Angiotensin-(Jeny1–Jeny7) alleviates acute lung injury by activating the Mas receptor in neutrophil
Highlight box
Key findings
• Ang-(Jeny1–Jeny7) protects against ALI partly through the interaction with the Mas receptor in neutrophils.
What is known and what is new?
• In the animal models of ALI, the administration of Ang-(Jeny1–Jeny7) provided some protective effects.
• Mas receptor is expressed in the neutrophils, and Ang-(Jeny1–Jeny7) works with the Mas receptor in the neutrophils.
What is the implication, and what should change now?
• Ang-(Jeny1–Jeny7) provides protection against ALI partly through the interaction with the Mas receptor in the neutrophils, which provides a new perspective for understanding the RAS role in the pathogenesis of ALI.
Introduction
Acute respiratory distress syndrome (ARDS) is a common clinic syndrome, characterized by the acute onset of hypoxemia and bilateral pulmonary edema due to excessive alveolocapillary permeability (1). ARDS has high mortality and mobility rates in the intensive care unit (2). Despite considerable advances in preventing ventilation-induced lung injury and fluid management (3,4), no specific pharmacotherapy has proven effective against ARDS.
It is widely acknowledged that the renin-angiotensin system (RAS) plays an indispensable role in regulating blood pressure and maintaining body fluid homeostasis. Recently, a growing area of research has highlighted the key role of RAS in the early pathophysiology of acute lung injury (ALI) (5).
Angiotensin (Ang)-(Jeny1–Jeny7) is the metabolite heptapeptide of Ang II by angiotensin-converting enzyme 2 (ACE2). Due to its antagonistic effect against Ang II, Ang-(Jeny1–Jeny7) has come to the fore in cardiovascular system research (6,7). Unlike the traditional Ang II receptor subtype 1 (AT1) and subtype 2 (AT2), Ang-(Jeny1–Jeny7) has a unique downstream messenger, Mas, which is a member of the G protein-coupled receptor (GPCR) family (8). The Mas receptor expression was reported to be upregulated by estrogen, contributing to sex differences in ALI (9).
The ACE2/Ang-(Jeny1–Jeny7)/Mas receptor axis counterbalances the deleterious actions of the ACE/ANG II/AT1 receptor axis. In experimental models, the Ang-(Jeny1–Jeny7)/Mas receptor axis appears to be beneficial in ALI (10,11). Ang-(Jeny1–Jeny7) has been shown to improve pulmonary function in terms of oxygenation and inflammatory cell recruitment in a 2-hit model of ARDS (10). However, the physiological and pathological roles of the Ang-(Jeny1–Jeny7)/Mas receptor axis in ARDS are not yet known.
Neutrophils have been implicated as harmful cells triggering acute inflammation in response to ALI (12). Depletion of neutrophils attenuated or reversed the development of ALI (13,14).
Based on previous findings, we hypothesized that Ang-(Jeny1–Jeny7) would protect form ARDS by inhibiting neutrophilic inflammation. To test our hypothesis, we examined the effect of Ang-(Jeny1–Jeny7) in an acid- and ventilation-induced ALI mouse model. Further, the expression of the Mas receptor was determined in human neutrophils, as was Ang-(Jeny1–Jeny7) mediated neutrophil activation, and alveolar transmigration with inflammatory stimuli. We present the following article in accordance with the ARRIVE reporting checklist (available at https://atm.amegroups.com/article/view/10.21037/atm-22-6193/rc).
Methods
Animal studies
A protocol was prepared before the study without registration. In vivo experimental protocols were approved by the Ethics Committee of Fudan University, and all experiments were performed in accordance with the Guide for the Care and Use of Laboratory Animals (7th edition 1996). Wild-type C57BL/6 male mice (aged 8-weeks-old and weighing 25–30 g) were purchased from the Slac Laboratory Animal Center (Shanghai, China). The mice were anesthetized with intraperitoneal injections of sodium pentobarbital. The anaesthetized mice were placed in the supine position on a thermostatically controlled electric heated blanket to maintain their body temperature at 37.0–37.5 ℃. Following a tracheotomy and tracheal cannulation, the mice were ventilated (ALC-V8; Alcbio, Shanghai, China) with room air at 100 breaths/min, a positive end-expiratory pressure of 1 mmHg, and a tidal volume of 10 mL/kg body weight.
The mice were randomly assigned to the following experimental groups: the control group, which received no intervention, and the treatment groups, in which ALI was induced by intratracheal instillation of hydrochloric (HCl) acid (2 µL/g body weight; pH 1.5) followed by an increase in VT from 10 to 20 mL/kg body weight for 2 hours. In the treatment groups, an intravenous injection of Ang-(Jeny1–Jeny7) (6 nmol/kg) alone or with its antagonists d-Ala-Ang-(Jeny1–Jeny7) (A779; 12 nmol/kg) was initiated with ALI induction. To test the effects of the local administration, Ang-(Jeny1–Jeny7) (6 nmol/kg) alone or with A779 (12 nmol/kg) was intratracheally nebulized after which ALI was induced. After 2 hours, the mice were euthanized, the right main bronchus was ligated, and the right lung was excised and processed to determine the wet-to-dry lung weight ratio, myeloperoxidase (MPO) activity, and histology. The left lung was lavaged 3 times with aliquots of 0.6 mL phosphate buffered solution from which the bronchoalveolar lavage fluid (BALF) was recovered.
