Blastocyst culture of non-top-quality cleavage embryos may increase the risk of anembryonic pregnancy following in vitro fertilization: a retrospective cohort study
Highlight box
Key findings
• The occurrence of AP may be due to epigenetic abnormalities associated with advanced maternal age and extended in vitro embryo culture, while CoQ10 supplementation may be a potential method in preventing AP.
What is known and what is new?
• AP is a frequent presentation of EPL but the pathogenesis of its occurrence and its association with ART has not been well documented.
• Advanced maternal age and extended culture of non-top-quality embryos may increase the risk of AP due to possible maternal epigenetic abnormalities, while CoQ10 supplementation may be a potential method in preventing AP.
What is the implication, and what should change now?
• Embryo quality on day 3 of the relevant ovum pickup cycle should be taken into consideration in a PGT cycle when all the non-top-quality embryos went through blastocyst culture. CoQ10 supplementation could be recommended in ART treatments.
Introduction
Early pregnancy loss (EPL) occurs in around 15% of naturally occurring pregnancies during the first trimester (1,2). Anembryonic pregnancy (AP), also known as blighted ovum, is EPL characterized by the absence of a visible embryo on ultrasound examination (3). AP is a frequent presentation of EPL with several retrospective analyses demonstrating that almost half of EPLs in natural conceptions were AP (3,4). While little is known about the pathogenesis of AP, it may be related to early embryonic death with continued development of the trophoblast and deficient chorionic villous vascularization (5,6). Although many experts believed that genomic abnormalities might be one of the major causes of AP, results have been inconsistent. Two studies showed no significant differences in the rate of chromosomal abnormalities between EPLs with and without an embryo (7,8), whilst several more recent studies showed a significantly lower rate of chromosomal abnormalities in anembryonic miscarriages indicating possible non-genomic anomalies taking part in its occurrence. (9-11). Two reports suggested that in vivo and in vitro environmental factors may lead to abnormal development of oocytes or embryos, resulting in AP. A case-control study by Yin et al. indicated that higher concentrations of plasma lead are associated with an increased risk of AP (12). Another study by the same group found that women with AP had statistically significantly lower levels of folate and higher levels of homocysteine (13).
Assisted reproductive technology (ART)-related manipulations, including controlled ovarian stimulation (COS), in vitro fertilization (IVF), intracytoplasmic sperm injection (ICSI), and embryo culture and cryopreservation, may directly influence the quality of oocytes and embryos. The association between ART and EPL has been widely studied (14-16). In a large-sample-sized retrospective cohort study in 2016 (14), uterine factor was identified as a significant risk factor for pregnancy loss among infertility diagnoses and an increased risk of loss could be observed in women with diminished ovarian reserve aged 30–34 years and in women with ovulatory dysfunction younger than 35 years. However, in a most recent study, no significant association could be observed between EPL and maternal age, mean hormone levels on trigger day, number of oocytes or embryo quality (15). The inconsistency of the results might be due to the differences in study design, observation endpoints or the various ethnicities of the candidates. Nevertheless, most of those studies did not take the different types of EPL into consideration, even though the pathogeneses of them are probably different.
Here in this study, we analyzed the association between ART and AP using normal live births (NLB) and EPL with embryos (EE) as references, in order to rule out the similar reason in the occurrence of both EE and AP. Meanwhile, nutritional supplementation during ART treatment was analyzed to identify the potential benefit in preventing this most severe dysmorphogenesis of human embryo development. We hope our results may help us understand the pathogenesis of AP and find out the potential safety issue in ART treatments. We present the following article in accordance with the STROBE reporting checklist (available at https://atm.amegroups.com/article/view/10.21037/atm-22-5809/rc).
Methods
Participants
A retrospective cohort study was conducted at Nanfang Hospital, Southern Medical University, South China. Data were retrieved from computerized patient records at Nanfang Hospital between January 2014 and December 2017. A total of 2,662 singleton intrauterine pregnancies were examined, excluding ectopic pregnancies, patients with incomplete clinical information, and those who were lost to follow-up. Fifty-six were excluded because of autoimmune abnormalities, congenital uterine malformations and use of gamete donors. Of the rest 2,606 singleton intrauterine pregnancies, 841 were further excluded for the following reasons: complete miscarriage, second- or third-trimester pregnancy loss, perinatal death or medical termination of pregnancy, pre-term or post-term labor, low birth weight or fetal macrosomia and birth defects. Finally, 1,765 singleton pregnancies without immunological, endocrinological, nor anatomical abnormalities were used to study the correlation between ART and AP (inclusion and exclusion criteria for participation are shown in Figure S1).
