SDHB reduction promotes oral lichen planus by impairing mitochondrial respiratory function
Highlight box
Key findings
• SDHB reduction promotes OLP by impairing mitochondrial respiratory function.
What is known and what is new?
• SDHB expression is associated with the malignant transformation from OLP to OSCC.
• SDHB expression was indeed significantly reduced with OLP occurrence, accompanied by the change of cell biogenetics to glycolytic metabolism from aerobic respiration, providing the plausible explanation for possible cellular deterioration and transformation to cancer.
What is the implication, and what should change now?
• SDHB is an effective indicator of OLP-induced carcinogenesis and may be a potential therapeutic target.
Introduction
Oral lichen planus (OLP) is a chronic inflammatory disorder that affects oral mucosa, with various clinical manifestations including papular, reticular, plaque-like, ulcerative, and atrophic lesions (1,2). Despite the lack of reliable epidemiological data, OLP is thought to be common, affecting about 1.5% of all people (3). Nowadays, the pathogenesis of OLP remains unclear, and microbial dysbiosis, innate and adaptive immunity and inflammation has been proposed to play crucial roles (4-6). The lesions of OLP lesions are chronic, rarely spontaneously resolve (7), and they always cause severe pain, morbidity, and undergo malignant transformation (8,9). OLP has been suggested as a potential a precancerous event for oral squamous cell carcinoma (OSCC), the common oral cancer subtype that comprises over 90% of cases (10). OLP has diverse molecular pathogenic mechanisms according to different etiologic factors and genotoxic stimuli, such as exposure to toxicity, viral infections, and metabolic factors (10,11). Therefore, there is an urgent need to understand the mechanisms underlying the OLP-related malignant transformation and provide targets for OLP progression.
Succinate dehydrogenase enzyme (SDH), the heterotetrameric protein with high conservation level, comprises 4 subunits: SDHA/B/C/D, which is responsible for catalyzing succinate oxidation to fumarate during tricarboxylic acid (TCA) cycle (12,13). Of them, SDHA and SDHB have catalytic effects, whereas the others assist in anchoring the former 2 to the mitochondrial membrane (14-16). Compared to the other 3 subunits, SDHB mutations are more aggressive and have a higher metastasis rate (17,18). The damage of SDHB function induces mitochondrial dysfunction, rewired metabolism, and pseudohypoxic signaling, all of which play important roles in promoting tumor progression. Deactivation of SDHB is extensively suggested to be associated with cancer malignancy (19,20), whereas its somatic mutations are frequently related to pheochromocytoma, paraganglioma, renal cell carcinoma, and gastrointestinal tumors (21,22). Apart from mutation inactivation, decreased expression of SDH subunits can be detected within multiple cancers including that of the brain and breast (23,24). Further studies show that intratumoral hypoxia, which is in favor of cancer cell proliferation, invasion and metastasis, inhibits the expression of SDHB, and the decreased expression of SDHB also promotes the hypoxia-induced injury (25,26). Meanwhile, as the mediation between oxidative phosphorylation and electron transport, SDHB regulates mitochondrial ROS production and drives macrophage inflammatory, including interleukin (IL)-1β, IL-18 release (27). However, the molecular mechanisms leading to SDH impairment in precancerous lesions remain largely unknown. Besides, impairment of SDHB activity leads to accumulation of the succinate, which could drive aberrant activation of transcription factors and global epigenetic reprogramming (28,29). Recently, metabolic alterations have been suggested to be related to OLP progression (30,31). Therefore, therapeutic strategies targeting mitochondrial metabolism might have important implications for the treatment of OLP progression.
In current study, we found that altered SDHB expression was closely associated with OLP progression. Mechanistically, decreased SDHB led to accumulation of succinate, which then promoted hypoxia-inducible factor (HIF)-1α activation and impaired mitochondrial respiration. Our study suggests that SDHB overexpression is the possible treatment for OLP cases showing decreased SDHB expression. We present the following article in accordance with the MDAR reporting checklist (available at https://atm.amegroups.com/article/view/10.21037/atm-22-5999/rc).
