Total deposition of ultrafine particles in the lungs of healthy men and women: experimental and theoretical results
Introduction
In the past decades ultrafine particles (UFP), representing the particulate fraction <0.10 µm in diameter, have increasingly aroused scientific interest because of several reasons: First, they occur in the ambient air in much greater number than particles belonging to coarser fractions (>0.10 µm); second, most particles of the UFP fraction are characterized by highly irregular geometries, so that their surface area and, as a consequence of that, their surface area to mass ratio are remarkably increased with respect to other particulate substances; third, due to their high number and surface area UFP are predestined to act as carriers of hazardous gaseous compounds (1-5).
Once UFP have entered the respiratory system they are subject to specific forces inducing their partial deposition on the epithelial walls of the tracheobronchial tree and the alveoli. Animal studies (1,2,6) as well as epidemiological and histological investigations (3,7) could sufficiently demonstrate that UFP may induce lung injuries and have greater adverse respiratory effects than fine or coarse particles in the urban air. These effects including among other an enhanced tendency of the UFP-targeted lung tissues to undergo inflammatory reactions, a noticeable change of blood coagulability, and, in the worst case, the initiation of malignant transformation are to a high degree the result of the ability of these particles to pass rapidly through any cellular barriers (8-12).
Concerning an exact quantification of UFP deposition in the human respiratory tract of healthy adults, first experimental studies date back to the middle of the 1980s (13-15). Based on the preliminary data provided by these investigations, further research projects dealing with the pulmonary deposition of the ultrafine fraction in subjects suffering from obstructive lung disease (16,17), probands of different ages (18) as well as male and female adults (19,20) were arranged. They all came to the conclusion that lung deposition of UFP is subject to considerable variations among the mentioned groups of probands. Main hypotheses with regard to deposition fluctuations between men and women include intersexual differences in lung morphometry and breathing behavior (4,5,21). Additionally, the mean daily activity profile containing specific working and resting phases is assumed to influence the total dose of UFP entering the respiratory tract and depositing at certain sites.
Since experimental research dealing with controlled inhalation of UFP has to be conducted according to exact protocols and recruitment of test subjects is partly accompanied by high temporal and financial exposure, theoretical investigations of UFP behavior in the airways and alveoli have increasingly become important in the last years (22-27). The hypothetical approach bears several additional advantages with respect to experiments: First, all parameters having a verifiable effect on UFP deposition can be varied arbitrarily, so that a wide spectrum of information is produced; second, behavior of hazardous UFP such as diesel soot or carbon nanotubes can be studied in detail apart from ethical concerns that are indeed highly justified on the experimental field; and third, consequential damages caused by these particles may be investigated more in detail by theoretical means, so that in the case of industrial accidents and related particle-lung interactions effective precautionary measures can be developed.
The study presented here pursues the main goal to provide a detailed theoretical overview of UFP deposition in healthy men and women and its dependence on diverse breathing parameters. In this respect, the contribution has to be regarded as an extension of the results provided by Jaques and Kim (4).
Methods
Experimental setup
For the inhalation experiments performed by Jaques and Kim (4), 11 men and 11 women ranging in age from 20 to 40 years were recruited. After a comprehensive health check the probands’ basic lung function was analyzed by spirometry and body plethysmography. Generation of ultrafine aerosol particles was carried out by condensation of sebacate oil vapor on nonhygroscopic metallic nuclei. The produced aerosols were diluted with filtered air and forwarded to the inhalation system. Number median diameter (NMD) of the generated particles amounted to 0.04, 0.06, 0.08, and 0.10 µm, respectively, whereas their geometric standard deviation (σg) ranged from 1.27 to 1.34. Aerosol concentration administered to the test subjects via the inhalation device was constantly set to ~50,000 particles/cm3.