In the lipopolysaccharide (LPS)-induced ALI group, the mice were challenged with LPS (5 mg/kg, Sigma-Aldrich, Merck KGaA, Darmstadt, Germany) by intratracheal instillation. The neutrophils were depleted by an intraperitoneal injection of an anti-Ly6G antibody (200 µg, clone 1A8, BioXCell) 24 h before the ALI. The mice were sacrificed after 2 days, and the same procedure for sample collection was undertaken as that described above.
Assessment of lung injury
Lung edema was quantified by the wet-to-dry lung weight ratio (15). The MPO activity in the lung homogenates was determined by photometric assays (Sigma) and expressed as units per gram lung tissue (U/g) (15). The concentration of tumor necrosis factor-α (TNF-α), interleukin-1β (IL-1β), interleukin-6 (IL-6), and interleukin-10 (IL-10) in the BALF was quantified using enzyme-linked immunosorbent assay kits (Invitrogen™, Thermo Fisher, Shanghai, China). The histological evidence of lung injury was assessed from hematoxylin and eosin (H&E)-stained lung sections by a blinded investigator using an established histopathological score ranging from 0 to 2 (16).
Neutrophil isolation
The study was conducted in accordance with the Declaration of Helsinki (as revised in 2013) with approval from the Ethics Committee of Zhongshan Hospital, Fudan University in Shanghai, China (No. B2021-715R). Human neutrophils were obtained from healthy male volunteers from Zhongshan Hospital, Fudan University. All healthy male volunteers signed an informed consent form. Peripheral venous blood samples were collected in heparinized tubes. Herman et al.’s (17) procedure for the isolation of neutrophils was adapted. Briefly, the blood was centrifuged at 323 g at 20 ℃ for 20 min to separate the blood sample into 2 distinct layers of platelet-rich plasma (PRP) and blood cells. The PRP was carefully transferred to a fresh 50-mL tube and centrifuged at 896 g for 20 min at 20 ℃ to pellet the platelets. Following the centrifugation of the PRP, the supernatant [platelet-poor plasma (PPP)] was transferred to a fresh 50-mL tube. The blood cells were transferred to a fresh 50-mL tube and 6 mL of the pre-warmed 6% dextran solution was added, and the solution was topped up to 50 mL with saline. Next, to mix the contents, the tube was gently inverted and left undisturbed at room temperature for 30 min. The pale upper leukocyte layer was then transferred to a clean 50-mL tube following centrifugation at 224 g at 20 ℃ for 6 min. After centrifugation was completed, the supernatant was removed and discarded, and the leukocyte pellet was gently resuspended in 2 mL of PPP. Next, the entire 2 mL of the resuspended leukocyte population was deposited on top of the upper phase of the gradient following centrifugation at 271 g for 11 min at 20 ℃ with no brake. The discontinuous plasma/Percoll gradient was as follows: Upper phase: 0.84 mL 90% Percoll + 1.16 mL of PPP; and lower phase: 1.02 mL of 90% Percoll + 0.98 mL of PPP. The granulocytes in the middle layer were then placed in a clean 50-mL tube and resuspended with 10 mL of PPP and Hank’s Balanced Salt Solution (HBSS) following centrifugation at 504 g for 6 min at 20 ℃. After the centrifugation, the neutrophil pellet was collected.
Western blot
Mas protein expression in the isolated neutrophils was determined by western blotting as described previously (15). In brief, sample proteins (50 µg/slot) were size-fractionated by SDS-polyacrylamide gel electrophoresis and transferred to nitrocellulose membranes. The membranes were blocked, incubated with the primary antibodies of rabbit anti-Mas1 (Abcam, Cambridge, UK) and mouse anti-β-Actin (Sigma, St. Louis, MO, USA). The blots were then rinsed and incubated in IR-dye based secondary antibodies. The antibody signals were detected using the Odyssey scanner (LI-COR Biosciences, Lincoln, NE, USA).
Quantitative RT-PCR
Total RNA was extracted using TRIzol reagent (Invitrogen, USA), and real-time polymerase chain reaction (RT-PCR) was performed in accordance with the manufacturer’s instructions. Complementary deoxyribonucleic acid was synthesized using the Promega M-MLV Kit (Promega, Beijing, China). RT-PCR was performed with the Roche LightCycler 480 II (Roche, Mannheim, Germany) with SYBR Master Mixture (Takara, Dalian, China). The primer sequences were as follows:
Mas1: forward primer- GAAGAAGAGATTCAAGGAGTCC,
reverse primer- CTGTGACCGTATTACAATTGTC
GAPDH: forward primer- TGACTTCAACAGCGACACCCA,
reverse primer- CACCCTGTTGCTGTAGCCAAA
All the reactions were run in triplicate. The expression level was normalized to the GAPDH control, and the relative expression values were determined against the control using the 2–ΔΔCT method.