Methods
Patients and tissue specimens
This work involved a total of 23 OLP samples obtained from Huashan Hospital, Fudan University during 2018 to 2020. The study was conducted in accordance with the Declaration of Helsinki (as revised in 2013). The study was approved by the Institutional Research Ethics Committee of Huashan Hospital, Fudan University (No. 2019-589) and informed consent was taken from all the patients. The information of study participants was shown in Table S1.
Cells and culture
Under sterile conditions, samples of reticular lesion tissue measuring 0.6 cm × 1.0 cm were collected from OLP cases. Later, sterile phosphate-buffered saline (PBS) containing 1% penicillin-streptomycin (PS; Gibco, Waltham, MA, USA) was added to immerse the samples for removing blood. Thereafter, keratinocyte serum-free medium (KSFM) was added to culture OLP type-I keratinocytes in vitro. Cells of 3–4 passages were harvested for further analysis. Meanwhile, HaCAT cells were purchased from the Shanghai Institute of Biochemistry and Cell Biology, Chinese Academy of Sciences (Shanghai, China) and cultivated with Dulbecco’s modified Eagle medium (DMEM) (Invitrogen, Carlsbad, CA, USA) containing 10% fetal bovine serum (FBS; HyClone, Logan, UT, USA) under 5% concentration of CO2 and 37 ℃ conditions. To mimic the hypoxic and inflammatory processes in epidermal injury, we stimulated HaCAT cells with 5 µg/mL LPS.
Western blot (WB) assay
The cell lysis buffer that contained protease/phosphorylase inhibitors was added to extract total cellular protein. Thereafter, 5% defatted milk supplemented within tris-buffered saline-Tween 20 (TBST) was supplemented to block membranes, followed by primary antibody incubation, including SDHB (ab178423; Abcam, Cambridge, MA, USA), hexokinase (HK)1 [Cell Signaling Technology (CST), 2024; CST, Danvers, MA, USA], HK2 (CST, 2867; CST), HIF-1α (ab51608; Abcam), matrix metallopeptidase 9 (MMP9) (ab194316; Abcam), pyruvate kinase M2 (PKM2) (ab150377; Abcam), glucose transporter type 1 (GLUT1) (ab115730; Abcam), apoptosis-associated speck-like protein containing a CARD (ASC) (AG-25B-0006PF-C100; Adipogen, San Diego, CA, USA), NOD-like receptor family pyrin domain containing 3 (NLRP3) (AG-20B-0014-C100; Adipogen), goat anti-mouse horseradish peroxidase (HRP) (ab97023; Abcam) and goat anti-rabbit HRP (ab6721; Abcam). Membranes were rinsed with TBST prior to further secondary antibody incubation, with β-actin as the endogenous reference.
RNA extraction and quantitative reverse transcription polymerase chain reaction (qRT-PCR)
This work adopted Trizol reagent (Invitrogen, USA) for extracting total RNAs in line with specific instructions. Table 1 displays the sequences of all primers. Total RNA (1 mg) was weighed to synthesize complementary DNA (cDNA) with the cDNA synthesis kit (Takara, Madison, WI, USA). Finally, SYBR Supermix (Takara) was employed for qRT-PCR assay.
Table 1
Gene | Forward primer | Reverse primer |
---|---|---|
SDHB | 5'-CATCAATGGAGGCAACACTCT-3' | 5'-CAAATCGGGAACAAGATCCTT-3' |
GLUT1 | 5'-CACCACCTCACTCCTGTTACT-3' | 5'-CATCCAAACCTCCTACCCT-3' |
PKM2 | 5'-CCATTGGTCATCAGGTTTCT-3' | 5'-TTCGGTGGGACTAAATTCTG-3' |
MMP9 | 5'-GCTGGGCTTAGATCATTCCTC-3' | 5'-ATTCACGTCGTCCTTATGCAA-3' |
NLRP3 | 5'-TGGACTATTTCCCCAAGATTG-3' | 5'-CCCGATGACAGTTCTCAATG-3' |
ASC | 5'-CCACCAACCCAAGCAAGATG-3' | 5'-CTCCAGGTCCTCCACCAGGTAG-3' |
qRT-PCR, quantitative reverse transcription polymerase chain reaction; SDHB, succinate dehydrogenase enzyme B; GLUT1, glucose transporter type 1; PKM2, pyruvate kinase M2; MMP9, matrix metallopeptidase 9; NLRP3, NOD-like receptor family pyrin domain containing 3; ASC, apoptosis-associated speck-like protein containing a CARD.
