6 tags from AMI, and 125,706.7±14,864.7 tags from AMI-T groups by 16s rRNA sequencing, and we screened 1,554±155.2, 2,244±163.7, 2,241±154.6 OTUs, respectively (Table 2). According to the OTU clustering results, a Venn diagram was drawn for OTUs at 97% similarity by default, and the number of OTUs common to the different groups were compared. The types of OTUs shared between the different groups are shown in the Venn diagram (Figure 1A). There was no significant change in the alpha diversity of the flora in the AMI group, as shown by Shannon and Simpson indexes, but the richness of the flora significantly decreased, as shown by Chao1 and ACE (Figure 1B-1E, Table 3). Principal component analysis (PCA), based on weighted UniFrac distance of the overall community structure, did not show any separation (Figure 2A). In terms of the total number, Figure 2B illustrates the differences between gut microbiota in the healthy control and AMI groups at the species level. In addition, no significant difference was found between the phylum level and the genus level (Figure 2C,2D). At the phylum level, Bacteroidetes’s proportion (32.20%±5.9%) was slightly elevated in AMI patients, whereas Firmicute’s (50.27%±16.8%) and proteobacteria (13.02%±6.3%) had no clear change (Figure 3A-3D). At genus level, the proportion of Bacteroides and Faecalibacterium had no change (Figure 3E,3F). Streptococcus only accounted for 0.34%±0.05% of the fecal microbiota in the healthy group; the proportion increased 10 times in the AMI patient group, and there was no decrease after treatment (Figure 3G). On the contrary, Lachnospira showed a downward trend, from 5.5% to 1.4% (Figure 3H).
Table 2
Groups | Fecal samples number | Tags (number of reads) | Clean-tags (number of reads) | OTUs |
---|---|---|---|---|
Control | 20 | 123,906.2±9,989.6 | 115,192.5±12,038.0 | 1,554±155.2 |
AMI | 20 | 123,915.3±16,551.6 | 113,519.1±17,839.0 | 2,244±163.7 |
AMI-T | 20 | 125,706.7±14,864.7 | 116,514.4±16,996.9 | 2,241±154.6 |
The data were presented as mean ± standard deviation. Control, healthy control group; AMI-T, AMI patients treatment group. AMI, acute myocardial infarction; OTUs, operational taxonomic units.
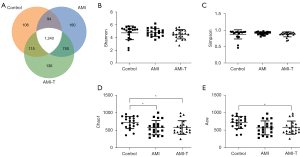
Table 3
Groups | Shannon | Simpson | Chao1 | Ace | Goods_coverage |
---|---|---|---|---|---|
Control | 4.75±1.07 | 0.88±0.14 | 721.57±172.56 | 723.44±171.09 | 0.999 |
AMI | 4.83±0.63 | 0.91±0.04 | 578.69±204.52* | 572.28±199.27 | 0.999 |
AMI-T | 4.53±0.72 | 0.89±0.08 | 581.88±195.16* | 578.66±189.42* | 0.999 |
The data shows the results of each index with mean ± standard deviation. *P<0.05 vs. control group. Control, healthy control group; AMI-T, AMI patients treatment group. AMI, acute myocardial infarction.
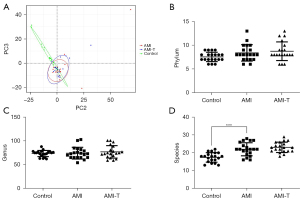
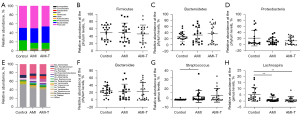
Species markers
An analysis of similarities (ANOSIM) non-parametric test showed that there were significant differences among groups with R=0.223 and a statistical probability of <0.05 (Figure 4A). To determine species differences between the groups, we used a linear discriminant analysis effect size (LEfSe) linear discriminant analysis (LDA)-based method. We used the logarithmic LDA score with significant difference set to 2 and found 5 genera, Verrucomicrobia, Bifidobacterium, Bifidobateriates, Dialister, and Collinsella, that may be fecal bacteria markers for patients with AMI (Figure 4B). In addition, after routine drug treatment, we observed significant changes in gut microbiota species by comparing the AMI group with the AMI-T group.