Test aerosols were inhaled from a collapsible bag with a volume of 20 L. For statistical reasons each proband inspired the aerosol via the oral path for 10 to 20 breaths. The following 6 breathing patterns were distinguished: (I) tidal volume (Vt) of 500 mL at a respiratory flow rate (Q) of 150 mL·s−1; (II) Vt =500 mL and Q =250 mL·s−1; (III) Vt =750 mL and Q =250 mL·s−1; (IV) Vt =750 mL and Q =375 mL·s−1; (V) Vt =1,000 mL and Q =250 mL·s−1; (VI) Vt =1,000 mL and Q =500 mL·s−1. The total number of particles inhaled (Ni) and exhaled (Ne) was calculated breath by breath using a computer system adjusted to the experimental setup. Total lung deposition fraction (TDF) was then computed by application of the simple formula TDF = (Ni – Ne)/Ni.
Theoretical approach to the behavior of UFP in the respiratory tract
Hypothetical predictions of inhalation, transport, and deposition of UFP were based on both the stochastic model of the human lung architecture generated by Koblinger and Hofmann (28) and the random particle transport approach outlined by the same authors (29). Concerning the stochastic lung model necessary variability of airway morphometry within a given airway generation was induced with the help of generation-specific probability density functions of essential geometric parameters (diameter, length, branching angle, gravity angle) and by randomly selecting values from these functions. According to the random walk model each particle inhaled follows its individual path through the tracheobronchial tree. At each airway bifurcation, selection of the further particle path is partly influenced by the volumetric splitting of the air flow, so that higher probability is given for the particle to enter the major daughter tube. Statistical evaluation of these single particle scenarios is enabled by application of the Monte Carlo method, where the trajectories of high numbers of particles (e.g., 10,000) are modeled simultaneously.
For the theoretical simulation of UFP deposition Brownian motion was assumed to represent the main deposition mechanism exerting on the particles. As comprehensively outlined in previous publications (21-25), deposition efficiency of UFP generally correlates with the residence time of particulate substances in single lung structures, or, in other words, the longer a particle requires passing an airway or an alveolus, the higher is its probability to be deposited on the respective epithelial wall. Further parameters influencing the effectiveness of deposition by Brownian motion are particle diameter, size of the structures passed by UFP, and, not less unimportant, temperature (25,26). Although diffusive particle transport plays the most essential role within the size category regarded for this study, also possible deposition caused by particle inertia and gravity were considered (21-28).
Modeling predictions were conducted by assuming monodisperse aerosols with particles adopting those sizes mentioned above. For the sake of simplicity a uniform particle density of 1.0 g·cm−3 was used. Mean functional residual capacity (FRC) of male probands was set to 3,911±892 mL, whereas that of female probands amounted to 3,314±547 mL (4). Respective values of the breathing patterns were directly adopted from the experimental study.
Results
Experimental and theoretical data of total UFP deposition
Comprehensive results obtained from experimental (4) and theoretical work was summarized in Table 1. For the sake of brevity only selected data and possible tendencies will be subject to a more detailed description. Regarding particles measuring 0.04 µm in size TDF analyzed for male subjects ranges from 0.41 to 0.64 (experiment) and from 0.47 to 0.66 (model), whereas TDF obtained for female probands varies between 0.47 and 0.68 (experiment) and between 0.56 and 0.72 (model). As clearly shown by the data, in both sexes, deposition decreases with the respiratory flow rate and, in the case of constant flow rate, exhibits an increase with tidal volume. Particles with a size of 0.06 µm deposit in the male respiratory tract by 33% to 56% (experiment) and by 36% to 57% (model). In the female respiratory tract respective TDF ranges from 37% to 56% (experiment) and from 45% to 62% (model). Breathing-related tendencies of UFP deposition are similar to those found for 0.04 µm particles. Inhalation of particles measuring 0.08 µm in size produces a TDF varying between 0.31 and 0.48 (experiment) and between 0.25 and 0.45 (model) in the case of male probands. For female subjects TDF ranging from 0.30 to 0.46 (experiment) and from 0.33 to 0.52 (model) could be calculated. Breathing-related deposition trends already reported for smaller particles were also found here, but are marked by lower intensities. With regard to particles adopting a diameter of 0.10 µm experimental TDF computed for males commonly ranges from 0.26 to 0.40, whilst theoretical TDF ranges from 0.22 to 0.40. Respective values found for females vary between 0.25 and 0.39 in the case of experiments and between 0.27 and 0.46 in the case of model predictions.