Immunohistochemical (IHC) assay
Tissues were rinsed with PBS thrice (5 min each) and then immersed for 15 minutes in pre-chilled alcohol prior to blocking by PBS that included 0.1% Triton X-100 and 1% bovine serum albumin (BSA) under ambient temperature for 1 hour. Thereafter, the primary antibody was added to incubate tissues at 4 ℃ overnight. Tissues were then rinsed with PBS thrice (10 min each), followed by 1 hour of incubation using SDHB (ab178423), anti-rabbit immunoglobulin G (IgG) under ambient temperature. Then, tissues were rinsed in PBS thrice (10 min each), followed by 4 minutes 100 µl DAB substrate solution incubation (Ventana/Roche; Tucson, AZ, USA). Last, tissues were stained by hematoxylin and dehydrated with increasing ethanol concentrations. Afterwards, the Fluoromount Aqueous Mounting Medium (F4680; Sigma, St. Louis, MO, USA) was added to seal the coverslip. The laser scanning confocal unit (Zeiss LSM 710; Carl Zeiss, Jena, Germany) was utilized to analyze each slice.
Firefly luciferase adenosine triphosphate (ATP) assay
An ATP Bioluminescence Assay Kit CLSII (Roche Applied Science, Mannheim, Germany) was employed for measuring total ATP in cells in line with specific protocols.
Mitochondrial membrane potential (MMP) assay
A JC-10 assay kit (40752ES60; Yeasen Biotechnology, Shanghai, China) was utilized to monitor MMP. Briefly, JC-10 was added to incubate cells for a 30-min period, followed by rinsing with a buffer twice. The fluorescence was detected under flow cytometry (FCM) using a CytoFLEX (Beckman Coulter, Brea, CA, USA).
Reactive oxygen species (ROS) measurement
After 48 hours, dichloro-dihydro-fluorescein diacetate (DCFH-DA; 10 µM) was added into cells within the phenol red-free culture medium (50101ES01; Yeasen Biotechnology) for a 30-min period. After staining, FCM was conducted to analyze cells with the CytoFLEX (Beckman Coulter).
Glucose uptake assay
The glucose uptake was detected by Cell Meter 2-NBDG kit (AAT BioQuest, Sunnvale, CA, USA). After seeding, the cells were treated with glucose-free Krebs-Ringer bicarbonate (KRB) buffer containing 129 mM NaCl, 10 mM HEPES, 5 mM NaHCO3, 4.8 mM KCl, 1.2 mM MgSO4, 1.2 mM KH2PO4, and 1.0 mM CaCl2, together with 0.1% BSA (pH 7.4) for 15 minutes, followed by incubation with the freshly prepared KRB buffer that contained a D-glucose fluorescent analogue 2-N-(7-nitrobenz-2-oxa-1,3-diazol-4-yl) amino-2-deoxy-D-glucose (600 mM, 2-NBDG; Molecular Probes Inc., Eugene, OR, USA) and 3.3 Mm glucose for 10–30 min. Finally, the plate reader (Tecan, Männedorf, Switzerland) was utilized to detect fluorescence intensities at the excitation and emission wavelengths of 485 and 530 nm, separately.
Statistical analysis
Data are representative of at least three independent experiments. Results were represented to be means ± standard error of the mean (SEM) and analyzed by one-way analysis of variance (ANOVA) using GraphPad Prism software (GraphPad Software, San Diego, CA, USA). Two groups were compared with independent group t-tests. *P<0.05, **P<0.01, and ***P<0.001 indicated statistical significance.
Results
SDHB expression decreased and succinate accumulated in OLP patients
In our previous study, we found that TCA cycle was suppressed during the development of OLP (30). SDHB, an important enzymatic constituent in the mitochondrial enzyme SDH (18), is constructively distributed among different species, and we first examined SDHB expression in tissues from OLP patients. By IHC, we found that OLP tissues exhibited significantly reduced SDHB expression compared with normal tissues (Figure 1A). SDHB messenger RNA (mRNA) and protein levels were measured among 23 OLP patients by qRT-PCR and WB. Normal oral mucosa (NOM) served as a control. According to Figure 1B,1C, OLP samples showed significantly decreased SDHB mRNA levels, and WB showed similar results. Expectedly, decreased SDHB levels within OLP samples resulted in damaged succinate activity as well as its later accumulation (Figure 1D). Taken together, SDHB showed down-regulation among OLP cases, and it was indicative of certain biological activity in pathology.
