Idebenone reduces sepsis-induced oxidative stress and apoptosis in hepatocytes via RAGE/p38 signaling
Highlight box
Key findings
• IDE has great potential to be a drug treatment for the improvement of sepsis-induced liver injury.
What is known and what is new?
• IDE alleviated LPS-induced hepatocyte activity damage and apoptosis via RAGE/p38 signaling.
• IDE alleviated LPS-induced oxidative stress and inflammatory release in hepatocytes via RAGE/p38 signaling.
What is the implication, and what should change now?
• IDE might be an effective drug for the treatment of sepsis-induced liver injury.
Introduction
Sepsis is a major contributor to the death of hospitalized patients in the intensive care unit (ICU) (1). Sepsis diagnosis can be especially difficult due to the multiple comorbidities and underlying diseases of patients (2). As a syndrome that is characterized by dysregulated responses of the host to invading pathogens, sepsis can induce a variety of organ dysfunctions (3). In the process of systemic infections, the liver regulates the body’s immune defense by clearing the bacteria and producing acute phase proteins (APP) and cytokines (4). Compared with the incidence of failure of other organs, the incidence of sepsis-induced liver failure is lower due to its high regenerative capacity and ability to withstand damage (5). Nevertheless, liver dysfunction and failure are closely related to serious complications in sepsis. Fortunately, many drugs were found to be effective for the improvement of sepsis-induced liver injury. For example, metformin was testified to be effective in the alleviation of sepsis-related liver injury (6). Additionally, Hwang and co-workers have testified that glucosamine improves survival of sepsis mice (7). Nevertheless, the efficacy of idebenone (IDE) on sepsis-related liver injury remains unknown.
As a synthetic analog of coenzyme Q10 (CoQ10), IDE acts as a critical antioxidant for the cell membrane and an elementary constituent of the adenosine triphosphate (ATP)-producing mitochondrial electron transport chain (8). A typical characteristic of IDE is its antioxidant capacity (9). The clinical safety of IDE is well-dpcumented and it has been currently used for the treatment of Freidrich’s ataxia and Alzheimer’s disease due to its high antioxidant capacity (10). To our knowledge, the functional role of IDE in numerous diseases has been widely discussed. For instance, IDE has been shown to help protect against rotenone-induced Parkinson’s disease (10). Qian et al. proposed that IDE could attenuate oxidative stress and neuroinflammation in rats with vascular dementia, which indicated the therapeutic potential of IDE for vascular dementia (11). Interestingly, IDE has also been found to protect against sepsis-induced lung damage (12). Nevertheless, whether IDE can alleviate sepsis-induced liver injury remains unknown.
The receptor for advanced glycation end products (RAGE), which belongs to the immunoglobulin superfamily, acts as a critical player under physiological and pathological conditions (13). Moreover, it is believed that RAGE is not only an inflammatory intermediary but also a key inducer of oxidative stress (14). It was reported previously that RAGE regulation is important for the improvement of sepsis-associated lung injury. For instance, RAGE inhibition pathways have been demonstrated to relieve inflammation in sepsis-induced acute lung liver (15). In addition, Zhang et al. reported that RAGE depletion promoted the release of inflammatory cytokines in sepsis-induced acute lung injury (16). Interestingly, the RAGE pathway also participates in the progression of sepsis-induced liver injury (17). Additionally, a previous study illustrated that IDE could repress RAGE pathway expression (18).
The present study was designed to investigate the efficacy of IDE on the inflammation and oxidative stress in sepsis-induced liver injury and reveal its hidden reaction mechanism. We present the following article in accordance with the ARRIVE reporting checklist (available at https://atm.amegroups.com/article/view/10.21037/atm-22-5758/rc).
Methods
Rat model of sepsis-induced liver injury
Male Sprague-Dawley rats (230±20 g, 8-week-old) provided by the Laboratory Animal Center of China were housed in temperature-controlled cages with 12 h light/dark cycle for 2 weeks. All rats were fed a standard pellet diet and water ad libitum. Varying concentrations of IDE were applied for the administration of rats (12). All rats were randomly separated into five groups (n=5): control group, sepsis group, sepsis + IDE 50 mg/kg group, sepsis + IDE 100 mg/kg group, and sepsis + IDE 200 mg/kg group. Before the cecal ligation and puncture (CLP) operation, the rats were anesthetized with 1% pentobarbital sodium (30 mg/kg body weight). Subsequently, a 2-cm incision was made on the abdominal wall and the cecum was exposed and ligated 0.5 cm from the tip with a 4-0 silk suture. The distal cecum was punctured using a 22-gauge needle to extrude a small quantity of fecal contents. After returning the cecum to the peritoneal cavity, 4-0 silk sutures were applied to close the two layers of the exposed abdominal wall. Next, 1 mL of normal saline was employed to resuscitate the rats. After 24 h, 10 rats were euthanized and the collected blood was centrifuged at 3,000 rpm for 15 min at 4 ℃ to obtain the serum samples. The harvested live tissues were immediately stored at −80 ℃.