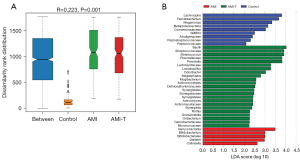
Features of microbial metabolism
TMA and SCFA represent a major class of metabolites produced from microbial metabolism of dietary components, and TMA transforms into TMAO after entering the liver. We found that levels of TMAO were much higher in the plasma of AMI patients than in healthy people, and they decreased slightly after admission, but there was no statistical significance compared with levels of before treatment (Figure 5A). SCFA showed similar levels in plasma, and the difference was not statistically significant (Figure 5B). Using Kyoto Encyclopedia of Genes and Genomes (KEGG) metabolic pathway classification, we found that the gut microbiota had a higher proportion of amino sugar, nucleotide sugar, fructose, and mannose metabolism compared to that of the healthy control group, whereas there was less microbial porphyrin and chlorophyll metabolism in AMI patients than the control group (Figure 5C).
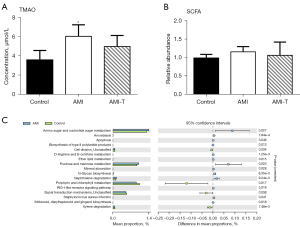
Discussion
In this study, we used 16S rRNA technology to compare the differences in fecal microbiota between AMI patients and healthy controls. The results showed that the AMI group had a different pattern of intestinal microflora compared with healthy individuals. There was no significant difference in diversity but a significant difference in the richness of the intestinal microflora. After PCI and 1 week of medical treatment (Table S1), the gut microbiota of patients with AMI showed no significant difference compared with before treatment (Figure 1B). SCFA and TMAO are direct or indirect biological products of gut microbiota and can participate in the regulation of cardiovascular disease (19-21). In our research, plasma TMAO levels were higher in AMI patients than in the healthy individuals; however, this trend was not seen for SCFAs.
Gut microbiota of human contains a large number of obligative anaerobic and few aerobic bacteria and could be identified on species level by 16S rRNA sequencing, which is a mature sequencing technology with sequencing the V3-V4 hypervariable region of 16S rDNA gene (22). Some anaerobes were killed during collection, but the bacterial species can still be identified by the residual DNA sample. 16S rRNA sequencing of bacteria have been used in the study of human diseases. Cardiovascular disease accounts for more than 40% of deaths in China and is the leading cause of mortality (8). In recent years, studies have shown that, in addition to diabetes, hypertension and other factors, an imbalance of gut microbiota and their metabolites can affect blood lipid levels promoting the development of atherosclerosis and lead to cardiovascular disease (3,23). In this study, we found that Firmicute and Bacteroidetes were two major bacterial phyla in AMI patients with average relative abundance of 50.27% and 32.2%, respectively, which is similar to that found in a case-control study of coronary artery diseases (24). However, Liu’s study showed that Bacteroidetes (59.6%) had a higher proportion than that of Firmicute (31.5%) (25). The reason for this significant difference results may be related to the Geographic factors, which is an important role for human microbiome diversity (26). Bacteroidetes and Firmicutes are two major groups of intestinal bacteria and their proportional structure can affect the intestinal microenvironment. We found that Firmicutes represented the largest microfloral component and Bacteroidetes the second largest. However, there is some controversy regarding the significance of the Firmicutes/Bacteroidetes (F/B) ratio (27), and there was no significant difference in the F/B ratio of AMI patients compared with controls in this study. In addition, two other groups showed significant differences between groups, Proteobacteria and Verrucomicrobia. The bacterial phylum Verrucomicrobia is now recognized as being ubiquitous and abundant in both soil and aquatic systems (28). Although Verrucomicrobia were significantly more abundant in AMI patients, the proportion was relatively small, and we speculated that the increase may have been due to environmental differences.