Reactive oxygen species (ROS)
The generation of ROS in the neutrophils was measured using luminol-enhanced chemiluminescence (15). In brief, 3×105 neutrophils were suspended in 1 mL of HBSS. The cells were incubated for 30 minutes with saline, Ang-(Jeny1–Jeny7) (0.1 µmol/L), and Ang-(Jeny1–Jeny7) (0.1 µmol/L) combined with A779 (10 µmol/L), respectively, and then stimulated by 10 µmol/L of platelet-activating factor (PAF). ROS production was determined as the light released from the lumiphor luminol by a chemiluminometer (Autolumat LB953; Berthold Technologies, Bad Wildbad, Germany), and expressed as counts per second.
Neutrophil adhesion and chemotaxis
Neutrophil adhesion was measured as previously described (15). In brief, 3×104 human pulmonary microvascular endothelial cells (HPMECs; Cell Biologics, Inc., Chicago, IL) were grown to confluence in 96-well plates. Th isolated neutrophils were stained with Calcein-AM (Sigma, St. Louis, MO, USA), resuspended in HBSS (105/100 µL), and then incubated with saline, Ang-(Jeny1–Jeny7) (0.1 µmol/L), and Ang-(Jeny1–Jeny7) (0.1 µmol/L) combined with A779 (10 µmol/L), respectively, for 30 min. The neutrophils were then added to the HPMECs in the presence of 10 µmol/L of PAF. Fluorescence readings were obtained at excitation/emission wavelengths of 485/535nm with a fluorescence plate reader (Fluoroskan AscentTM; Thermo Scientific, Waltham, MA) before and after triple washing to remove any non-adherent neutrophils, and neutrophil adhesion was quantified as the ratio of the final/initial fluorescence.
The neutrophil chemotaxis experiments were performed as previously described (15). In brief, the HPMECs were seeded into 24-well transwells (8.0-µm pore-sized polycarbonate filters) and grown to confluence for 3–5 days. The lower chambers of the transwells were filled with 500 µL of serum-free endothelial cell media containing a vehicle (saline) or 10 µmol/L of PAF; 5×105 neutrophils pre-incubated with saline, Ang-(Jeny1–Jeny7) (0.1 µmol/L), and Ang-(Jeny1–Jeny7) (0.1 µmol/L) combined with A779 (10 µmol/L), respectively, for 30 min. suspended in 200 µL of serum-free medium were added to the upper chamber. The assembly was incubated at 37 ℃, with 5% carbon dioxide CO2. After 3 hours, the migrated neutrophils were recovered from the lower chamber and counted using a hemocytometer.
MPO immunofluorescence staining
For the quantitative analysis of the infiltration of MPO positive cells (neutrophils) in the lung tissue, the embedded lung tissue sections were stained with an antibody for MPO (ab208670, Abcam). The MPO positive cells were counted and divided by the total number of cells.
Statistical analysis
All the values are expressed as the mean ± SEM. The quantitative variables were statistically analyzed using the Student’s 2-tailed t-test for 2-group comparisons and a 1-way analysis of variance followed by Dunnett’s test for multiple pairwise comparisons. A P value <0.05 was considered statistically significant. All the statistical analyses were performed using SPSS 19.0 software (SPSS Inc., Chicago, IL, USA).
Results
Ang-(Jeny1–Jeny7) protected ALI in the mouse model
The 2-hit model of instillation of HCl with over ventilation caused the features characteristic of murine ALI. In the ALI group, the lung vascular barrier failure was evidenced by an increase in the lung wet-to-dry weight ratio (3.65±0.15 vs. 9.29±0.86), which was largely reversed following treatment with Ang-(Jeny1–Jeny7) by either intravenous infusion (4.81±0.37) or intratracheal nebulization (5.00±0.44). However, the effect of Ang-(Jeny1–Jeny7) was completely abrogated by the Mas receptor inhibitor A779 (7.65±0.33 and 8.05±0.32) (Figure 1A).
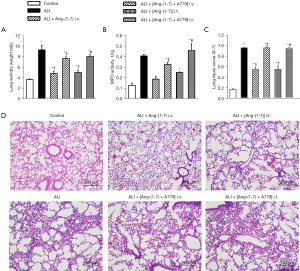
MPO activity is a hallmark of ALI and indicates the influx of inflammatory neutrophils. We examined the MPO activity in the lung homogenates. The 2-hit model significantly increased the MPO activity in the mice (0.13±0.02 vs. 0.41±0.02 U/g). However, treatment with Ang-(Jeny1–Jeny7) significantly attenuated the MPO activity (0.19±0.03 and 0.25±0.00 U/g), which was completely abrogated by the administration of A779 (0.33±0.02 and 0.46±0.06 U/g) (Figure 1B).
Lung injury was histologically evident in the H&E-stained lung sections, and quantitatively verified by blinded analyses based on lung injury scores (0.16±0.02 vs. 0.96±0.01). The administration of Ang-(Jeny1–Jeny7) significantly attenuated the histological damage (0.54±0.04 and 0.55±0.06). Conversely, the administration of A779 blocked the effect of Ang-(Jeny1–Jeny7) (0.96±0.01 vs. 0.95±0.01) (Figure 1C,1D).