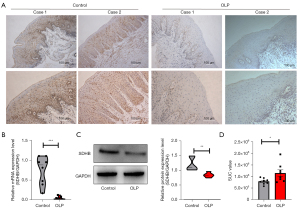
SDHB is crucially involved in the prognosis of lipopolysaccharide- (LPS-) and CoCl2-stimulated OLP cell model
OLP is a process in which tissue hypoxia and chronic inflammatory infiltration lead to the deterioration of OLP keratinocytes (32,33). We first stimulated HaCAT cells with LPS and CoCl2 to mimic the hypoxic and inflammatory processes in epidermal injury. Under the stimulation of 5 µg/mL LPS, the expression of SDHB decreased within the prolongation of inflammation time. Under the stimulation of CoCl2 at different concentrations, the expression of SDHB was also reduced within the increase of the degree of hypoxia (Figure 2A,2B). To further gain a real picture of OLP development, we isolated OLP keratinocytes. Likewise, after stimulation with LPS and CoCl2 in OLP keratinocytes, we monitored the expression level of SDHB at different time points or different concentrations. As shown in Figure 2C,2D, in the LPS model, 5 µg/mL LPS caused a significant down-regulation of SDHB, reaching the highest inhibitory effect at 24 hours. The same results were observed in the hypoxia model (Figure 2C,2D).
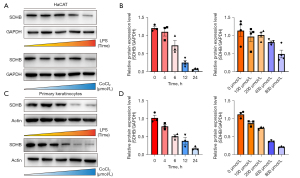
SDHB induced mitochondrial injury and bioenergetic changes
SDHB has been identified as an important enzymatic constituent in SDH, which shows extensive expression among different species and is responsible for succinate oxidization into fumarate during TCA cycle together with electron feeding in the mitochondrial respiratory chain to produce ATP (34,35). To evaluate the changes in bioenergetic metabolism caused by reduced SDHB expression during OLP, we assessed the cellular ATP level and ROS level, respectively. The ATP level was detected with the firefly luciferase ATP assay which revealed mild elevation during inflammation and hypoxia compared with the normal group (Figure 3A). Since ROS are generated during aerobic respiration, the reduced respiratory activity may thereby decrease ROS generation. To measure whether SDHB decreased mitochondrial respiration, this work utilized DCFH-DA, the fluorescent probes, to measure ROS contents. As expected, low staining intensity with DCFH-DA were detected in the inflammatory and hypoxia processes (Figure 3B,3C). Based on the above findings, we deduced that SDHB deletion impairs mitochondrial activity while reducing respiratory activity, which was evaluated by calculating MMP (Δψm). It was found that the membrane potential decreased with decreasing SDHB expression, suggesting that SDHB inhibition impairs the progression of the TCA cycle and reduces mitochondrial respiration (Figure 3D,3E). The above results imply that changes in SDHB level impair mitochondrial respiration, thus activating and up-regulating cytoplasmic glycolysis to generate ATP in cells. We further examined glucose uptake by adding the fluorescent glucose analog 2-NBDG to the medium to test our hypothesis. The results showed increased glucose uptake as SDHB expression decreased in comparison with normal controls (Figure 3F). Collectively, SDHB expression alteration impairs mitochondrial function and induces a switch in bioenergetics to glycolytic metabolism from aerobic respiration.
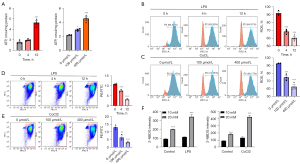
SDHB enhances HIF-1α and glycolytic metabolism
To further explore our hypothesis, we analyzed the extent of metabolic and inflammatory changes during OLP progression. The expression of genes involved in bioenergy metabolism as well as inflammation, including glycolysis, and inflammatory pathways, was examined (Figure 4A). In both models, we first found that the expression of HIF-1α was up-regulated, indicating that reduction of SDHB induces SDH deficiency in cells and changes in oxygen content in the cellular environment, resulting in the stabilization of HIF-1α. At the same time, we found that the expression of HK2 was decreased whereas that of HK1 was greatly elevated (Figure 4A). By contrast, inflammasome expression slightly decreased correspondingly, the level of inflammation in this process was only slightly increased, and the inflammatory syndrome gradually subsided over time (Figure 4B). These results support that SDHB mediates glycolysis by promoting the stabilization of HIF-1α.