Participants were grouped according to pregnancy outcomes, namely, NLB, EE, and AP. ART-related manipulations and early IVF outcomes in the relevant ovum pick-up cycle were compared.
Patients were not directly involved in this research, and no results arising from the study were returned to them. A Core Outcome Set (COS) was not used in the design of this study.
Ethical approval
The study was approved by the Institutional Review Board for Nanfang Hospital (No. NFEC-2017-055). The study was conducted in accordance with the Declaration of Helsinki (as revised in 2013). Due to the retrospective nature of the study, the requirement of written informed consent was waived.
Definition of pregnancy outcomes
In the IVF-population, a routine blood test for plasma beta-human chorionic gonadotropin (β-HCG) was performed at 12days after the embryo cleavage stage or 10 days after blastocyst transfer. If the β-HCG concentration was >100 mIU/mL, trans-vaginal ultrasound (TVS) examination was performed 30, 37, or 44–63 days after embryo transfer. A clinical pregnancy was defined as an intrauterine gestational sac with a heartbeat 30 days after embryo transfer. A NLB was defined as a full-term birth (37–42 weeks) with normal body weight infants (2,500–4,000 g). Early miscarriage was defined as spontaneous pregnancy loss prior to 13 weeks gestation. AP was diagnosed if the embryonic pole was invisible in two consecutive trans-vaginal sonographic (TVS) examinations.
Ovarian stimulation and oocyte retrieval
Patients undergoing ART were treated with gonadotropin-releasing hormone (GnRH)-agonist or -antagonist protocols. In both cases, ovarian stimulation was performed until at least 2 follicles reached a diameter of ≥18 mm, followed by administration of 5,000–10,000 IU human chorionic gonadotropin (Livzon Pharmaceutical Group Inc., China) to induce final follicular maturation. Oocyte retrieval was performed 36 hours later by TVS-guided follicle aspiration, and luteal phase support was given via daily injections of 60mg progesterone (Zhejiang Xianju Pharmaceutical Co., China).
Embryo culture, fresh embryo transfer and cryopreservation
Oocytes were cultured individually and fertilized by IVF or ICSI. Fertilization was checked the next day. Embryos were cultured individually in microdroplets under oil (Vitrolife, Sweden) at 37 °C, using 5% O2 and 6% CO2, and embryo development was evaluated daily. A maximum of 3 embryos were transferred on day 3, and embryo morphological assessment was conducted by 2 fixed embryologists. According to The Istanbul consensus workshop (2011) on embryo assessment, a top-quality embryo was defined as normal fertilized (2PN) embryos characterized by the presence of 4 blastomeres on day 2 and 7–8 blastomeres on day 3, with the absence of multinucleated blastomeres and <10% cellular fragments. Almost all the top-quality embryos were cryopreserved on day 3, while the remaining embryos with no visible pronucleus (0PN) or with a single pronucleus (1PN) fertilized eggs, as well as non-top-quality normal fertilized (2PN) embryos (including good and impaired quality embryos) were selected for blastocyst culture. Exceptionally, in patients with a scarred uterus or with previous late trimester miscarriage due to cervical incompetence, and in patients who requested blastocyst transfer, all day 3 embryos were sent for blastocyst culture. It was recommended that patients having fewer than one top-quality embryo could cryopreserve the remaining good quality embryos. Furthermore, impaired quality embryos were sent for blastocyst culture and embryo grade higher than 3BB was defined as top-quality blastocyst according to the Istanbul consensus (17). Sequential media was used for blastocyst culture following the manufacturer’s instructions (Vitrolife, Sweden). Vitrification was performed using a Kitazoto Vitrification Kit (Japan). Embryo quality was recorded on day 3 of the relevant ovum pick-up cycle and before transfer in the frozen-thawed cycle.
Frozen-thawed embryo transfer (FET)
In a FET cycle, hormone replacement treatment (HRT) protocols were used for endometrial preparation. A maximum of three embryos were transferred. Embryos were warmed on the day of embryo transfer, and luteal phase support was given by daily injections of 60mg progesterone (Zhejiang Xianju Pharmaceutical Co., China).