A protocol was prepared before the study without registration. The animal experiments was conducted in Zhaofenghua Biotechnology (Nanjing) Co., Ltd. Animal experiments were performed under a project license (No. IACUC-20210408-01) granted by the Ethics Committee of Zhaofenghua Biotechnology (Nanjing) Co., Ltd., in compliance with the Zhaofenghua Biotechnology (Nanjing) Co., Ltd. guidelines for the care and use of animals.
Serum preparation
A non-heparinized capillary tube was applied to extract blood from the medial epicanthus of the rats’ eyes. Blood centrifugation was conducted at 1,000 ×g for 30 min in a cooling centrifuge, followed by collection in Eppendorf tubes. The blood, which was stored at −20 ℃, was utilized to measure the serum alanine aminotransferase (ALT), aspartate aminotransferase (AST), tumor necrosis factor-α (TNF-α), interleukin-1β (IL-1β), and interleukin-6 (IL-6).
Liver tissue preparation
After euthanasia, the collected livers were separated into three portions. A 10% liver homogenate in normal saline was prepared using a homogenizer. The first portion was then centrifuged at 1,000 ×g for 15 min at 4 ℃. The collected supernatant was applied to estimate the levels of TNF-α, IL-1β, IL-6, malondialdehyde (MDA), superoxide dismutase (SOD), and glutathione peroxidase (GSH-Px). To assess Bcl-2, Bax, and cleaved caspase3, the second portion was stored at −80 ℃. For histopathological examination, the third portion was maintained in a 10% formalin solution in normal saline.
Cell culture
Primary hepatocytes provided by Geneline Bioscience (Shanghai, China) were cultivated in Dulbecco’s modified Eagle medium (DMEM) supplemented with 10% fetal bovine serum (FBS, Guangzhou Perseco Biotechnology Co., Ltd., Guangzhou, China) and 1% antibiotics at 37 ℃ in the presence of 5% CO2. A sepsis-induced liver injury model was established by administering primary hepatocytes with 0.5 µg/mL lipopolysaccharide (LPS) for 24 h.
Cell transfection
Plasmids carrying the RAGE gene [overexpression (Ov)-RAGE] and its corresponding negative control (Ov-NC) were constructed by GenePharma (Shanghai, China). The above-mentioned plasmids were transfected into primary hepatocytes using Lipofectamine 2000 (Invitrogen, CA, USA) for 24 h. After 24 h, the transfection efficacy was tested utilizing real-time quantitative polymerase chain reaction (RT-qPCR) and western blot.
Hematoxylin-eosin (HE) staining
The histopathological examination of the liver sections was carried out according to the method described in a previous study (19). Briefly, the liver tissues were fixed with formalin, embedded in paraffin, and then stained using HE. A light microscope (Thermo Fisher Scientific, Inc., Shanghai, China) was used for observation.
Measurement of ALT and AST
The levels of ALT and AST were estimated using ALT and AST assay kits strictly according to the manufacturer’s suggested protocol.
Enzyme-linked immunosorbent assay (ELISA)
To evaluate the release of inflammatory cytokines, the levels of TNF-α, IL-1β, and IL-6 in the serum and liver tissue were appraised using ELISA kits according to the standard recommendations. The optical density was determined at 450 nm and the inflammatory cytokines were calculated using the standard curve.
Measurement of MDA, SOD, and GSH-Px
The contents of MDA, SOD, and GSH-Px in the liver tissues and cell suspensions were measured using MDA, SOD, and GSH-Px assay kits according to the recommended specifications, which were all provided by the Nanjing Jiancheng Bioengineering Institute (China).
Terminal deoxynucleotidyl transferase-mediated nick-end labeling (TUNEL) staining
The effects of IDE on the apoptosis of LPS-induced hepatocytes were evaluated using a TUNEL assay kit (Invitrogen; Thermo Fisher Scientific, Inc., Shanghai, China) strictly according to the manufacturers’ specifications. Briefly, the hepatocytes were subjected to 4% paraformaldehyde embedding and 0.25% Triton-X 100 permeabilization. Subsequently, the phosphate-buffered saline (PBS)-rinsed cells were exposed to a TUNEL reaction solution for 1 h and 4',6-diamidino-2-phenylindole (DAPI) was applied to counterstain the cell nuclei. Lastly, a fluorescent microscope (Olympus Corporation, Beijing, China) was employed to capture the apoptotic cells in five randomly selected fields.