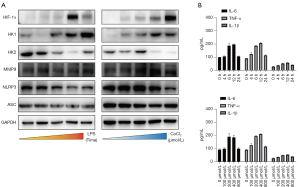
SDHB overexpression switches bioenergetic metabolism during OLP process
To further investigate whether SDHB protein was related to OLP development, we examined how SDHB up-regulation affected OLP cells by adopting overexpression plasmids (Figure 5A). To assess how SDHB up-regulation affected bioenergetic metabolism, firefly luciferase assay was conducted to determine ATP levels within cells with SDHB over-expression. Cells with SDHB over-expression showed significantly decreased ATP contents relative to those transfected with vector (Figure 5B). To analyze how SDHB up-regulation affected aerobic respiration, the fluorescent probe DCFH-DA was used to measure ROS formation. In contrast, higher staining intensity of DCFH-DA was measured within cells with SDHB over-expression relative to those transfected with vector (Figure 5C). The glucose absorption capacity of cells with SDHB over-expression was examined with the fluorescent glucose analog 2-NBDG loading assay. Glucose uptake was slightly reduced in SDHB-overexpressing cells relative to cells transfected with vector and mock cells (Figure 5D). Consequently, SDHB overexpression in OLP cells disrupted TCA cycle progression.
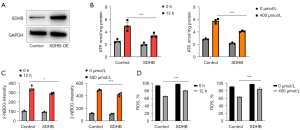
SDHB is a potential therapeutic target for OLP patients
Additional NOM and OLP mucosa tissues were collected to verify changes in glycolysis and HIF-1α pathways. The WB analysis showed that PKM2, GLUT1, and MMP9 were up-regulated in OLP tissues compared with NOM, whereas HK2 expression was down-regulated (Figure 6A,6B). The same results were obtained at the mRNA level (Figure 6C).
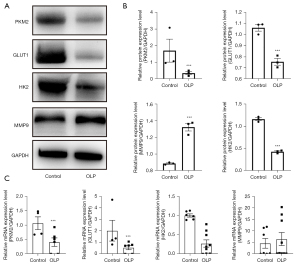
Discussion
Our results provide a potential mechanism for the correlation between OLP development and mitochondrial dysfunction. In this study, several cellular models of OLP development were established in vitro, and SDHB expression was indeed significantly reduced with OLP occurrence, accompanied by a large amount of energy production. The stable reduction of SDHB led to cellular oxygen environmental changes and mitochondrial respiration impairment, thereby promoting the transition from aerobic respiration to glycolysis (36,37). In the process of cancer, the significant enhancement of glycolysis is the main means to provide energy for tumor proliferation and development (35). As Warburg described, compared to surrounding normal tissue, cancer has an increased reliance on the glycolytic pathway, and this is required to produce energy and synthesize macromolecules for sustaining the fast cellular growth with large amounts of oxygen (38).
According to our results, SDHB down-regulation within OLP cells and the change of cell biogenetics from aerobic respiration to glycolytic metabolism provide the plausible explanation for possible cellular deterioration and transformation to cancer. The SDHB low expression cells had nearly completely lost MMP and reduced ROS concentrations, indicating severe mitochondrial respiration disruption. Our findings also suggest that as SDHB decreases, cellular glucose uptake increases, changes in related biological genetic enzymes further provide a Warburg-like effect that has occurred in OLP as precancerous lesions. The overexpression of SDHB resulted in an unpredictable decrease in ATP production and Δψm, but increased ROS generation. Besides, cells overexpressing SDHB exhibited slightly decreased glucose absorption, suggesting that overexpression of SDHB induces some reduction in glycolytic activity. However, based on careful analysis of the reduced levels of Δψm in SDHB overexpression or silencing cells, the reduced Δψm in cells with SDHB overexpression significantly decreased relative to cells with SDHB silencing. Therefore, SDHB up-regulation may have a mild impact on the damaging TCA cycle, later leading to glycolytic pathway dysregulation.