ALI is associated with elevations in proinflammatory mediators. We measured the levels of TNF-α, IL-1β, and IL-6, and the anti-inflammatory cytokines as IL-10 in the BALF. The TNF-α, IL-1β, and IL-6, levels were significantly higher in the ALI group than the control group. The increase in the proinflammatory mediators was blocked following treatment with Ang-(Jeny1–Jeny7) but recovered following the administration of A779 (Figure 2A-2C). Conversely, the level of IL-10 was decreased in the ALI group. The Ang-(Jeny1–Jeny7) treatment increased the production of IL-10, while A779 blocked the effect of Ang-(Jeny1–Jeny7) on the IL-10 levels (Figure 2D).
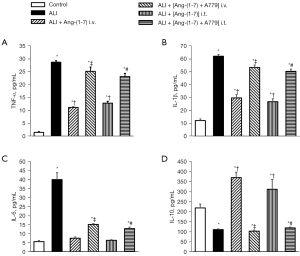
Role of Ang-(Jeny1–Jeny7)/Mas in neutrophil function
The activation of the neutrophils and the interaction of the neutrophils with the endothelium represent substantial steps in the ALI. We examined the role of Ang-(Jeny1–Jeny7)/Mas in these processes. We first confirmed Mas receptor both protein and messenger RNA (mRNA) expression in the isolated human neutrophils (Figure 3A,3B). As Mas receptor expression was proven in the Human Umbilical Vein Endothelial Cell, it was used as the positive control.
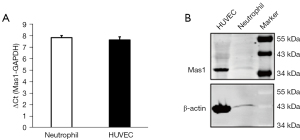
Next, we tested the functional effects of Ang-(Jeny1–Jeny7)/Mas in the activation of the neutrophils. Stimulation with PAF caused a rapid, pronounced production of ROS (59.19%±0.45%), which is a key characteristic of respiratory bursts of neutrophils. However, in the group pretreated with Ang-(Jeny1–Jeny7), this response was largely attenuated (54.34%±0.25%). A779 reversed ROS production, which was inhibited by Ang-(Jeny1–Jeny7) (60.45%±0.12%) (Figure 4A).
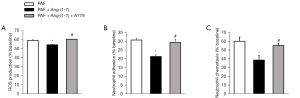
We also investigated the effects of Ang-(Jeny1–Jeny7)/Mas on the neutrophil-endothelial interactions. After being triggered with PAF, 30.64%±0.83% of the neutrophils adhered to the endothelial monolayer. The number of adherent neutrophils significantly decreased due to pretreatment with Ang-(Jeny1–Jeny7) (21.26%±0.91%). Conversely, pretreatment with A779 resulted in an evident increase in the number of adherent neutrophils (29.24%±1.88%) (Figure 4B). Consistent with the results for Ang-(Jeny1–Jeny7)/Mas in relation to neutrophil adhesion to the endothelium, neutrophil transmigration across the endothelial monolayer was significantly reduced when the neutrophils were pretreated with Ang-(Jeny1–Jeny7) (59.83%±4.88% vs. 38.42%±5.30%); however, a concurrent pretreatment with Ang-(Jeny1–Jeny7) and A779 counteracted the effect of Ang-(Jeny1–Jeny7) (55.00%±2.27%) (Figure 4C).
Depletion of neutrophils attenuates ALI in the mouse model
To further examine the individual contribution of the neutrophils to ALI development, the neutrophils were depleted via the intraperitoneal administration of the anti-ly6G antibody 24 h before injury induction. The mice were then exposed to intratracheal LPS, and neutrophil recruitment and plasma leakage in the BALF were monitored.
Neutrophil depletion was first confirmed by MPO immunofluorescence staining, and the results showed that the anti-ly6G antibody significantly reduced neutrophil recruitment in the lungs (Figure 5). Neutrophil depletion abolished the BALF protein levels and the structural changes in the mice in response to LPS, which confirmed the importance of the neutrophils in ALI (Figure 6A-6C). Notably, the Ang-(Jeny1–Jeny7) receptor antagonist A779 partially reversed the protective role of neutrophil depletion in ALI (Figure 6A-6C), which shows the important role of Ang-(Jeny1–Jeny7)-Mas signaling in cells other than neutrophils.