Western blot
Liver tissue was homogenized in lysis buffer and centrifuged at 13,200 rpm for 20 min. Primary hepatocytes were lysed in Nonidet P-40 lysis buffer (Thermo Fisher Scientific, Inc., Shanghai, China). Following separation with 8% sodium dodecyl sulfate-polyacrylamide gel electrophoresis (SDS-PAGE), the proteins were transferred onto polyvinylidene fluoride (PVDF) membranes. Subsequently, the PVDF membranes were blocked using 5% non-fat milk or 5% bovine serum albumin (BSA) and subsequently cultivated with primary antibodies targeting Bcl-2 (ab196495; 1:1,000; Abcam, Shanghai, China), Bax (ab32503; 1:1,000; Abcam), cleaved caspase3 (19677-1-AP; 1:10,000; Proteintech, Wuhan, China), RAGE (ab216329; 1:1,000; Abcam), phosphorylated (p)-p38 (ab195049; 1:1,000; Abcam), p38 (ab170099; 1:1,000; Abcam), or glyceraldehyde-3-phosphate dehydrogenase (GAPDH) (ab181602; 1:10,000; Abcam) overnight at 4 °C. Thereafter, they were subjected to horseradish peroxidase (HRP)-labeled rabbit anti-rat secondary antibody (ab6734; 1:1,000; Abcam) or HRP-labeled rabbit anti-mouse secondary antibody (ab6728; 1:2,000; Abcam) at room temperature for 2 h. Finally, enhanced chemiluminescence (ECL) (Yeasen Biotech, Shanghai, China) and Image J software (version 146, National Institutes of Health, USA) were adopted for the visualization and analysis of the protein bands.
Cell counting kit-8 (CCK-8)
The viability of primary hepatocytes was estimated using CCK-8. Initially, the primary hepatocytes were inoculated into 96-well plates at a density of 5×103 cells/well and then cultivated for 24 h. Next, 10 µL of CCK-8 reagent (Beyotime, China) was added into each well to further cultivate the cells for another 3 h. Lastly, the optical density value was measured at 450 nm using a microplate reader (Shenzhen Ziker Biotechnology Co., Ltd., Shenzhen, China).
RT-qPCR
RNA extracted from the sample cells with Trizol® reagent (Guangzhou Saiyan Biotechnology Co., Ltd., Guangzhou, China) was synthesized into complementary DNA (cDNA) using a commercial RevertAidTM cDNA Synthesis kit (Beijing Think-Far Technology Co., Ltd., Beijing, China). QPCR was performed using the SYBR Premix Ex TaqTM II kit (Thermo Fisher Scientific, Inc.) according to the standard protocol. The following requirements for PCR were applied: initial denaturation at 95 ℃ for 8 min; denaturation at 95 ℃ for 25 sec; annealing at 60 ℃ for 30 sec; extension at 72 ℃ for 30 sec; and final extension at 72 ℃ for 10 min. A comparative cycle threshold (Ct) method was applied to assess the relative gene expressions. The primer sequences were as follows: RAGE forward primer: 5'-CCTCCCCAATGGTTCACTCC-3', reverse primer: 5'-AGCGACTGTTCCACCTTCAG-3', or GAPDH forward primer: 5'-TGTGGGCATCAATGGATTTGG-3', reverse primer: 5'-ACACCATGTATTCCGGGTCAAT-3'.
Reactive oxygen species (ROS) measurement
Primary hepatocytes were injected into a 96-well plate and then cultivated with dichloro-dihydro-fluorescein diacetate (DCFH-DA) solution (1:1,000). The ROS content was decided at wavelengths of 488 and 525 nm using a fluorescence microplate reader. The control group served as the baseline absorbance value to demonstrate the average fluorescence intensity of each group.
Statistical analysis
All collected data were presented as the mean ± standard deviation and analyzed using GraphPad Prism software (version 8.0; GraphPad Software, Inc., USA). One-way analysis of variance (ANOVA) with Tukey’s post hoc test was employed to compare multiple groups. A P value less than 0.05 indicated that the experimental data were of statistical significance.
Results
IDE improved liver function and alleviated sepsis-induced pathological damage
Compared to the control group, the liver pathological score was markedly elevated after sepsis induction (Figure 1A). However, this was reduced by IDE treatment in a concentration-dependent manner, indicating that IDE could alleviate liver pathological damage. Also, in contrast to the control group, sepsis stimulation elevated the expressions of ALT and AST, while IDE treatment exerted the opposite effect on them, as evidenced by the decreased levels of ALT and AST in sepsis-induced rats with IDE administration (50, 100, and 200 mg/kg). This result implied that IDE administration could improve liver function following sepsis-induced damage (Figure 1B,1C).
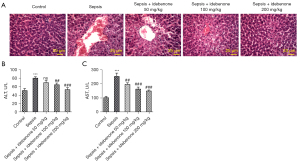
IDE inhibited the levels of inflammatory cytokines in the serum and liver tissues of sepsis-induced rats
As shown in Figure 2A, the levels of TNF-α, IL-1β, and IL-6 in the serum were significantly increased by sepsis induction compared to those in the control group. However, in contrast to the sepsis group, the levels of these inflammatory cytokines were reduced by IDE administration in a concentration-dependent manner. Similarly, compared to the sepsis group, the increased levels of TNF-α, IL-1β, and IL-6 in sepsis-induced liver tissues were also diminished following the administration of IDE (Figure 2B). The above results showed that IDE treatment exerted a suppressive effect on the inflammatory response in sepsis-induced rats.