Although the exact mechanism by which SDH disruption in mitochondrial metabolism leads to tumorigenesis is unclear, it was recently reported that SDHB mutations could lead to dysfunction of the respiratory chain together with stabilization of HIFs, followed by the pseudohypoxic state (39). It may promote angiogenesis while facilitating tumorigenesis, but it does not explain all transformation of phenotypes, besides, its associated mechanisms remain to be elucidated. SDHB loss or reduction has a critical effect on the respiratory chain and TCA cycle, which causes bioenergetic shift to cytosolic glycolysis from mitochondrial respiration (32,34,37). Tumor cells depend on aerobic glycolysis to generate ATP, which is used as the antitumor therapeutic target. Inhibiting glycolysis can avoid ATP generation and reduce tumor cell proliferation. Therefore, attenuated glucose metabolism in cancer cells has been the possible new area to develop the new antitumor treatments. Cases with SDHB down-regulation within precancerous lesions and dismal prognostic outcomes are considered for drug treatments targeting the Warburg effect (40,41). From a clinical perspective, our current findings suggest SDHB as an effective indicator of OLP-induced carcinogenesis and a possible therapeutic target.
Conclusions
We found that altered SDHB expression was closely associated with OLP progression. At cell level, decreased SDHB led to accumulation of succinate, which then promoted HIF-1α activation and impaired mitochondrial respiration. Our study suggests that SDHB reduction promotes OLP by impairing mitochondrial respiratory function.
Acknowledgments
Funding: This work was supported by grants from the National Natural Science Foundation of China (Nos. 81901008, 81470736).
Footnote
Reporting Checklist: The authors have completed the MDAR reporting checklist. Available at https://atm.amegroups.com/article/view/10.21037/atm-22-5999/rc
Data Sharing Statement: Available at https://atm.amegroups.com/article/view/10.21037/atm-22-5999/dss
Conflicts of Interest: All authors have completed the ICMJE uniform disclosure form (available at https://atm.amegroups.com/article/view/10.21037/atm-22-5999/coif). The authors have no conflicts of interest to declare.
Ethical Statement: The authors are accountable for all aspects of the work in ensuring that questions related to the accuracy or integrity of any part of the work are appropriately investigated and resolved. The study was conducted in accordance with the Declaration of Helsinki (as revised in 2013). The study was approved by the Institutional Research Ethics Committee of Huashan Hospital, Fudan University (No. 2019-589) and informed consent was taken from all the patients.
Open Access Statement: This is an Open Access article distributed in accordance with the Creative Commons Attribution-NonCommercial-NoDerivs 4.0 International License (CC BY-NC-ND 4.0), which permits the non-commercial replication and distribution of the article with the strict proviso that no changes or edits are made and the original work is properly cited (including links to both the formal publication through the relevant DOI and the license). See: https://creativecommons.org/licenses/by-nc-nd/4.0/.