IVF outcome measures
The total number of mature oocytes was defined as the number of oocytes in meiosis II (MII) for an ICSI cycle and the number of fertilized oocytes in an IVF cycle. Normal fertilized oocytes were 2PN oocytes on the second day of fertilization.
Blood samples and hormone assays
Blood samples were taken prior to treatment on days 2–5 of the patient’s spontaneous menstrual cycle for assessment of follicle stimulating hormone (FSH), luteinizing hormone (LH), and estradiol (E2) levels. Serum E2, LH, and progesterone (P4) levels were also measured on the day of HCG administration. Serum hormonal levels were measured using an electrochemiluminescence (ECL) immunoassay (Roche, USA).
Semen analysis
Semen analysis was performed according to the WHO laboratory manual (18), including evaluation of ejaculate volume, concentration of spermatozoa, and sperm morphology and motility. Mild to moderate impairment of semen quality was defined as sperm concentration of 2–15×106/mL, 11–32% progressive motility sperm, or 2–3% normal morphology sperm. Severely impaired semen quality was defined as sperm concentration less than 2×106/mL, less than 10% progressive motility sperm, or less than 1% normal morphology sperm. Azoospermia was defined as no sperm seen following centrifugation.
DNA isolation and karyotype analysis
A total of 328 patients sent their products of conception (POC) for karyotype analysis following the doctor’s advice, including 99 AP patients (84.6%) and 229 EE (81.7%) patients. Genomic DNA was extracted using the Animal Tissue DNA Kit (Simgen, Hangzhou, China) and karyotype analysis was performed by multiplex ligation-dependent probe amplification (MLPA, MRC-Holland, the Netherlands) according to manufacturer’s instructions. Maternal cell contamination (MCC) was excluded by examination of microsatellite markers in the POC samples using an AGCU 17+1 kit (AGCU ScienTech Incorporation, Wu Xi, China).
Statistical analysis
Continuous data were indicated with mean ± standard deviation (SD) while categorical data were indicated with number and percentage (%). The normality of the continuous variables was determined using the Kolmogorov-Smirnov test, and demographic and baseline reproductive characteristics of different groups of patients were compared using one-way analysis of variance (ANOVA) for continuous variables and a chi-squared test for categorical variables. Multivariable logistic regression was used to evaluate the association between ART-related manipulations and the occurrence of AP. Variables examined included maternal age, paternal age, female body mass index (BMI), total antral follicle count, baseline FSH, infertility duration, infertility type, infertility factors, history of spontaneous miscarriages, total dose of gonadotropins, embryo quality, serum β-HCG level, and different stages of embryos. The selected variables are potential factors that were previously published to be possibly associated with ART outcomes. All tests were two-tailed and the level of statistical significance was set at P<0.05, and factors were determined to be positively or negatively associated with AP when the odds ratio (OR) was higher or lower than 1, respectively. Analysis was performed using SPSS statistical software version 12.0.1 for Windows.
Results
Demographics
Basic characteristics of the participants were summarized in Table 1. In the 1,765 singleton pregnancies, fresh embryo transfer was performed in 946 cases, while the remaining 819 cases were frozen-thawed cycles. There were 1,368 NLBs, 280 EEs, and 117 APs. The overall percentage of APs in EPL was 29.5% (117/397). Parental age (P<0.001), duration of infertility (P<0.001), and the percentage of secondary infertility (P=0.004) were significantly higher for both the AP and EE groups compared to the NLB group. Female BMI was significantly higher in the AP group than in the NLB group (P=0.013). There were no significant differences between groups for any of the other characteristics examined (Table 1).