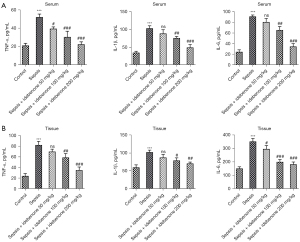
IDE inhibited the level of oxidative stress in liver tissues of sepsis-induced rats
Compared to the control group, sepsis induction remarkably elevated MDA activity but inhibited the activities of SOD and GSH-Px in the liver tissues. However, compared to the sepsis group, IDE treatment decreased the activity of MDA and partially enhanced the activities of SOD and GSH-Px in a concentration-dependent manner (Figure 3A-3C). Collectively, these results demonstrate that IDE repressed the oxidative stress in liver tissues of sepsis-induced rats.
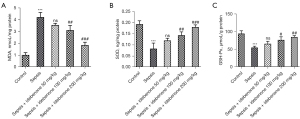
IDE inhibited the apoptosis of liver tissue cells in sepsis-induced rats
Compared to the sepsis group, the sepsis-induced promotion of apoptosis of liver tissue cells was diminished after IDE administration (Figure 4A). In addition, sepsis induction decreased Bcl-2 expression but dramatically increased the contents of Bax and cleaved caspase 3 compared to those in the control group, which were subsequently reversed by IDE treatment, highlighting the repressive effects of IDE on apoptosis in sepsis-induced rats (Figure 4B,4C).
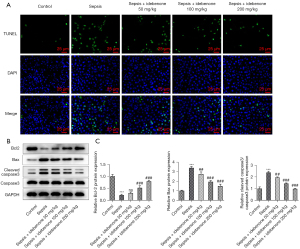
IDE suppressed the expressions of RAGE/p38 signaling
As demonstrated in Figure 5A,5B, the protein contents of RAGE and p-p38 in LPS-induced hepatocytes were markedly elevated by sepsis stimulation compared with the control group. In contrast to the sepsis group, IDE administration lowered the contents of RAGE and p-p38 in a concentration-dependent manner, illustrating that IDE treatment could inhibit RAGE/p38 signaling in hepatocytes with LPS induction.
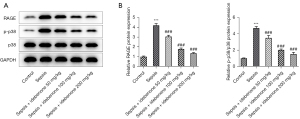
IDE alleviated LPS-induced hepatocyte activity damage and suppressed the expressions of RAGE/p38 signaling
The CCK-8 results demonstrated that IDE treatment had no significant impacts on the viability of hepatocytes (Figure 6A). Compared to the control group, LPS induction remarkably reduced the viability of hepatocytes, while IDE administration partially revived the cell viability in contrast to that in the LPS group (Figure 6B). Moreover, the enhanced expressions of RAGE and p-p38 in hepatocytes caused by LPS stimulation were decreased by IDE administration (Figure 6C,6D). It is worth noting that the viability in the LPS + IDE 5 µM group was higher than those in the LPS + IDE 1 µM group and LPS + IDE 2.5 µM group. Therefore, an IDE concentration of 5 µM was applied for the subsequent experiments.
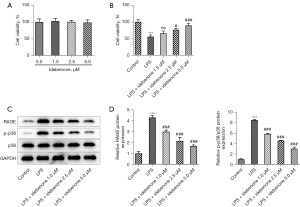
IDE alleviated LPS-induced hepatocyte activity damage and apoptosis via RAGE/p38 signaling
In contrast to Ov-NC, the messenger RNA (mRNA) and protein expressions of RAGE were significantly elevated following cell transfection with Ov-RAGE (Figure 7A,7B). We found that the reduced cell viability in LPS-induced hepatocytes was partially revived after IDE treatment (Figure 7C). Nevertheless, RAGE overexpression reversed the cell viability-promoting effects of IDE, as evidenced by the decreased cell viability in LPS + IDE 5 µM + Ov-RAGE group in contrast with the LPS + IDE 5 µM + Ov-NC group.
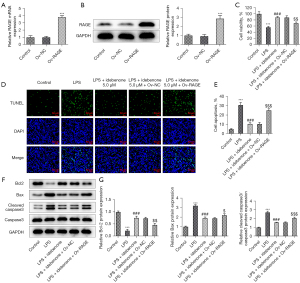
Additionally, IDE administration diminished the apoptosis of LPS-induced hepatocytes compared to the sepsis group, which was then countervailed following RAGE overexpression (Figure 7D,7E). Moreover, IDE treatment elevated Bcl-2 content and diminished the contents of Bax and cleaved caspase3 in LPS-stimulated hepatocytes compared with those in the LPS group, while RAGE overexpression exerted the opposite effects on these proteins, as evidenced by the lower Bcl-2 content as well as the elevated contents of Bax and cleaved caspase3 in the LPS + IDE 5 µM + Ov-RAGE group (Figure 7F,7G). The above results demonstrated that IDE alleviated LPS-induced hepatocyte activity damage and apoptosis via RAGE/p38 signaling.