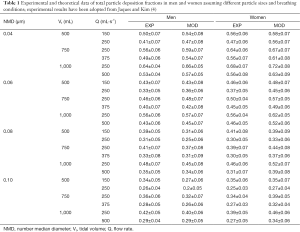
Full table
Advanced modeling predictions
Since the experimental work is exclusively concerned with the presentation and interpretation of total deposition data and their dependence on the selected breathing patterns, further modeling predictions were focused on regional and airway generation-specific deposition behavior of UFP. Hence, for the particle sizes and breathing conditions mentioned above extrathoracic, tracheobronchial, and alveolar deposition fractions were computed. Results of these advanced predictions are summarized in Figures 1-4 and will be only subject to a coarse outline. Regarding UFP measuring 0.04 µm in size extrathoracic deposition fraction ranges from 0.032 to 0.046 in male subjects and from 0.030 to 0.040 in female ones. In both sexes a significant decline of this fraction can be recognized, when the respiratory flow rate is enhanced (Figure 1A). Tracheobronchial deposition fraction predicted for male probands varies between 0.32 and 0.41, whilst that one predicted for females varies between 0.37 and 0.44. Increasing velocity of the inhaled air stream commonly results in a diminution of the particle number colliding with the airway walls (Figure 1B). Alveolar deposition fraction adopts values ranging from 0.10 to 0.21 in men and ranging from 0.14 to 0.25 in women. Here, also a negative correlation between deposited particulate mass and respiratory flow rate may be found (Figure 1C).
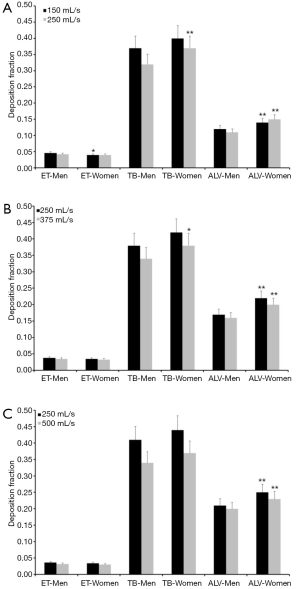
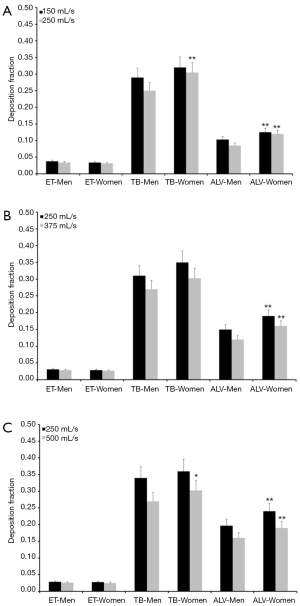
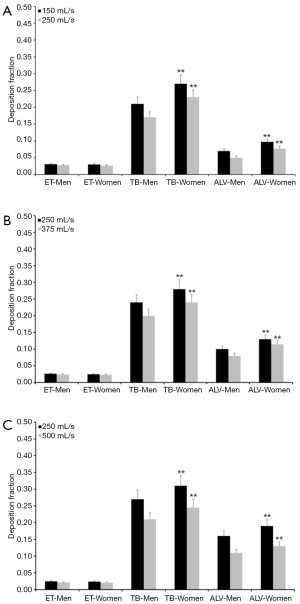
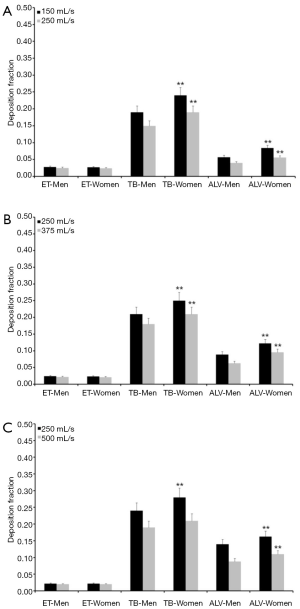
Concerning particles adopting a diameter of 0.06 µm predicted deposition fractions are generally subject to a decrease with respect to 0.04 µm particles, whereas relationships between extent of deposition and breathing conditions are very similar to the case described above. Extrathoracic deposition fraction ranges from 0.026 to 0.038 in male probands and from 0.025 to 0.034 in female ones. Tracheobronchial deposition fraction amounts to 0.25–0.34 in males and to 0.30–0.36 in females, whereas alveolar deposition fraction adopts values ranging from 0.085 to 0.20 (men) and from 0.12 to 0.24 (women; Figure 2). Particles with a diameter of 0.08 µm