Metabolites of gut microbiota, including TMAO, SCFAs, Phenylacetyl glutamine (PAGln), are important mediator for gut microorganisms to participate in the regulation of human diseases. TMAO is the product of TMA by hepatoflavin dependent monooxygenase in the liver. Early clinical studies have shown that elevated levels of blood TMAO positively correlate with an increased risk of coronary heart disease (29). In animal study, exogenous effects caused the concentration of TMAO in the blood of mice to increase, but had no significant effect on cardiovascular disease (10). The reduction of TMAO level could enhance the stability of atherosclerotic plaque which is risk factor of AMI. At the same time, TMAO can increase the release of intracellular calcium ions (Ca2+), enhance the reactivity of platelets, and increase the risk of thrombosis (30). The results of this study show that there is a significant higher concentration of TMAO in AMI patients’ plasma than that of healthy humans. After PCI treatment, the concentrations of TMAO in patient plasmas showed a downward trend with no significant. The plasma concentration of TMAO in patients with AMI may be influenced by the disorder community of their gut microbiota. The results indicate that TMAO may be a biomarker in the development of AMI.
SCFA, including formic, acetic, propionic, isobutyric, butyric, isovaleric, and valeric acids, are common metabolites of gut microbiota and are rapidly absorbed by the hindgut, and they not only store energy but also reduce osmotic pressure. SCFA play an important role in maintaining the normal function of the large intestine and the morphology and function of colonic epithelial cells. SCFA can be associated with GPR41 and directly regulate the sympathetic nervous system (12). In recent years, interest in the “microbiota-gut-brain axis” has emerged, in which gut microbiota metabolites play an important mediating role (5). Based on the study of gut microbiota in patients with cardiovascular disease, Yu et al. proposed a “gut-brain-heart” axis, through which SCFA may be involved in cardiovascular regulation (31). It is strange that the SCFA plasma levels of AMI patients in this study were not significantly higher than those of healthy individuals. More basic research is needed to ascertain the interactions and roles of SCFA in the cardiovascular system.
The present study had some limitations. First, in this study, to reduce the interference of other factors on gut microbiota, such as drinking or smoking, we chose the participants who had no history of drinking, smoking or diabetes. However, the daily diet was not considered, which was an important confounding factor in affecting gut microbiota. Second, this study was just a small sample study and only 20 patients and 20 health control were recruited. The results of this experiment had certain limitations. Third, the study did not examine the expression of cytokines related to cardiovascular disease in peripheral and not assess the relationship of the gut microbiota and cytokine expression. In addition, analysis of changes in the gut microbiota should include changes in the number and species of microflora. However, the 16S rRNA assay used in this experiment was only able to detect differences in species, but cannot quantitatively analyze the proportion differences in the number of gut microbiota. In summary, our study found that the gut microbiota of AMI have a difference with that of health people and the microbial metabolites may have role in AMI development.
Conclusions
By comparing the gut microbiota of AMI patients and healthy people, it was found that the richness of gut microbiota of AMI patients was significantly reduced, but the content of metabolites, such as SCFA and TMAO, of gut microbiota in peripheral blood did not differ from that in the healthy group. Drug treatment and PCI treatment had no significant effect on the gut microbiota of patients with AMI. Changes to the structure of gut microbiota in AMI patients should be taken seriously. In the future, the treatment of patients with AMI after PCI should incorporate the regulation of gut microbiota.
Acknowledgments
We would like to thank all medical staff and patients in the Department of Cardiology, Yijishan Hospital, Wannan Medical College for providing us with samples for this study.
Funding: This work was supported by the Key Project of Anhui Province University Outstanding Young Talents Support Fund (gzyqZD2021143), Development of Modern Medical and Pharmaceutical Industry in Anhui Province project (2021/2022) and the National Natural Science Foundation of China (81803035).
Footnote
Reporting Checklist: The authors have completed the MDAR reporting checklist. Available at https://atm.amegroups.com/article/view/10.21037/atm-22-5671/rc
Data Sharing Statement: Available at https://atm.amegroups.com/article/view/10.21037/atm-22-5671/dss
Conflicts of Interest: All authors have completed the ICMJE uniform disclosure form (available at https://atm.amegroups.com/article/view/10.21037/atm-22-5671/coif). The authors have no conflicts of interest to declare.