Table 1
Characteristics | NLB (n=1,368) | EE (n=280) | AP (n=117) | P value |
---|---|---|---|---|
Paternal age (years) | 33.72±5.18 | 35.82±5.32* | 35.74±5.35* | <0.001 |
Maternal age (years) | 31.13±4.16 | 33.64±5.05* | 33.18±4.55* | <0.001 |
BMI | 21.26±2.70 | 21.55±2.81 | 21.96±2.79* | 0.013 |
AFC | 15.34±7.57 | 14.73±7.71 | 14.91±7.78 | 0.426 |
Baseline FSH (mIU/mL) | 6.83±2.26 | 6.87±2.41 | 6.52±1.78 | 0.328 |
Baseline LH (mIU/mL) | 5.31±2.97 | 5.49±3.24 | 5.09±2.33 | 0.467 |
Baseline E2 (pg/mL) | 38.28±16.55 | 39.58±16.43.19 | 37.04±16.12 | 0.318 |
Infertility duration (years) | 4.35±2.88 | 5.35±4.01* | 4.91±3.40 | <0.001 |
Previous miscarriage | 0.201 | |||
0 | 1239 (90.6%) | 239 (85.4%) | 106 (90.6%) | |
1 | 115 (8.4%) | 35 (12.5% | 9 (7.7%) | |
2 | 11 (0.8%) | 5 (1.8%) | 2 (1.7%) | |
>2 | 3 (0.2%) | 1 (0.4%) | 0 (0%) | |
Infertility type | 0.004 | |||
Primary | 716 (52.3%) | 118 (42.1%)* | 53 (45.3%)* | |
Secondary | 652 (47.7%) | 162 (57.9%)* | 64 (54.7%)* | |
Infertility factors | 0.750 | |||
Maternal factors | 938 (68.6%) | 199 (71.1%) | 78 (66.7%) | |
Paternal factors | 259 (18.9%) | 20 (7.1%) | 19 (16.2%) | |
Both parental factors | 143 (10.5%) | 26 (9.3%) | 16 (13.7%) | |
Unexplained | 28 (2.0%) | 5 (1.8%) | 4 (3.4%) | |
Paternal factors | 0.591 | |||
None | 543 (39.7%) | 119 (42.5%) | 43 (36.8%) | |
Mild to moderate | 522 (38.2%) | 109 (38.9%) | 47 (40.2%) | |
Severe | 190 (13.9%) | 36 (12.9%) | 16 (13.7%) | |
Azoospermia | 113 (8.3%) | 16 (5.7%) | 11 (9.4%) | |
Tubal factors | 0.846 | |||
Yes | 1072 (78.4%) | 222 (79.3%) | 94 (80.3%) | |
No | 296 (21.6%) | 58 (20.7%) | 23 (19.7%) | |
Endometriosis | 0.204 | |||
Yes | 107 (7.8%) | 23 (8.2%) | 9 (7.7%) | |
No | 659 (48.2%) | 155 (55.4%) | 61 (52.1%) | |
Unknown | 602 (44.0%) | 102 (36.4%) | 47 (40.2%) | |
Ovulation disorders | 0.877 | |||
Yes | 207 (15.1%) | 40 (14.3%) | 19 (16.2%) | |
No | 1161 (84.9%) | 240 (85.7%) | 98 (83.8%) |
Continuous data were indicated with mean ± SD while categorical data were indicated with number and percentage (%). *, indicates statistically significant difference between the EE/AP cohort and the NLB cohort. NLB, normal live births; EE, early pregnancy loss with embryo; AP, anembryonic pregnancy; BMI, body mass index; AFC, antral follicle counts; FSH, follicle stimulating hormone; LH, luteinizing hormone; E2, estradiol; SD, standard deviation.
ART-related manipulations and AP
Variables related to the relevant ovum pick-up cycle for each of the 3 groups were compared and summarized in Table 2. The total dose of gonadotrophins administered to the EE group was significantly higher than to the NLB or AP groups (P<0.001), while no statistical difference was observed between the AP and NLB groups (P=0.256). Serum β-HCG levels were significantly lower in both the EE (P<0.001) and AP (P<0.001) groups compared to the NLB group. No statistical differences were observed between groups for any of the other variables examined.