deposit in the male extrathoracic region by 2.2–3.1% and in the female extrathoracic airways by 2.1–3.0%. Tracheobronchial deposition fraction of these particles ranges from 0.17 to 0.27 (men) and from 0.23 to 0.31 (women), whilst alveolar deposition fraction varies between 0.05 and 0.16 (men) as well as between 0.08 and 0.19 (women; Figure 3). With regard to particles adopting a diameter of 0.10 µm particle deposition fractions predicted for male probands range from 0.020 to 0.028 (extrathoracic), from 0.18 to 0.24 (tracheobronchial), and from 0.04 to 0.14 (alveolar). Respective deposition fractions predicted for female probands range from 0.020 to 0.027 (extrathoracic), from 0.21 to 0.28 (tracheobronchial), and from 0.06 to 0.16 (alveolar; Figure 4).
For a selected breathing pattern (Vt =1,000 mL, Q =250 mL·s−1) deposition of UFP in single airway generations was modeled. By definition, the trachea corresponds to airway generation 0 and the tracheobronchial tree was assumed to contain 26 airway generations. Generation-specific deposition of particles measuring 0.04 µm in size is characterized by differences between male and female subjects insofar as maximum deposition fraction is located in airway generation 19 in men and in airway generation 18 in women. Additionally, deposition fractions predicted for female probands are slightly higher than those predicted for male probands (Figure 5A). Deposition patterns produced for 0.06 µm particles have their maximum values in airway generation 19 (men and women), with deposition fractions adopting values between 0.06 and 0.07 (Figure 5B). Regarding particles with a size of 0.08 µm local particle deposition is already noticeably reduced with respect to smaller UFP and thus ranges from 0.001 to 0.06. Deposition fractions predicted for female subjects exceed those reported for male probands by about 15%. Maximum deposition again occurs in airway generation 19 (men and women; Figure 5C). Particles adopting a diameter of 0.10 µm are generally marked by lowest local deposition fractions. In male probands respective values range from 0.001 to 0.043, whereas in females values varying between 0.001 and 0.051 were calculated. Highest deposition fractions are observable in airway generation 19 (men and women; Figure 5D).