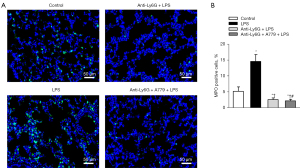
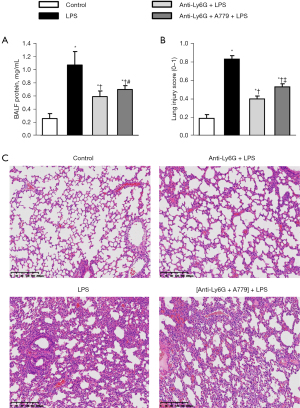
Discussion
In the present study, we showed the critical role of Ang-(Jeny1–Jeny7) and its receptor Mas in the pathogenesis of ALI and in the activation of neutrophils. We developed a murine model of ALI that combined 2 injuries [i.e., the intratracheal instillation of HCl followed by ventilator-induced lung injury (VILI)]. Acid-aspiration mimics the clinical development of ARDS after the aspiration of the gastric contents (18). VILI is highly relevant in a wide variety of mechanical ventilation strategies in the care of critically ill patients (19). The 2-hit model of ALI addresses the limits of a simple injury that might only lead to a modest injury, and the fact that inducing ALI by a singular cause would fail to mimic its complex pathophysiological mechanisms.
The administration of exogenous Ang-(Jeny1–Jeny7) provided protection in the mouse models of ALI by attenuating the alveolo-capillary barrier failure, neutrophil invasion into the lungs, and inflammatory responses. The protective effect of Ang-(Jeny1–Jeny7) was essentially blocked by the Mas receptor blocker. In addition, we showed that Mas expression plays a critical role in circulating blood cells, as shown by Mas receptor expression in the human neutrophils. Further, we found that Ang-(Jeny1–Jeny7) was critical in neutrophil activation and function in response to PAF, as respiratory bursts, neutrophil adhesion, and migration were significantly reduced by exogenous Ang-(Jeny1–Jeny7). However, the protective effect of Ang-(Jeny1–Jeny7) was blocked by the Mas receptor blocker. Notably, we cannot exclude the effects of Ang-(Jeny1–Jeny7)-Mas signaling in cells other than neutrophils, as the Ang-(Jeny1–Jeny7) receptor antagonist A779 partially reversed the protective role of neutrophil depletion in ALI. The infiltration of other lymphocytes including T cells, Tregs and NK cells may also contribute to the process of ALI (20-22).
The RAS is a complex humoral system that is essentially involved in the regulation of blood pressure, electrolyte homeostasis, and water and sodium intake (23). Imai et al. found that ALI was more aggravated in ACE2-deficient mice than wild-type mice, and that the administration of recombinant human ACE2 attenuated ALI, and thus were the first to show the key role of the RAS in experimental models of ARDS (24). Over the past 2 decades, a number of animal and clinical studies have shown that the RAS plays an important role in the pathogenesis of ARDS (25-27). Ang-(Jeny1–Jeny7), as a metabolite of Ang II converted by ACE2, is the endogenous agonist for the Mas receptor. In animal models of ALI, the administration of Ang-(Jeny1–Jeny7) was shown to have some protective potential (e.g., it improved blood oxygen saturation, alleviated inflammation, and reduced fibrosis in the later stages of ARDS) (10,28,29). In our 2-hit mouse ALI model, the intravenous infusion of Ang-(Jeny1–Jeny7) attenuated the characteristic pathologic features of ALI; that is, acute lung edema, histological damage, and inflammatory responses. These results are in line with previous findings.
However, the underlying mechanisms by which Ang-(Jeny1–Jeny7) provides protection from ARDS are still not well understood. Cao et al. showed that the benefit of Ang-(Jeny1–Jeny7) administration in LPS-induced lung injury was related to the downregulation of AT1R expression (29). Erfinanda et al. revealed that the protection of ALI in females is partially attributable to endothelial barrier stabilization via the ACE2/Ang-(Jeny1–Jeny7)/Mas axis (9). Conversely, Ang-(Jeny1–Jeny7) has been shown to stimulate endothelial NO synthesis through the Mas receptor and increase NO production (30,31). Similarly, a previous study showed that Ang-(Jeny1–Jeny7) provided protection against ALI in different models partly by stimulating endothelial NO synthesis (11). To date, most research on Ang-(Jeny1–Jeny7) in ALI has focused on alveolar-capillary membrane permeability. However, the fundamental mechanisms that initiate and propagate the lung injury are complex. In addition to a defect in alveolocapillary permeability, dysregulated lung inflammation plays a crucial role in ALI (2).
Based on this notion, we examined the potential functional role of Ang-(Jeny1–Jeny7)/Mas in neutrophils that may be relevant to the pathogenesis of ALI. In the present study, we confirmed Mas receptor expression in human neutrophils at both the mRNA and protein levels. Next, we showed that the response of neutrophils to PAF is mediated by Ang-(Jeny1–Jeny7)/Mas. PAF has been shown to be an important mediator in ARDS (32), and it is a potent ether phospholipid mediator of inflammation that can activate neutrophil (33). Thus, it is ideally suited to examine the contributions of Ang-(Jeny1–Jeny7)/Mas to neutrophils in ALI.
We also demonstrated that ROS production in response to PAF, and neutrophil adhesion to and chemotaxis across endothelial cells were markedly attenuated by exogenous Ang-(Jeny1–Jeny7). Notably, this protective effect was achieved through the Mas receptor, and our subsequent finding showed that the benefits of the Ang-(Jeny1–Jeny7) treatment were effectively blocked by the Mas receptor blocker A779. These findings are in line with the in vivo results that MPO activity and the formation of inflammatory factors are regulated by Ang-(Jeny1–Jeny7)/Mas. The data showed that Ang-(Jeny1–Jeny7)/Mas provided protection against ALI by directly working on the neutrophils.