Table 2
Outcomes | NLB (n=1,368) | EE (n=280) | AP (n=117) | P value |
---|---|---|---|---|
Total dose of gonadotrophins (IU) | 2,226±951 | 2,613±1191* | 2,374±1027 | <0.001 |
Total days of controlled ovarian stimulation | 10.4±2.3 | 10.7±2.8 | 10.3±2.0 | 0.115 |
E2 at HCG administration (pg/mL)# | 2,371±1,132 | 2,287±1,177 | 2,561±1,472 | 0.375 |
LH at HCG administration (pg/mL)# | 2.3±1.8 | 2.5±1.9 | 2.1±1.4 | 0.420 |
P4 at HCG administration (pg/mL)# | 0.79±0.27 | 0.78±0.30 | 0.80±0.31 | 0.919 |
Number of oocytes retrieved | 13.2±6.8 | 12.7±7.3 | 13.7±7.2 | 0.325 |
Number of mature oocytes | 11.5±6.2 | 11.1±6.6 | 12.0±6.3 | 0.401 |
Number of fertilized oocytes | 10.2±5.8 | 9.9±6.2 | 11.0±5.8 | 0.173 |
Number of normal fertilized oocytes | 8.3±4.8 | 8.0±5.1 | 9.1±4.7 | 0.143 |
Number of formed top-quality embryos | 4.1±3.1 | 3.9±3.4 | 4.4±3.0 | 0.422 |
Controlled ovarian hyperstimulation | 0.164 | |||
Agonist protocol | 1122 (82.0%) | 216 (77.1%) | 95 (81.2%) | |
Antagonist protocol | 246 (18.0%) | 64 (22.9%) | 22 (18.8%) | |
Fertilization method | 0.483 | |||
IVF | 1046 (76.5%) | 212 (75.7%) | 92 (78.6%) | |
ICSI | 292 (21.3%) | 57 (20.4%) | 23 (19.7%) | |
IVF-ICSI | 30 (2.2%) | 11 (3.9%) | 2 (1.7%) | |
Endometrial thickness on the day of ET (mm) | 1.1±0.3 | 1.0±0.2 | 1.0±0.2 | 0.459 |
Days after ET at serum β-HCG testing | 11.3±0.8 | 11.2±1.2 | 11.2±1.5 | 0.174 |
Serum β-HCG (mIU/mL) | 310.5±292.3 | 244.7±195.5* | 184.1±194.1*¶ | <0.001 |
Continuous data were indicated with mean ± SD while categorical data were indicated with number and percentage (%). #, indicates the analysis of data only from fresh embryo transfer cycle with the number of NLB, EE, and AP being 758, 140, and 46, respectively. *, indicates statistically significant differences from NLB cases. ¶, indicates statistically significant differences between the EE and AP cohorts. NLB, normal live births; EE, early pregnancy loss with embryo; AP, anembryonic pregnancy; E2, estradiol; LH, luteinizing hormone; P4, progesterone; HCG, human chorionic gonadotropin; IVF, in vitro fertilization; ICSI, intracytoplasmic sperm injection; ET, embryo transfer; SD, standard deviation.
The embryo transfer profile and outcomes for fresh and frozen-thawed cycles are summarized in Figure 1. A total of 63% (942/1,495) of cleavage stage embryos were transferred in fresh cycle, while 98.5% (266/270) of blastocysts were transferred in FET cycle. Briefly, the percentage of AP in EPL was significantly higher in FET cycle (34.0% vs. 24.5%, P=0.047). However, since the number of fresh blastocyst transfer cases was very limited (n=4), when only cleavage stage embryo transfer cycles were included, no statistical difference could be observed between fresh and frozen cycles (Figure 2A), although there appeared to be a tendency towards a slight increase in the incidence of AP using FET (29.4% vs. 24.6%, P=0.735).
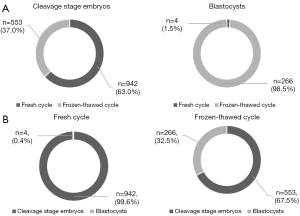

To achieve 1,765 successful singleton pregnancies, 3,127 cleavage stage embryos and 473 blastocysts were transferred, including 2,656 and 100 top-quality embryos on day 3 of the relevant ovum pick-up cycle, respectively. The top-quality rate of transferred embryos evaluated on day 3 was significantly lower in blastocyst transfer cycle (21.1% vs. 84.9%, P<0.001). However, when evaluated before transfer, the top-quality rate was significantly higher in blastocysts (88.6% vs. 82.4%, P=0.001). The percentage of AP in EPLs was 40.0% of blastocyst transfers, which was almost 15% more than cleavage stage embryo transfer (26.6%, P=0.022). Excluding totally and partially top-quality embryo transfer cycles, transferring totally non-top-quality originated blastocysts almost doubled the incidence of AP in EPL (45.9% vs. 24.4%, P=0.037). Although the AP percentage was as high as 55.6% in totally top-quality, and as low as 6.7% in partially top-quality originated blastocysts, the relevant sample size was too small to reach a statistical significance (P=0.068 and P=0.167, respectively; Figure 2B). A significantly lower rate of oral supplementation of CoQ10 before oocyte retrieval could be observed in the AP group compared with both the EE and NLB groups (32.5% vs. 48.6% and 55.8%, respectively, P<0.001; Figure 3).