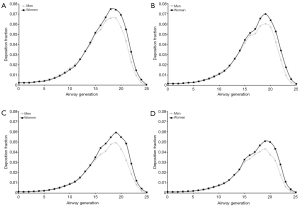
Discussion and conclusions
It is considered a proven fact that inhaled UFPs deposit in rather large amounts in the human respiratory tract. As demonstrated by both experimental and theoretical studies total deposition fractions (TDF) of UFP may vary between 20% and 95% and depend on (I) particle size, with smaller UFP exhibiting a higher tendency for deposition than larger ones; (II) the breathing conditions; and (III) the morphometry of the respiratory system taking up the particulate substances (13-15,21-28,30). In the present contribution experimental results including TDF of UFP with different sizes (0.04, 0.06, 0.08, and 0.10 µm) were compared with respective fractions predicted by a stochastic model for the same size classes. In both the practical and theoretical study males and females were recruited as test subjects in order to investigate intersexual discrepancies in UFP deposition. In addition, breathing conditions were varied by modification of the tidal volume and the respiratory flow rate. As a first fundamental result obtained from experimental and hypothetical work, female probands exhibit TDF exceeding those of male subjects by up to 15%. Only particles measuring 0.10 µm in size are deposited to almost the same extent in female and male lungs. Previous studies have yielded evidence that gender effects on UFP deposition are not forcedly a result of different lung volumes and body sizes (4,13). It is much more assumed that some other factors may play an essential role with regard to the differential total deposition observed between men and women. Morphometric studies could demonstrate that the upper airways are much smaller in females than in males, even if lung volume and body size are constant among the sexes (31,32). By assuming constant respiratory flow rates among male and female subjects, this morphometric particularity occurring in women may have an influence on the velocity of the air stream and therefore may force particle deposition by turbulent diffusion. The used model represents a simplification of this circumstance insofar as all airways are subject to a constant down-scaling in female probands, so that all lung regions are concerned by an increased diffusive effect.
As a general observation an increase of tidal volume causes a partly remarkable rise of UFP deposition, whereas an increase of the respiratory flow rate has a reciprocal effect on deposition (Figure 6). Higher tidal volumes have the effect that more inhaled air has to pass the air-conducting structures to reach the pulmonary gas-exchange zone located in the alveoli. Since the volume of the tracheobronchial system has to be evaluated as rather static (c. 150 mL), more alveolar spheres are subjected to inflation and the inhaled aerosol is distributed to deeper parts of the lungs. Within these structures, being significantly reduced in size with respect to the upper airways of the respiratory tract (18,21,31), Brownian motion is characterized by higher efficiency and particle deposition fractions are increased. Besides the circumstance that any increase of the tidal volume forces inhaled particles to penetrate deeper into the lung, also the time of the particles for residing in deeper lung regions positively correlates with the volume of inspired air (21,25-28). Jaques and Kim (4) have found that, for a given value of the breath-cycle time of 4 s (inhalation time = exhalation time =2 s), the residence time of particles in the alveolar region increases from 2.8 to 3.4 s as tidal volume is enhanced from 500 to 1,000 mL. The combination of longer residence times with short deposition distance commonly results in a higher efficiency of diffusion. By increasing the respiratory flow rate small structures of peripheral airways and alveoli are reached more rapidly but residence times are noticeably decreased again, so that diffusive transport of UFP is practically limited to shorter time spans (Figure 6) (21,29). It has to be noted, however, that extremely fast inhalation and exhalation of aerosols bearing UFP again result in a reduction of the deposition fraction and an increased number of expired particles (22,24).
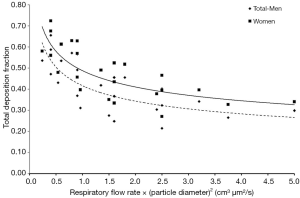
Advanced theoretical computations show that in both sexes the tracheobronchial and alveolar regions represent main targets of UFP, whereas the extrathoracic region is only affected by deposition fractions ranging from 2% to 5%. Whilst low tidal volumes and low flow rates lead to preferred deposition of UFP in the airways, high volumes of inhaled air have the effect of enhanced alveolar deposition. Due to the reasons already mentioned above women are commonly marked by higher deposition fractions than men. Concretely speaking, regional deposition fractions may differ between male and female subjects by up to 10%, so that exposure to ambient aerosols with high concentrations of UFP may have more dramatic health effects on women than on men.