Ethical Statement: The authors are accountable for all aspects of the work in ensuring that questions related to the accuracy or integrity of any part of the work are appropriately investigated and resolved. The study was conducted in accordance with the Declaration of Helsinki (as revised in 2013). The study was approved by ethics board of Wannan Medical College (No. 2020371) and informed consent was taken from all the patients.
Open Access Statement: This is an Open Access article distributed in accordance with the Creative Commons Attribution-NonCommercial-NoDerivs 4.0 International License (CC BY-NC-ND 4.0), which permits the non-commercial replication and distribution of the article with the strict proviso that no changes or edits are made and the original work is properly cited (including links to both the formal publication through the relevant DOI and the license). See: https://creativecommons.org/licenses/by-nc-nd/4.0/.
References
- Tibaut M, Mekis D, Petrovic D. Pathophysiology of Myocardial Infarction and Acute Management Strategies. Cardiovasc Hematol Agents Med Chem 2017;14:150-9. [Crossref] [PubMed]
- Trongtorsak A, Kewcharoen J, Thangjui S, et al. Admission hyperglycemia in acute myocardial infarction is associated with an increased risk of arrhythmias: A systematic review and meta-analysis. J Arrhythm 2022;38:307-15. [Crossref] [PubMed]
- Davar D, Zarour HM. Facts and Hopes for Gut Microbiota Interventions in Cancer Immunotherapy. Clin Cancer Res 2022;28:4370-84. [Crossref] [PubMed]
- Tilg H, Adolph TE, Trauner M. Gut-liver axis: Pathophysiological concepts and clinical implications. Cell Metab 2022;34:1700-18. [Crossref] [PubMed]
- de Wouters d'Oplinter A, Huwart SJP, Cani PD, et al. Gut microbes and food reward: From the gut to the brain. Front Neurosci 2022;16:947240. [Crossref] [PubMed]
- Whitman WB, Coleman DC, Wiebe WJ. Prokaryotes: the unseen majority. Proc Natl Acad Sci U S A 1998;95:6578-83. [Crossref] [PubMed]
- Zimmermann M, Zimmermann-Kogadeeva M, Wegmann R, et al. Mapping human microbiome drug metabolism by gut bacteria and their genes. Nature 2019;570:462-7. [Crossref] [PubMed]
- Ley RE, Peterson DA, Gordon JI. Ecological and evolutionary forces shaping microbial diversity in the human intestine. Cell 2006;124:837-48. [Crossref] [PubMed]
- Qin J, Li R, Raes J, et al. A human gut microbial gene catalogue established by metagenomic sequencing. Nature 2010;464:59-65. [Crossref] [PubMed]
- Ufnal M, Zadlo A, Ostaszewski R. TMAO: A small molecule of great expectations. Nutrition 2015;31:1317-23. [Crossref] [PubMed]
- Zhang Q, Guo X, Xie C, et al. Unraveling the metabolic pathway of choline-TMA-TMAO: Effects of gypenosides and implications for the therapy of TMAO related diseases. Pharmacol Res 2021;173:105884. [Crossref] [PubMed]
- Kimura I, Inoue D, Maeda T, et al. Short-chain fatty acids and ketones directly regulate sympathetic nervous system via G protein-coupled receptor 41 (GPR41). Proc Natl Acad Sci U S A 2011;108:8030-5. [Crossref] [PubMed]
- Drożdż K, Nabrdalik K, Hajzler W, et al. Metabolic-Associated Fatty Liver Disease (MAFLD), Diabetes, and Cardiovascular Disease: Associations with Fructose Metabolism and Gut Microbiota. Nutrients 2021;14:103. [Crossref] [PubMed]
- Yang T, Santisteban MM, Rodriguez V, et al. Gut dysbiosis is linked to hypertension. Hypertension 2015;65:1331-40. [Crossref] [PubMed]