IDE alleviated LPS-induced oxidative stress and inflammatory release in hepatocytes via RAGE/p38 signaling
As shown in Figure 8A-8C, LPS induction increased MDA activity but decreased the activities of SOD and GSH-Px compared to the control group, which were then reversed by IDE treatment. Also, compared to the LPS + IDE 5 µM + Ov-NC group, RAGE overexpression markedly elevated MDA activity and reduced the activities of SOD and GSH-Px in LPS-induced hepatocytes with IDE treatment. Additionally, IDE treatment lowered the content of ROS relative to the LPS group, which was subsequently reversed following RAGE overexpression (Figure 8D). Furthermore, IDE treatment markedly decreased the levels of TNF-α, IL-1β, and IL-6 in LPS-induced hepatocytes compared to the LPS group. However, RAGE overexpression exerted the opposite effects on these proteins, as illustrated by the significantly elevated contents of TNF-α, IL-1β, and IL-6 in the LPS + IDE 5 µM + Ov-RAGE group compared to those in the LPS + IDE 5 µM + Ov-NC group (Figure 8E). In summary, IDE alleviated LPS-induced oxidative stress and inflammatory release in hepatocytes via RAGE/p38 signaling.
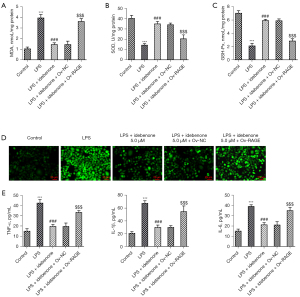
Discussion
Previous studies have reported that sepsis could contribute to serious liver injury (20). Normal liver function is key to the survival of sepsis patients (21). It is well known that the dysregulation of inflammatory cytokines and liver damage resulting from oxidative stress can lead to a poor prognosis in sepsis patients (22,23). As is known to all, inflammation, oxidative stress and apoptosis were involved in the development of sepsis-induced liver injury, the intervention of which might help to fight against it (21,24). In recent years, great progresses have been achieved in the exploration of effective drugs for sepsis-induced liver injury and the potential of targeted treatments has been highlighted (25,26).
IDE is an essential component of the mitochondrial electron transport chain, which produces ATP (27). Elevated expressions of AST and ALT in the serum are considered to indicate hepatic damage (28). A previous study reported that IDE treatment could decrease the expressions of AST and ALT, thereby improving liver function (29). In the present study, we discovered that the increased levels of AST and ALT resulting from sepsis induction were lowered after IDE treatment. Moreover, the histopathological examination results of the liver also showed that IDE treatment alleviated liver pathological damage.
Sepsis is characterized by systemic inflammation (30). Circulating proinflammatory mediators, including TNF-α, IL-1β, and IL-6 are elevated and are considered to be important biomarkers for the diagnosis and prognosis of sepsis patients (31). Thus, the modulation of inflammatory cytokines might be an effective therapy for the improvement of sepsis-induced liver injury. IDE, which possesses an anti-inflammatory property, has been reported to regulate inflammation in multiple diseases (32). Blanco et al. reported that IDE exerted suppressive effects on inflammatory damage in lupus (33). In addition, IDE protects against spontaneous chronic murine colitis by relieving inflammation (34). In the present study, it was discovered that the elevated expressions of TNF-α, IL-1β, and IL-6 in the serum and liver tissues of sepsis-induced rats were lowered following IDE administration. In the pathogenesis of sepsis, LPS, which is a potent activator, can be utilized to trigger an inflammatory response (31). Herein, it was found that LPS induction significantly elevated the levels of TNF-α, IL-1β, and IL-6 in hepatocytes, which were subsequently diminished by IDE treatment.
Redox reactions represent the basis for numerous biochemical mechanisms imperative for physiological cell function (35,36). Oxidants and antioxidants are critical players in the abovementioned mechanisms (37). It is known that oxidative stress is a critical factor in the pathogenesis of sepsis, in which the excessive production of ROS (due to CLP) can cause macrophage activation, contributing to further inflammation and damage (38). A previous study demonstrated that the GSH-Px enzyme plays a pivotal role in safeguarding organisms from oxidative damage (39). Additionally, MDA has been shown to indicate lipid peroxidation as well as tissue damage (19). Interestingly, IDE can regulate oxidative stress in many diseases. For instance, IDE inhibited the oxidative stress in retinal pigment epithelium cells in age-related macular degeneration (AMD) (40). Also, Qian et al. showed that IDE treatment increases MDA activity and reduces SOD activity (11). In the present study, it was found that sepsis induction elevated MDA activity but decreased the activities of SOD and GSH-Px, which were then reversed by IDE administration. Similarly, the enhanced MDA activity as well as the diminished activities of SOD and GSH-Px in LPS-stimulated hepatocytes were also countervailed after IDE treatment.
Deregulated cell apoptosis is one of the mechanisms involved in sepsis-induced liver injury (41). It has been reported that apoptosis is vital in the progression of organ damage after sepsis (42), and previous studies have demonstrated that the inhibition of apoptosis could improve outcomes in experimental models of severe sepsis (43,44). Therefore, the regulation of apoptosis could also be a method of alleviating sepsis-induced liver injury. Guan et al. proposed that IDE maintained the survival of mutant myocilin cells through the suppression of apoptosis (45). In the present study, the sepsis-induced promotion of apoptosis in liver tissue cells was inhibited by IDE treatment in a concentration-dependent manner. It is likely that the enhanced apoptosis in LPS-induced hepatocytes was also reduced after IDE treatment. Bcl-2, Bax, and cleaved caspase3 are markers of apoptosis (46). The western blot results demonstrated that IDE treatment elevated the Bcl-2 content and reduced the contents of Bax and cleaved caspase3 in both sepsis-induced and LPS-induced hepatocytes.