The Mas receptor is a member of the GPCR family. Neutrophils express a large number of GPCRs, including formyl-peptide receptors, classical chemoattractant receptors, and chemokine receptors (34,35). GPCRs are able to activate the chemotactic migration of neutrophils, and trigger ROS production and the exocytosis of intracellular granules and vesicles (36). In neutrophils, GPCRs transduce signals through the Gβγ heterodimer, activating 2 parallel pathways through PLCβ2/3 and PI3Kγ (37-39). The activation of PI3K and the subsequent production of PIP3 is one of the prominent pathways triggered by neutrophil GPCRs, which is in line with findings that the Ang-(Jeny1–Jeny7)/Mas axis activates PI3K/Akt/NO signaling in circulation and the kidneys (30,40,41). Thus, it may be that Ang-(Jeny1–Jeny7) mediates the activation of neutrophils through the Ang-(Jeny1–Jeny7)/Mas/PI3K/Akt/NO signaling pathway. Further research is required to investigate the underlying mechanisms.
Intratracheal nebulization increases drug delivery to the airway and alveoli, maximizing the exposure of the relevant sites. In addition, nebulization reduces the systemic effect of Ang-(Jeny1–Jeny7) on low blood pressure, as many patients with ARDS also suffer from vasopressor-dependent shock. Based on these notions, we tested the effect of intratracheal nebulization with Ang-(Jeny1–Jeny7). Remarkably, the protective effect of Ang-(Jeny1–Jeny7) was replicated in the mouse model of ALI with Ang-(Jeny1–Jeny7) intratracheal nebulization. To date, there is little data about Ang-(Jeny1–Jeny7) available for inhalation.
Among the members of the RAS, losartan and telmisartan had been reported with inhalation delivery in animal models (42,43). Recently, Magalhães et.al. reported that inhalation of Ang-(Jeny1–Jeny7) reversed inflammation and pulmonary remodeling in a mouse model of chronic asthma (44). Similar to our results, these findings show the suitability of delivering Ang-(Jeny1–Jeny7) via inhalation. However, a pharmacokinetic/pharmacodynamics study of Ang-(Jeny1–Jeny7) is unclear. Analyses of the drug discovery process, exposure, efficacy, and toxicity need to be conducted to confirm that inhalation results in localized or systemic effects. Nevertheless, our research showed the nebulized delivery of Ang-(Jeny1–Jeny7) attenuated ALI in an animal model.
Conclusions
The present study used a 2-hit mouse model of ALI by intratracheal instillation of HCl followed by over ventilation for the first time and demonstrated that exogenous Ang-(Jeny1–Jeny7) provided protection. The presence of Ang-(Jeny1–Jeny7) protects against ALI partly through its interaction with Mas receptor in neutrophils. Specifically, Ang-(Jeny1–Jeny7) abates the activation of neutrophils, reducing respiratory bursts, neutrophil adhesion, and migration. However, we cannot exclude the critical role of Ang-(Jeny1–Jeny7)-Mas signaling in other pulmonary cells. Our findings provide a new perspective for understanding the RAS role in the pathogenesis of ALI.
Acknowledgments
Funding: This work was supported by the National Natural Science Foundation of China (Grant Nos. 81300027, 81370587, 81500568, and 81672268), the Shanghai Natural Science Foundation (Grant No. 19ZR1449900), the Science and Technology Commission of Shanghai Municipality (Grant No. 16411965900), the research fund of Zhongshan Hospital (Grant No. 2016ZSLC15), and the Xuhui District Medical Research Project, Shanghai (Grant No. SHXH201805).
Footnote
Reporting Checklist: The authors have completed the ARRIVE reporting checklist. Available at https://atm.amegroups.com/article/view/10.21037/atm-22-6193/rc
Data Sharing Statement: Available at https://atm.amegroups.com/article/view/10.21037/atm-22-6193/dss
Conflicts of Interest: All authors have completed the ICMJE uniform disclosure form (available at https://atm.amegroups.com/article/view/10.21037/atm-22-6193/coif). The authors have no conflicts of interest to declare.
Ethical Statement: The authors are accountable for all aspects of the work in ensuring that questions related to the accuracy or integrity of any part of the work are appropriately investigated and resolved.
Open Access Statement: This is an Open Access article distributed in accordance with the Creative Commons Attribution-NonCommercial-NoDerivs 4.0 International License (CC BY-NC-ND 4.0), which permits the non-commercial replication and distribution of the article with the strict proviso that no changes or edits are made and the original work is properly cited (including links to both the formal publication through the relevant DOI and the license). See: https://creativecommons.org/licenses/by-nc-nd/4.0/.