According to previous studies TDF measured in male and female subjects were characterized by a potential difference, with supramicrometer particles (dp =1–7.5 µm) exhibiting most significant intersexual divergence (19,20,33,34). In the concrete case, particles with diameter exceeding 2 µm are characterized by total deposition which is 10–30% greater in women than in men, whereas 1 µm particles do not produce a gender effect on total deposition at all. As already mentioned elsewhere in this contribution smaller dimension of the female tracheobronchial tree with respect to the male one has a noticeable effect on particle deposition, whereby, in the case of large particles, inertial impaction is subject to an enhancement (30). Concerning UFP a similar gender effect is mainly observable for those particles having an inherently high deposition probability (dp <0.1 µm). Although intersexual discrepancies in total, regional, and local particle deposition are usually on the order of several percent and thus may be evaluated as subtle, they may become more essential under certain inhalation conditions.
Regarding the theoretical deposition of UFP in single airway generations of the lungs, the image sketched above finds its full confirmation. Additional information obtainable from the prediction of local deposition fraction among other includes a concentration of deposited particle mass between airway generation 17 and airway generation 21. In addition, local deposition in female lungs exceeds that in male lungs by at least several percent. As found in earlier studies (21,25-28) higher inhalation volumes and respiratory flow rates cause a dislocation of main deposition towards more peripheral structures.
In this context it has to be noted that the rate constant of coagulation is much greater for UFP than for particles measuring >0.1 µm in size. Coagulation, however, results in a continuous increase of particle size and, as a consequence of that, in a change of the TDF if duration of the breath-cycle exceeds a certain threshold value. As argued by Jaques and Kim (4) efficiency of particle coagulation is chiefly dictated by aerosol concentration and a significant change in particle size may be only expected for high particle numbers in the inhaled air stream (>107 particles/m3). For most experimental setups an aerosol concentration of about 105–106 particles/cm3 is measured and breath-cycle time is smaller than 10 s, so that coagulation may be regarded as negligible effect.
A main question concerns the fate of UFP after being deposited on the bronchial and alveolar walls. In general, particulate mass deposited in the respiratory system undergoes a clearance process which can be subdivided into several phases with different clearance velocities (mucociliary clearance, slow bronchial clearance, and alveolar clearance) (35-41). UFPs, which have not been captured on the mucus layer, are taken up by epithelial cells or transferred through the extracellular matrix to a high extent (42) which strongly increases their chance to reach the blood capillaries. There they may induce local effects such as stenosis or may be subject to a further transport through the cardiovascular system and a penetration into main organs of the peripheral body regions. In the worst case they may be the principal cause for a systemic failure of the human organism (3,7) or the initiation of malignant transformations (43,44). If the probability of UFP-induced disease is assumed to correlate with the total lung deposition dose (i.e., the number of deposited particles per unit surface area and time), female lungs seem to undergo a higher health risk than male lungs.
Acknowledgements
None.
Footnote
Conflicts of Interest: The author has no conflicts of interest to declare.
Ethical Statement: This study was approved by the institutional ethic review board and informed consent was obtained from all patients.