Zhang et al. found that the RAGE pathway was associated with sepsis-associated encephalopathy (47). Another previous study also verified that the suppression of RAGE could improve survival in experimental models of severe sepsis (48). Importantly, Bopp et al. posited that RAGE might be a therapeutic target in sepsis (49). Also, Sun et al. demonstrated that IDE could inhibit the expression of RAGE signaling (18). Meanwhile, RAGE suppression could reduce the expression of p38 signaling (50). In this study, the elevated expressions of RAGE and p-p38 due to sepsis induction were decreased by IDE treatment in a concentration-dependent manner, indicating that IDE could block RAGE/p38 signaling. To further investigate the mechanism of IDE and RAGE/p38 signaling in sepsis-induced liver injury, the above functional experiments were conducted again and the results revealed that the suppressive effects of IDE treatment on cell viability damage, apoptosis, oxidative stress, and inflammation in LPS-induced hepatocytes were countervailed following RAGE overexpression. This implied that IDE protected against oxidative stress, apoptosis, and inflammation in sepsis-induced liver injury via the regulation of RAGE/p38 signaling.
Conclusions
In conclusion, this study demonstrated the efficacy of IDE in sepsis-induced liver injury both in vivo and in vitro and illustrated that IDE protected against sepsis-induced oxidative stress, apoptosis, and inflammation via the suppression of RAGE/p38 signaling. This is the first study to identify the potential of IDE as a drug treatment for the improvement of sepsis-induced liver injury.
Limitations
Although this study was the first to explore the efficacy of IDE in sepsis-induced liver injury, there were some limitations. For example, the side effects of IDE on patients were not investigated in the present study. Therefore, further studies are needed in the future.
Acknowledgments
Funding: None.
Footnote
Reporting Checklist: The authors have completed the ARRIVE reporting checklist. Available at https://atm.amegroups.com/article/view/10.21037/atm-22-5758/rc
Data Sharing Statement: Available at https://atm.amegroups.com/article/view/10.21037/atm-22-5758/dss
Conflicts of Interest: All authors have completed the ICMJE uniform disclosure form (available at https://atm.amegroups.com/article/view/10.21037/atm-22-5758/coif). The authors have no conflicts of interest to declare.
Ethical Statement: The authors are accountable for all aspects of the work in ensuring that questions related to the accuracy or integrity of any part of the work are appropriately investigated and resolved. Animal experiments were performed under a project license (No. IACUC-20210408-01) granted by the Ethics Committee of Zhaofenghua Biotechnology (Nanjing) Co., Ltd., in compliance with the Zhaofenghua Biotechnology (Nanjing) Co., Ltd. guidelines for the care and use of animals.
Open Access Statement: This is an Open Access article distributed in accordance with the Creative Commons Attribution-NonCommercial-NoDerivs 4.0 International License (CC BY-NC-ND 4.0), which permits the non-commercial replication and distribution of the article with the strict proviso that no changes or edits are made and the original work is properly cited (including links to both the formal publication through the relevant DOI and the license). See: https://creativecommons.org/licenses/by-nc-nd/4.0/.
References
- Rello J, Valenzuela-Sánchez F, Ruiz-Rodriguez M, et al. Sepsis: A Review of Advances in Management. Adv Ther 2017;34:2393-411. [Crossref] [PubMed]
- Novosad SA, Sapiano MR, Grigg C, et al. Vital Signs: Epidemiology of Sepsis: Prevalence of Health Care Factors and Opportunities for Prevention. MMWR Morb Mortal Wkly Rep 2016;65:864-9. [Crossref] [PubMed]
- Fleischmann C, Scherag A, Adhikari NK, et al. Assessment of Global Incidence and Mortality of Hospital-treated Sepsis. Current Estimates and Limitations. Am J Respir Crit Care Med 2016;193:259-72. [Crossref] [PubMed]
- Sun J, Zhang J, Wang X, et al. Gut-liver crosstalk in sepsis-induced liver injury. Crit Care 2020;24:614. [Crossref] [PubMed]
- Weiss YG, Bellin L, Kim PK, et al. Compensatory hepatic regeneration after mild, but not fulminant, intraperitoneal sepsis in rats. Am J Physiol Gastrointest Liver Physiol 2001;280:G968-73. [Crossref] [PubMed]