References
- Arduino PG, Gambino A, Macciotta A, et al. Population-based cohort study to assess the gingival lesions in 1319 patients with lichen planus. Oral Dis 2022; Epub ahead of print. [Crossref]
- Daume L, Kreis C, Bohner L, et al. Clinical characteristics of oral lichen planus and its causal context with dental restorative materials and oral health-related quality of life. BMC Oral Health 2021;21:262. [Crossref] [PubMed]
- Oivio UM, Pesonen P, Ylipalosaari M, et al. Prevalence of oral mucosal normal variations and lesions in a middle-aged population: a Northern Finland Birth Cohort 1966 study. BMC Oral Health 2020;20:357. [Crossref] [PubMed]
- Chen J, Liu K, Sun X, et al. Microbiome landscape of lesions and adjacent normal mucosal areas in oral lichen planus patient. Front Microbiol 2022;13:992065. [Crossref] [PubMed]
- Soler-Ferran D, Louis F, Woo SB, et al. Infiltration of Mature KLRG1 Expressing Cytotoxic T Cells in Oral Lichen Planus. Head Neck Pathol 2022;16:1124-9. [Crossref] [PubMed]
- Wang R, Zhang X, Wang S. Differential genotypes of TNF-α and IL-10 for immunological diagnosis in discoid lupus erythematosus and oral lichen planus: A narrative review. Front Immunol 2022;13:967281. [Crossref] [PubMed]
- Zotti F, Nocini R, Capocasale G, et al. Oral Lichen Planus: risk factors of malignant transformation and follow up. Ten years retrospective study. J Clin Exp Dent 2021;13:e630-6. [Crossref] [PubMed]
- Chiang CP, Yu-Fong Chang J, Wang YP, et al. Oral lichen planus - Differential diagnoses, serum autoantibodies, hematinic deficiencies, and management. J Formos Med Assoc 2018;117:756-65. [Crossref] [PubMed]
- Arduino PG, Magliano A, Gambino A, et al. Risk of Malignant Transformation in 3173 Subjects with Histopathologically Confirmed Oral Lichen Planus: A 33-Year Cohort Study in Northern Italy. Cancers (Basel) 2021;13:5740. [Crossref] [PubMed]
- Chiu YW, Su YF, Yang CC, et al. Is OLP potentially malignant? A clue from ZNF582 methylation. Oral Dis 2021; Epub ahead of print. [Crossref]
- Yang Q, Guo B, Sun H, et al. Identification of the key genes implicated in the transformation of OLP to OSCC using RNA-sequencing. Oncol Rep 2017;37:2355-65. [Crossref] [PubMed]
- Moosavi B, Zhu XL, Yang WC, et al. Genetic, epigenetic and biochemical regulation of succinate dehydrogenase function. Biol Chem 2020;401:319-30. [Crossref] [PubMed]
- Armstrong N, Storey CM, Noll SE, et al. SDHB knockout and succinate accumulation are insufficient for tumorigenesis but dual SDHB/NF1 loss yields SDHx-like pheochromocytomas. Cell Rep 2022;38:110453. [Crossref] [PubMed]
- Tseng PL, Wu WH, Hu TH, et al. Decreased succinate dehydrogenase B in human hepatocellular carcinoma accelerates tumor malignancy by inducing the Warburg effect. Sci Rep 2018;8:3081. [Crossref] [PubMed]
- Huang S, Millar AH. Succinate dehydrogenase: the complex roles of a simple enzyme. Curr Opin Plant Biol 2013;16:344-9. [Crossref] [PubMed]
- Dona M, Waaijers S, Richter S, et al. Loss of sdhb in zebrafish larvae recapitulates human paraganglioma characteristics. Endocr Relat Cancer 2021;28:65-77. [Crossref] [PubMed]
- Andrews KA, Ascher DB, Pires DEV, et al. Tumour risks and genotype-phenotype correlations associated with germline variants in succinate dehydrogenase subunit genes SDHB, SDHC and SDHD. J Med Genet 2018;55:384-94. [Crossref] [PubMed]
- Gill AJ. Succinate dehydrogenase (SDH)-deficient neoplasia. Histopathology 2018;72:106-16. [Crossref] [PubMed]
- Liu Y, Pang Y, Zhu B, et al. Therapeutic Targeting of SDHB-Mutated Pheochromocytoma/Paraganglioma with Pharmacologic Ascorbic Acid. Clin Cancer Res 2020;26:3868-80. [Crossref] [PubMed]
- Chen L, Liu T, Zhang S, et al. Succinate dehydrogenase subunit B inhibits the AMPK-HIF-1α pathway in human ovarian cancer in vitro. J Ovarian Res 2014;7:115. [Crossref] [PubMed]