References
- Oberdörster G, Ferin J, Gelein R, et al. Role of the alveolar macrophage in lung injury: studies with ultrafine particles. Environ Health Perspect 1992;97:193-9. [Crossref] [PubMed]
- Oberdorster G, Gelein RM, Ferin J, et al. Association of particulate air pollution and acute mortality: involvement of ultrafine particles? Inhal Toxicol 1995;7:111-24. [Crossref] [PubMed]
- Peters A, Wichmann HE, Tuch T, et al. Respiratory effects are associated with the number of ultrafine particles. Am J Respir Crit Care Med 1997;155:1376-83. [Crossref] [PubMed]
- Jaques PA, Kim CS. Measurement of total lung deposition of inhaled ultrafine particles in healthy men and women. Inhal Toxicol 2000;12:715-31. [Crossref] [PubMed]
- Kim CS, Jaques PA. Analysis of total respiratory deposition of inhaled ultrafine particles in adult subjects at various breathing patterns. Aerosol Sci Technol 2004;38:525-40. [Crossref]
- Li N, Sioutas C, Cho A, et al. Ultrafine particulate pollutants induce oxidative stress and mitochondrial damage. Environ Health Perspect 2003;111:455-60. [Crossref] [PubMed]
- Wichmann HE, Peters A. Epidemiological evidence of the effects of ultrafine particle exposure. Phil Trans Royal Soc London A 2000;358:2751-69. [Crossref]
- Donaldson K, Tran CL. Inflammation caused by particles and fibers. Inhal Toxicol 2002;14:5-27. [Crossref] [PubMed]
- Schins RP, Lightbody JH, Borm PJ, et al. Inflammatory effects of coarse and fine particulate matter in relation to chemical and biological constituents. Toxicol Appl Pharmacol 2004;195:1-11. [Crossref] [PubMed]
- Sturm R. Theoretical and experimental approaches to the deposition and clearance of ultrafine carcinogens in the human respiratory tract. Thoracic Cancer 2011;2:61-8. [Crossref]
- Seaton A, Godden D, MacNee W, et al. Particulate air pollution and acute health effects. Lancet 1995;345:176-8. [Crossref] [PubMed]
- Hofmann W, Sturm R, Winkler-Heil R, et al. Stochastic model of ultrafine particle deposition and clearance in the human respiratory tract. Radiat Prot Dosimetry 2003;105:77-80. [Crossref] [PubMed]
- Blanchard JD, Willeke K. Total deposition of ultrafine sodium chloride particles in human lungs. J Appl Physiol 1984;57:1850-6. [PubMed]
- Wilson FJ, Hiller FC, Wilson JD, et al. Quantitative deposition of ultrafine stable particles in the human respiratory tract. J Appl Physiol 1985;58:223-9. [PubMed]
- Schiller CF, Gebhart J, Heyder J, et al. Factors influencing total deposition of ultrafine aerosol particles in the human respiratory tract. J Aerosol Sci 1986;17:328-32. [Crossref]
- Anderson PJ, Wilson JD, Hiller FC. Respiratory tract deposition of ultrafine particles in subjects with obstructive or restrictive lung disease. Chest 1990;97:1115-20. [Crossref] [PubMed]
- Brown JS, Zeman KL, Bennett WD. Ultrafine particle deposition and clearance in the healthy and obstructed lung. Am J Respir Crit Care Med 2002;166:1240-7. [Crossref] [PubMed]
- Sturm R. Theoretical models of carcinogenic particle deposition and clearance in children’s lungs. J Thorac Dis 2012;4:368-76. [PubMed]
- Bennett WD, Zeman KL, Kim C. Variability of fine particle deposition in healthy adults: effect of age and gender. Am J Respir Crit Care Med 1996;153:1641-7. [Crossref] [PubMed]
- Kim CS, Hu SC, Ding J. Deposition distribution of inhaled particles in healthy human lungs: Comparative studies for ultrafine, fine and coarse particles. Am J Respir Crit Care Med 1998;157:A474.
- International commission on radiological protection (ICRP). Human respiratory tract model for radiological protection, Publication 66. Oxford: Pergamon Press, 1994.