- Liang H, Song H, Zhang X, et al. Metformin attenuated sepsis-related liver injury by modulating gut microbiota. Emerg Microbes Infect 2022;11:815-28. [Crossref] [PubMed]
- Hwang JS, Kim KH, Park J, et al. Glucosamine improves survival in a mouse model of sepsis and attenuates sepsis-induced lung injury and inflammation. J Biol Chem 2019;294:608-22. [Crossref] [PubMed]
- Gueven N, Ravishankar P, Eri R, et al. Idebenone: When an antioxidant is not an antioxidant. Redox Biol 2021;38:101812. [Crossref] [PubMed]
- Jaber S, Polster BM. Idebenone and neuroprotection: antioxidant, pro-oxidant, or electron carrier? J Bioenerg Biomembr 2015;47:111-8. [Crossref] [PubMed]
- Avcı B, Günaydın C, Güvenç T, et al. Idebenone Ameliorates Rotenone-Induced Parkinson's Disease in Rats Through Decreasing Lipid Peroxidation. Neurochem Res 2021;46:513-22. [Crossref] [PubMed]
- Qian X, Xu Q, Li G, et al. Therapeutic Effect of Idebenone on Rats with Vascular Dementia via the MicroRNA-216a/RSK2/NF-κB Axis. Neuropsychiatr Dis Treat 2021;17:533-43. [Crossref] [PubMed]
- Akpinar E, Kutlu Z, Kose D, et al. Protective Effects of Idebenone against Sepsis Induced Acute Lung Damage. J Invest Surg 2022;35:560-8. [Crossref] [PubMed]
- Cai Z, Liu N, Wang C, et al. Role of RAGE in Alzheimer's Disease. Cell Mol Neurobiol 2016;36:483-95. [Crossref] [PubMed]
- Guan L, Mao Z, Yang S, et al. Dioscin alleviates Alzheimer's disease through regulating RAGE/NOX4 mediated oxidative stress and inflammation. Biomed Pharmacother 2022;152:113248. [Crossref] [PubMed]
- Li K, Yang J, Han X. Ketamine attenuates sepsis-induced acute lung injury via regulation of HMGB1-RAGE pathways. Int Immunopharmacol 2016;34:114-28. [Crossref] [PubMed]
- Zhang Z, Zhou J, Liao C, et al. RAGE deficiency attenuates the protective effect of Lidocaine against sepsis-induced acute lung injury. Inflammation 2017;40:601-11. [Crossref] [PubMed]
- Weinhage T, Wirth T, Schütz P, et al. The Receptor for Advanced Glycation Endproducts (RAGE) Contributes to Severe Inflammatory Liver Injury in Mice. Front Immunol 2020;11:1157. [Crossref] [PubMed]
- Sun YN, Liu LB, Xue YX, et al. Effects of insulin combined with idebenone on blood-brain barrier permeability in diabetic rats. J Neurosci Res 2015;93:666-77. [Crossref] [PubMed]
- Aboyoussef AM, Mohammad MK, Abo-Saif AA, et al. Granisetron attenuates liver injury and inflammation in a rat model of cecal ligation and puncture-induced sepsis. J Pharmacol Sci 2021;147:358-66. [Crossref] [PubMed]
- Yan J, Li S, Li S. The role of the liver in sepsis. Int Rev Immunol 2014;33:498-510. [Crossref] [PubMed]
- Wu Y, Zhao M, Lin Z. Pyrroloquinoline quinone (PQQ) alleviated sepsis-induced acute liver injury, inflammation, oxidative stress and cell apoptosis by downregulating CUL3 expression. Bioengineered 2021;12:2459-68. [Crossref] [PubMed]
- Shen C, Li J. LncRNA XIST silencing protects against sepsis-induced acute liver injury via inhibition of BRD4 expression. Inflammation 2021;44:194-205. [Crossref] [PubMed]
- van der Poll T, van de Veerdonk FL, Scicluna BP, et al. The immunopathology of sepsis and potential therapeutic targets. Nat Rev Immunol 2017;17:407-20. [Crossref] [PubMed]
- Malkoç M, Patan H, Yaman SÖ, et al. l-theanine alleviates liver and kidney dysfunction in septic rats induced by cecal ligation and puncture. Life Sci 2020;249:117502. [Crossref] [PubMed]
- Hong MK, Hu LL, Zhang YX, et al. 6-Gingerol ameliorates sepsis-induced liver injury through the Nrf2 pathway. Int Immunopharmacol 2020;80:106196. [Crossref] [PubMed]
- Savio LEB, de Andrade Mello P, Figliuolo VR, et al. CD39 limits P2X7 receptor inflammatory signaling and attenuates sepsis-induced liver injury. J Hepatol 2017;67:716-26. [Crossref] [PubMed]
- Muscoli C, Fresta M, Cardile V, et al. Ethanol-induced injury in rat primary cortical astrocytes involves oxidative stress: effect of idebenone. Neurosci Lett 2002;329:21-4. [Crossref] [PubMed]
- Yuan R, Tao X, Liang S, et al. Protective effect of acidic polysaccharide from Schisandra chinensis on acute ethanol-induced liver injury through reducing CYP2E1-dependent oxidative stress. Biomed Pharmacother 2018;99:537-42. [Crossref] [PubMed]