- Assadipour Y, Sadowski SM, Alimchandani M, et al. SDHB mutation status and tumor size but not tumor grade are important predictors of clinical outcome in pheochromocytoma and abdominal paraganglioma. Surgery 2017;161:230-9. [Crossref] [PubMed]
- Jochmanova I, Wolf KI, King KS, et al. SDHB-related pheochromocytoma and paraganglioma penetrance and genotype-phenotype correlations. J Cancer Res Clin Oncol 2017;143:1421-35. [Crossref] [PubMed]
- Kim S, Kim DH, Jung WH, et al. Succinate dehydrogenase expression in breast cancer. Springerplus 2013;2:299. [Crossref] [PubMed]
- Bardella C, Pollard PJ, Tomlinson I. SDH mutations in cancer. Biochim Biophys Acta 2011;1807:1432-43. [Crossref] [PubMed]
- Kang K, Wang L, Yu K, et al. Z-scheme MoS(2)/Co(3)S(4)@PEG nanoflowers: Intracellular NIR-II photocatalytic O(2) production facilitating hypoxic tumor therapy. Biomater Adv 2023;144:213168. [Crossref] [PubMed]
- Liu Y, Xue C, Lu H, et al. Hypoxia causes mitochondrial dysfunction and brain memory disorder in a manner mediated by the reduction of Cirbp. Sci Total Environ 2022;806:151228. [Crossref] [PubMed]
- Chen J, Zhang J, Wu J, et al. Low shear stress induced vascular endothelial cell pyroptosis by TET2/SDHB/ROS pathway. Free Radic Biol Med 2021;162:582-91. [Crossref] [PubMed]
- Mills EL, Kelly B, Logan A, et al. Succinate Dehydrogenase Supports Metabolic Repurposing of Mitochondria to Drive Inflammatory Macrophages. Cell 2016;167:457-70.e13. [Crossref] [PubMed]
- Moog S, Lussey-Lepoutre C, Favier J. Epigenetic and metabolic reprogramming of SDH-deficient paragangliomas. Endocr Relat Cancer 2020;27:R451-63. [Crossref] [PubMed]
- Yang Q, Sun H, Wang X, et al. Metabolic changes during malignant transformation in primary cells of oral lichen planus: Succinate accumulation and tumour suppression. J Cell Mol Med 2020;24:1179-88. [Crossref] [PubMed]
- Toader MP, Taranu T, Constantin MM, et al. High serum level of interleukin-6 is linked with dyslipidemia in oral lichen planus. Exp Ther Med 2021;22:987. [Crossref] [PubMed]
- Blank A, Schmitt AM, Korpershoek E, et al. SDHB loss predicts malignancy in pheochromocytomas/sympathethic paragangliomas, but not through hypoxia signalling. Endocr Relat Cancer 2010;17:919-28. [Crossref] [PubMed]
- Ge X, Wang L, Li M, et al. Vitamin D/VDR signaling inhibits LPS-induced IFNγ and IL-1β in Oral epithelia by regulating hypoxia-inducible factor-1α signaling pathway. Cell Commun Signal 2019;17:18. [Crossref] [PubMed]
- Selak MA, Armour SM, MacKenzie ED, et al. Succinate links TCA cycle dysfunction to oncogenesis by inhibiting HIF-alpha prolyl hydroxylase. Cancer Cell 2005;7:77-85. [Crossref] [PubMed]
- Liu Z, Apontes P, Fomenko EV, et al. Mangiferin Accelerates Glycolysis and Enhances Mitochondrial Bioenergetics. Int J Mol Sci 2018;19:201. [Crossref] [PubMed]
- Liu S, Xiao Z, Ai F, et al. miR-142-5p promotes development of colorectal cancer through targeting SDHB and facilitating generation of aerobic glycolysis. Biomed Pharmacother 2017;92:1119-27. [Crossref] [PubMed]
- Fang Z, Sun Q, Yang H, et al. SDHB Suppresses the Tumorigenesis and Development of ccRCC by Inhibiting Glycolysis. Front Oncol 2021;11:639408. [Crossref] [PubMed]
- Wortley C. Warburg: father of the metabolic approach to cancer. Lancet Gastroenterol Hepatol 2022;7:911. [Crossref] [PubMed]
- Jones R, McDonald KE, Willson JA, et al. Mutations in succinate dehydrogenase B (SDHB) enhance neutrophil survival independent of HIF-1α expression. Blood 2016;127:2641-4. [Crossref] [PubMed]
- Qin C, Yang G, Yang J, et al. Metabolism of pancreatic cancer: paving the way to better anticancer strategies. Mol Cancer 2020;19:50. [Crossref] [PubMed]
- Abdel-Wahab AF, Mahmoud W, Al-Harizy RM. Targeting glucose metabolism to suppress cancer progression: prospective of anti-glycolytic cancer therapy. Pharmacol Res 2019;150:104511. [Crossref] [PubMed]
(English Language Editor: J. Jones)