- Sturm R, Hofmann W. A theoretical approach to the deposition and clearance of fibers with variable size in the human respiratory tract. J Hazard Mater 2009;170:210-8. [Crossref] [PubMed]
- Sturm R. Theoretical deposition of nanotubes in the respiratory tract of children and adults. Ann Transl Med 2014;2:6. [PubMed]
- Sturm R. Nanotubes in the human respiratory tract - Deposition modeling. Z Med Phys 2015;25:135-45. [Crossref] [PubMed]
- Sturm R. Spatial visualization of theoretical nanoparticle deposition in the human respiratory tract. Ann Transl Med 2015;3:326. [PubMed]
- Sturm R. A stochastic model of carbon nanotube deposition in the airways and alveoli of the human respiratory tract. Inhal Toxicol 2016;28:49-60. [Crossref] [PubMed]
- Sturm R. Inhaled nanoparticles. Physics Today 2016;69:70-1. [Crossref]
- Koblinger L, Hofmann W. Monte Carlo modeling of aerosol deposition in human lungs. Part I: Simulation of particle transport in a stochastic lung structure. J Aerosol Sci 1990;21:661-74. [Crossref]
- Koblinger L, Hofmann W. Analysis of human lung morphometric data for stochastic aerosol deposition calculations. Phys Med Biol 1985;30:541-56. [Crossref] [PubMed]
- Hinds WC, editor. Aerosol Technology: Properties, Behavior, and Measurement of Airborne Particles. New York: John Wiley, 2012.
- Martin TR, Castile RG, Fredberg JJ, et al. Airway size is related to sex but not lung size in normal adults. J Appl Physiol 1987;63:2042-7. [PubMed]
- Eckel HE, Sittel C, Zorowka P, et al. Dimensions of the laryngeal framework in adults. Surg Radiol Anat 1994;16:31-6. [Crossref] [PubMed]
- Pritchard JN, Jefferies SJ, Black A. Sex differences in the regional deposition of inhaled particles in the 2.5–7.5 µm size range. J Aerosol Sci 1986;17:385-9. [Crossref]
- Kim CS, Lewars GA, Sackner MA. Measurement of total lung aerosol deposition as an index of lung abnormality. J Appl Physiol 1985;1988:1527-36. [PubMed]
- Hofmann W, Sturm R. Stochastic model of particle clearance in human bronchial airways. J Aerosol Med 2004;17:73-89. [Crossref] [PubMed]
- Sturm R, Hofmann W. A multi-compartment model for slow bronchial clearance of insoluble particles-extension of the ICRP human respiratory tract models. Radiat Prot Dosimetry 2006;118:384-94. [Crossref] [PubMed]
- Sturm R. A computer model for the clearance of insoluble particles from the tracheobronchial tree of the human lung. Comp Biol Med 2007;37:680-90. [Crossref] [PubMed]
- Sturm R. A three-dimensional model of tracheobronchial particle distribution during mucociliary clearance in the human respiratory tract. Z Med Phys 2013;23:111-9. [Crossref] [PubMed]
- Sturm R, Hofmann W, Scheuch G, et al. Particle clearance in human bronchial airways: comparison of stochastic model predictions with experimental data. Ann Occup Hyg 2002;46:329-39. [Crossref] [PubMed]
- Sturm R, Hofmann W. Stochastic modeling predictions for the clearance of insoluble particles from the tracheobronchial tree of the human lung. Bull Math Biol 2007;69:395-415. [Crossref] [PubMed]
- Sturm R. Age-dependence and intersubject variability of tracheobronchial particle clearance. Pneumon 2011;24:77-84.
- Geiser M, Rothen-Rutishauser B, Kapp N, et al. Ultrafine particles cross cellular membranes by nonphagocytic mechanisms in lungs and in cultured cells. Environ Health Perspect 2005;113:1555-60. [Crossref] [PubMed]
- Deshpande A, Narayanan PK, Lehnert BE. Silica-induced generation of extracellular factor (s) increases reactive oxygen species in human bronchial epithelial cells. Toxicol Sci 2002;67:275-83. [Crossref] [PubMed]
- Bräuner EV, Forchhammer L, Møller P, et al. Exposure to ultrafine particles from ambient air and oxidative stress-induced DNA damage. Environ Health Perspect 2007.1177-82. [Crossref] [PubMed]