- Abdelazim SA, Darwish HA, Ali SA, et al. Potential antifibrotic and angiostatic impact of idebenone, carnosine and vitamin E in nano-sized titanium dioxide-induced liver injury. Cell Physiol Biochem 2015;35:2402-11. [Crossref] [PubMed]
- Xiong H, Wang H, Yu Q. Circular RNA circ_0003420 mediates inflammation in sepsis-induced liver damage by downregulating neuronal PAS domain protein 4. Immunopharmacol Immunotoxicol 2021;43:271-82. [Crossref] [PubMed]
- Liu A, Wang W, Fang H, et al. Baicalein protects against polymicrobial sepsis-induced liver injury via inhibition of inflammation and apoptosis in mice. Eur J Pharmacol 2015;748:45-53. [Crossref] [PubMed]
- Lauro F, Ilari S, Giancotti LA, et al. Pharmacological effect of a new idebenone formulation in a model of carrageenan-induced inflammatory pain. Pharmacol Res 2016;111:767-73. [Crossref] [PubMed]
- Blanco LP, Pedersen HL, Wang X, et al. Improved Mitochondrial Metabolism and Reduced Inflammation Following Attenuation of Murine Lupus With Coenzyme Q10 Analog Idebenone. Arthritis Rheumatol 2020;72:454-64. [Crossref] [PubMed]
- Shastri S, Shinde T, Perera AP, et al. Idebenone Protects against Spontaneous Chronic Murine Colitis by Alleviating Endoplasmic Reticulum Stress and Inflammatory Response. Biomedicines 2020;8:384. [Crossref] [PubMed]
- Jones DP. Radical-free biology of oxidative stress. Am J Physiol Cell Physiol 2008;295:C849-68. [Crossref] [PubMed]
- Zhang J, Wang X, Vikash V, et al. ROS and ROS-Mediated Cellular Signaling. Oxid Med Cell Longev 2016;2016:4350965. [Crossref] [PubMed]
- Mantzarlis K, Tsolaki V, Zakynthinos E. Role of Oxidative Stress and Mitochondrial Dysfunction in Sepsis and Potential Therapies. Oxid Med Cell Longev 2017;2017:5985209. [Crossref] [PubMed]
- Crimi E, Sica V, Slutsky AS, et al. Role of oxidative stress in experimental sepsis and multisystem organ dysfunction. Free Radic Res 2006;40:665-72. [Crossref] [PubMed]
- Samarghandian S, Azimi-Nezhad M, Farkhondeh T, et al. Anti-oxidative effects of curcumin on immobilization-induced oxidative stress in rat brain, liver and kidney. Biomed Pharmacother 2017;87:223-9. [Crossref] [PubMed]
- Arend N, Wertheimer C, Laubichler P, et al. Idebenone Prevents Oxidative Stress, Cell Death and Senescence of Retinal Pigment Epithelium Cells by Stabilizing BAX/Bcl-2 Ratio. Ophthalmologica 2015;234:73-82. [Crossref] [PubMed]
- Oberholzer C, Oberholzer A, Clare-Salzler M, et al. Apoptosis in sepsis: a new target for therapeutic exploration. FASEB J 2001;15:879-92. [Crossref] [PubMed]
- Ning M, Liu Y, Wang D, et al. Knockdown of TRIM27 alleviated sepsis-induced inflammation, apoptosis, and oxidative stress via suppressing ubiquitination of PPARγ and reducing NOX4 expression. Inflamm Res 2022;71:1315-25. [Crossref] [PubMed]
- Zhang H, Wang W, Fang H, et al. GSK-3β inhibition attenuates CLP-induced liver injury by reducing inflammation and hepatic cell apoptosis. Mediators Inflamm 2014;2014:629507. [Crossref] [PubMed]
- Li Z, Jia Y, Feng Y, et al. Methane alleviates sepsis-induced injury by inhibiting pyroptosis and apoptosis: in vivo and in vitro experiments. Aging (Albany NY) 2019;11:1226-39. [Crossref] [PubMed]
- Guan Y, Li J, Zhan T, et al. Idebenone Maintains Survival of Mutant Myocilin Cells by Inhibiting Apoptosis. Chin Med J (Engl) 2016;129:2001-4. [Crossref] [PubMed]
- Li P, Wang Y, Liu X, et al. Atypical antipsychotics induce human osteoblasts apoptosis via Wnt/β-catenin signaling. BMC Pharmacol Toxicol 2019;20:10. [Crossref] [PubMed]
- Zhang L, Jiang Y, Deng S, et al. S100B/RAGE/Ceramide signaling pathway is involved in sepsis-associated encephalopathy. Life Sci 2021;277:119490. [Crossref] [PubMed]
- Lutterloh EC, Opal SM, Pittman DD, et al. Inhibition of the RAGE products increases survival in experimental models of severe sepsis and systemic infection. Crit Care 2007;11:R122. [Crossref] [PubMed]
- Bopp C, Bierhaus A, Hofer S, et al. Bench-to-bedside review: The inflammation-perpetuating pattern-recognition receptor RAGE as a therapeutic target in sepsis. Crit Care 2008;12:201. [Crossref] [PubMed]
- Wang X, Sun X, Niu M, et al. RAGE Silencing Ameliorates Neuroinflammation by Inhibition of p38-NF-κB Signaling Pathway in Mouse Model of Parkinson's Disease. Front Neurosci 2020;14:353. [Crossref] [PubMed]
(English Language Editor: A. Kassem)