- Verhaar BJH, Prodan A, Nieuwdorp M, et al. Gut Microbiota in Hypertension and Atherosclerosis: A Review. Nutrients 2020;12:2982. [Crossref] [PubMed]
- Han Y, Gong Z, Sun G, et al. Dysbiosis of Gut Microbiota in Patients With Acute Myocardial Infarction. Front Microbiol 2021;12:680101. [Crossref] [PubMed]
- Dong C, Yang Y, Wang Y, et al. Gut microbiota combined with metabolites reveals unique features of acute myocardial infarction patients different from stable coronary artery disease. J Adv Res 2022; Epub ahead of print. [Crossref] [PubMed]
- Meyer KA, Benton TZ, Bennett BJ, et al. Microbiota-Dependent Metabolite Trimethylamine N-Oxide and Coronary Artery Calcium in the Coronary Artery Risk Development in Young Adults Study (CARDIA). J Am Heart Assoc 2016;5:e003970. [Crossref] [PubMed]
- Bartolomaeus H, Balogh A, Yakoub M, et al. Short-Chain Fatty Acid Propionate Protects From Hypertensive Cardiovascular Damage. Circulation 2019;139:1407-21. [Crossref] [PubMed]
- Chambers ES, Preston T, Frost G, et al. Role of Gut Microbiota-Generated Short-Chain Fatty Acids in Metabolic and Cardiovascular Health. Curr Nutr Rep 2018;7:198-206. [Crossref] [PubMed]
- Shi W, Huang Y, Yang Z, et al. Reduction of TMAO level enhances the stability of carotid atherosclerotic plaque through promoting macrophage M2 polarization and efferocytosis. Biosci Rep 2021;41:BSR20204250. [Crossref] [PubMed]
- Johnson JS, Spakowicz DJ, Hong BY, et al. Evaluation of 16S rRNA gene sequencing for species and strain-level microbiome analysis. Nat Commun 2019;10:5029. [Crossref] [PubMed]
- Yu L, Meng G, Huang B, et al. A potential relationship between gut microbes and atrial fibrillation: Trimethylamine N-oxide, a gut microbe-derived metabolite, facilitates the progression of atrial fibrillation. Int J Cardiol 2018;255:92-8. [Crossref] [PubMed]
- Toya T, Corban MT, Marrietta E, et al. Coronary artery disease is associated with an altered gut microbiome composition. PLoS One 2020;15:e0227147. [Crossref] [PubMed]
- Liu C, Sun Z, Shali S, et al. The gut microbiome and microbial metabolites in acute myocardial infarction. J Genet Genomics 2022;49:569-78. [Crossref] [PubMed]
- Pasolli E, Asnicar F, Manara S, et al. Extensive Unexplored Human Microbiome Diversity Revealed by Over 150,000 Genomes from Metagenomes Spanning Age, Geography, and Lifestyle. Cell 2019;176:649-662.e20. [Crossref] [PubMed]
- Mariat D, Firmesse O, Levenez F, et al. The Firmicutes/Bacteroidetes ratio of the human microbiota changes with age. BMC Microbiol 2009;9:123. [Crossref] [PubMed]
- Chiang E, Schmidt ML, Berry MA, et al. Verrucomicrobia are prevalent in north-temperate freshwater lakes and display class-level preferences between lake habitats. PLoS One 2018;13:e0195112. [Crossref] [PubMed]
- Guasti L, Galliazzo S, Molaro M, et al. TMAO as a biomarker of cardiovascular events: a systematic review and meta-analysis. Intern Emerg Med 2021;16:201-7. [Crossref] [PubMed]
- Zhu W, Gregory JC, Org E, et al. Gut Microbial Metabolite TMAO Enhances Platelet Hyperreactivity and Thrombosis Risk. Cell 2016;165:111-24. [Crossref] [PubMed]
- Yu Z, Han J, Chen H, et al. Oral Supplementation With Butyrate Improves Myocardial Ischemia/Reperfusion Injury via a Gut-Brain Neural Circuit. Front Cardiovasc Med 2021;8:718674. [Crossref] [PubMed]
(English Language Editor: J. Jones)