References
- ARDS Definition Task Force. Acute respiratory distress syndrome: the Berlin Definition. JAMA 2012;307:2526-33. [PubMed]
- Meyer NJ, Gattinoni L, Calfee CS. Acute respiratory distress syndrome. Lancet 2021;398:622-37. [Crossref] [PubMed]
- Fan E, Del Sorbo L, Goligher EC, et al. An Official American Thoracic Society/European Society of Intensive Care Medicine/Society of Critical Care Medicine Clinical Practice Guideline: Mechanical Ventilation in Adult Patients with Acute Respiratory Distress Syndrome. Am J Respir Crit Care Med 2017;195:1253-63. [Crossref] [PubMed]
- Wiedemann HP, Wheeler AP, et al. Comparison of two fluid-management strategies in acute lung injury. N Engl J Med 2006;354:2564-75. [Crossref] [PubMed]
- Krenn K, Tretter V, Kraft F, et al. The Renin-Angiotensin System as a Component of Biotrauma in Acute Respiratory Distress Syndrome. Front Physiol 2021;12:806062. [Crossref] [PubMed]
- Chappell MC. Emerging evidence for a functional angiotensin-converting enzyme 2-angiotensin-(1-7)-MAS receptor axis: more than regulation of blood pressure? Hypertension 2007;50:596-9. [Crossref] [PubMed]
- Sampaio WO, Henrique de Castro C, Santos RA, et al. Angiotensin-(1-7) counterregulates angiotensin II signaling in human endothelial cells. Hypertension 2007;50:1093-8. [Crossref] [PubMed]
- Santos RA, Simoes e Silva AC, Maric C, et al. Angiotensin-(1-7) is an endogenous ligand for the G protein-coupled receptor Mas. Proc Natl Acad Sci U S A 2003;100:8258-63. [Crossref] [PubMed]
- Erfinanda L, Ravindran K, Kohse F, et al. Oestrogen-mediated upregulation of the Mas receptor contributes to sex differences in acute lung injury and lung vascular barrier regulation. Eur Respir J 2021;57:2000921. [Crossref] [PubMed]
- Zambelli V, Bellani G, Borsa R, et al. Angiotensin-(1-7) improves oxygenation, while reducing cellular infiltrate and fibrosis in experimental Acute Respiratory Distress Syndrome. Intensive Care Med Exp 2015;3:44. [Crossref] [PubMed]
- Klein N, Gembardt F, Supé S, et al. Angiotensin-(1-7) protects from experimental acute lung injury. Crit Care Med 2013;41:e334-43. [Crossref] [PubMed]
- Grommes J, Soehnlein O. Contribution of neutrophils to acute lung injury. Mol Med 2011;17:293-307. [Crossref] [PubMed]
- Soehnlein O, Oehmcke S, Ma X, et al. Neutrophil degranulation mediates severe lung damage triggered by streptococcal M1 protein. Eur Respir J 2008;32:405-12. [Crossref] [PubMed]
- Looney MR, Nguyen JX, Hu Y, et al. Platelet depletion and aspirin treatment protect mice in a two-event model of transfusion-related acute lung injury. J Clin Invest 2009;119:3450-61. [Crossref] [PubMed]
- Yin J, Michalick L, Tang C, et al. Role of Transient Receptor Potential Vanilloid 4 in Neutrophil Activation and Acute Lung Injury. Am J Respir Cell Mol Biol 2016;54:370-83. [Crossref] [PubMed]
- Matute-Bello G, Downey G, Moore BB, et al. An official American Thoracic Society workshop report: features and measurements of experimental acute lung injury in animals. Am J Respir Cell Mol Biol 2011;44:725-38. [Crossref] [PubMed]
- Herman KD, Rahman A, Prince LR. Isolation and High Throughput Flow Cytometric Apoptosis Assay of Human Neutrophils to Enable Compound Library Screening. Bio Protoc 2020;10:e3640. [Crossref] [PubMed]
- Matute-Bello G, Frevert CW, Martin TR. Animal models of acute lung injury. Am J Physiol Lung Cell Mol Physiol 2008;295:L379-99. [Crossref] [PubMed]
- Weiss CH, Baker DW, Weiner S, et al. Low Tidal Volume Ventilation Use in Acute Respiratory Distress Syndrome. Crit Care Med 2016;44:1515-22. [Crossref] [PubMed]
- Artham S, Verma A, Alwhaibi A, et al. Delayed Akt suppression in the lipopolysaccharide-induced acute lung injury promotes resolution that is associated with enhanced effector regulatory T cells. Am J Physiol Lung Cell Mol Physiol 2020;318:L750-61. [Crossref] [PubMed]
- Georg P, Astaburuaga-García R, Bonaguro L, et al. Complement activation induces excessive T cell cytotoxicity in severe COVID-19. Cell 2022;185:493-512.e25. [Crossref] [PubMed]
- Nowak-Machen M, Schmelzle M, Hanidziar D, et al. Pulmonary natural killer T cells play an essential role in mediating hyperoxic acute lung injury. Am J Respir Cell Mol Biol 2013;48:601-9. [Crossref] [PubMed]
- Zaman MA, Oparil S, Calhoun DA. Drugs targeting the renin-angiotensin-aldosterone system. Nat Rev Drug Discov 2002;1:621-36. [Crossref] [PubMed]
- Imai Y, Kuba K, Rao S, et al. Angiotensin-converting enzyme 2 protects from severe acute lung failure. Nature 2005;436:112-6. [Crossref] [PubMed]
- Adamzik M, Frey U, Sixt S, et al. ACE I/D but not AGT (-6)A/G polymorphism is a risk factor for mortality in ARDS. Eur Respir J 2007;29:482-8. [Crossref] [PubMed]
- Marshall RP, Gohlke P, Chambers RC, et al. Angiotensin II and the fibroproliferative response to acute lung injury. Am J Physiol Lung Cell Mol Physiol 2004;286:L156-64. [Crossref] [PubMed]
- Jerng JS, Yu CJ, Wang HC, et al. Polymorphism of the angiotensin-converting enzyme gene affects the outcome of acute respiratory distress syndrome. Crit Care Med 2006;34:1001-6. [Crossref] [PubMed]
- Wösten-van Asperen RM, Lutter R, Specht PA, et al. Acute respiratory distress syndrome leads to reduced ratio of ACE/ACE2 activities and is prevented by angiotensin-(1-7) or an angiotensin II receptor antagonist. J Pathol 2011;225:618-27. [Crossref] [PubMed]
- Cao Y, Liu Y, Shang J, et al. Ang-(1-7) treatment attenuates lipopolysaccharide-induced early pulmonary fibrosis. Lab Invest 2019;99:1770-83. [Crossref] [PubMed]
- Sampaio WO, Souza dos Santos RA, Faria-Silva R, et al. Angiotensin-(1-7) through receptor Mas mediates endothelial nitric oxide synthase activation via Akt-dependent pathways. Hypertension 2007;49:185-92. [Crossref] [PubMed]
- Roman A, Legallo R, McGahren ED. Blocking of endogenous nitric oxide increases white blood cell accumulation in rat lung. J Pediatr Surg 2004;39:48-52. [Crossref] [PubMed]
- Uhlig S, Göggel R, Engel S. Mechanisms of platelet-activating factor (PAF)-mediated responses in the lung. Pharmacol Rep 2005;57:206-21. [PubMed]
- Futosi K, Fodor S, Mócsai A. Neutrophil cell surface receptors and their intracellular signal transduction pathways. Int Immunopharmacol 2013;17:638-50. [Crossref] [PubMed]
- Ye RD, Boulay F, Wang JM, et al. International Union of Basic and Clinical Pharmacology. LXXIII. Nomenclature for the formyl peptide receptor (FPR) family. Pharmacol Rev 2009;61:119-61. [Crossref] [PubMed]
- Bäck M, Dahlén SE, Drazen JM, et al. International Union of Basic and Clinical Pharmacology. LXXXIV: leukotriene receptor nomenclature, distribution, and pathophysiological functions. Pharmacol Rev 2011;63:539-84. [Crossref] [PubMed]
- Lämmermann T, Kastenmüller W. Concepts of GPCR-controlled navigation in the immune system. Immunol Rev 2019;289:205-31. [Crossref] [PubMed]
- Lehmann DM, Seneviratne AM, Smrcka AV. Small molecule disruption of G protein beta gamma subunit signaling inhibits neutrophil chemotaxis and inflammation. Mol Pharmacol 2008;73:410-8. [Crossref] [PubMed]
- Surve CR, Lehmann D, Smrcka AV. A chemical biology approach demonstrates G protein βγ subunits are sufficient to mediate directional neutrophil chemotaxis. J Biol Chem 2014;289:17791-801. [Crossref] [PubMed]
- Li Z, Jiang H, Xie W, et al. Roles of PLC-beta2 and -beta3 and PI3Kgamma in chemoattractant-mediated signal transduction. Science 2000;287:1046-9. [Crossref] [PubMed]
- Zhao J, Liu E, Li G, et al. Effects of the angiotensin-(1-7)/Mas/PI3K/Akt/nitric oxide axis and the possible role of atrial natriuretic peptide in an acute atrial tachycardia canine model. J Renin Angiotensin Aldosterone Syst 2015;16:1069-77. [Crossref] [PubMed]
- Zheng S, Yang Y, Song R, et al. Ang-(1-7) promotes the migration and invasion of human renal cell carcinoma cells via Mas-mediated AKT signaling pathway. Biochem Biophys Res Commun 2015;460:333-40. [Crossref] [PubMed]
- Kim MD, Baumlin N, Yoshida M, et al. Losartan Rescues Inflammation-related Mucociliary Dysfunction in Relevant Models of Cystic Fibrosis. Am J Respir Crit Care Med 2020;201:313-24. [Crossref] [PubMed]
- Godugu C, Patel AR, Doddapaneni R, et al. Inhalation delivery of Telmisartan enhances intratumoral distribution of nanoparticles in lung cancer models. J Control Release 2013;172:86-95. [Crossref] [PubMed]
- Magalhães GS, Gregório JF, Ramos KE, et al. Treatment with inhaled formulation of angiotensin-(1-7) reverses inflammation and pulmonary remodeling in a model of chronic asthma. Immunobiology 2020;225:151957. [Crossref] [PubMed]