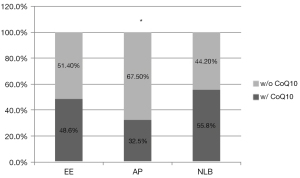
Using multivariable logistic regression model, we found that blastocyst transfer might be a risk factor for the occurrence of AP compared with cleavage stage embryo transfer using NLB (OR: 3.829, P=0.001) or EE as controls (OR: 3.959, P=0.002). Advanced maternal age might also increase the risk of AP (OR: 1.098, P=0.006) and deceased β-HCG level might indicate the occurrence of AP (OR <1, P<0.001), while CoQ10 supplementation might be a protective factor of AP (OR <1, P<0.001; Table 3).
Table 3
Variable | Regression coefficient | Standard error | P | OR | 95% CI | |
---|---|---|---|---|---|---|
Lower limit | Upper limit | |||||
Using NLB as a reference | ||||||
Female age | 0.155 | 0.037 | 0.000 | 1.168 | 1.087 | 1.254 |
Male age | 0.030 | 0.026 | 0.253 | 1.030 | 0.979 | 1.085 |
Female BMI | 0.031 | 0.038 | 0.407 | 1.032 | 0.958 | 1.111 |
Total antral follicle count | 0.001 | 0.022 | 0.967 | 1.001 | 0.959 | 1.044 |
Baseline FSH | −0.076 | 0.057 | 0.180 | 0.927 | 0.830 | 1.036 |
Infertility duration | −0.007 | 0.033 | 0.836 | 0.993 | 0.930 | 1.060 |
Oral supplementation of CoQ10 before oocyte retrieval | ||||||
Yes | −1.614 | 0.242 | 0.000 | 0.199 | 0.124 | 0.320 |
No | ||||||
Total dose of gonadotrophins | 0.000 | 0.000 | 0.494 | 1.000 | 1.000 | 1.000 |
Number of top-quality embryo transferred1 | 0.555 | 0.286 | 0.052 | 1.742 | 0.995 | 3.049 |
Number of top-quality embryo transferred2 | −0.326 | 0.285 | 0.252 | 0.722 | 0.413 | 1.261 |
Serum β-HCG | −0.004 | 0.001 | 0.000 | 0.996 | 0.994 | 0.997 |
Infertility type | ||||||
Primary | −0.138 | 0.239 | 0.564 | 0.871 | 0.545 | 1.392 |
Secondary | – | – | – | – | – | – |
Paternal factors | ||||||
Azoospermia | −0.418 | 0.389 | 0.283 | 0.659 | 0.307 | 1.412 |
Oligozoospermia | −0.535 | 0.421 | 0.204 | 0.585 | 0.256 | 1.337 |
None | – | – | – | – | – | – |
Polycystic ovary syndrome | ||||||
Yes | −0.267 | 0.409 | 0.514 | 0.766 | 0.343 | 1.708 |
No | – | – | – | – | – | – |
Endometriosis | ||||||
Yes | −0.210 | 0.231 | 0.363 | 0.810 | 0.515 | 1.274 |
Unknown | 0.075 | 0.406 | 0.854 | 1.078 | 0.486 | 2.390 |
No | – | – | – | – | – | – |
Previous miscarriage | ||||||
Yes | −0.149 | 0.372 | 0.689 | 0.861 | 0.415 | 1.786 |
No | – | – | – | – | – | – |
Different stage of embryo | ||||||
Blastocyst | 1.715 | 0.395 | 0.000 | 5.559 | 2.563 | 12.057 |
Cleavage | – | – | – | – | – | – |
Using EE as a reference | ||||||
Female age | 0.009 | 0.041 | 0.818 | 1.009 | 0.932 | 1.093 |
Male age | 0.029 | 0.029 | 0.314 | 1.030 | 0.972 | 1.091 |
Female BMI | 0.055 | 0.042 | 0.187 | 1.057 | 0.973 | 1.147 |
Total antral follicle count | −0022 | 0.024 | 0.359 | 0.978 | 0.934 | 1.025 |
Baseline FSH | −0.043 | 0.061 | 0.477 | 0.958 | 0.850 | 1.079 |
Infertility duration | −0.049 | 0.036 | 0.174 | 0.953 | 0.888 | 1.022 |
Oral supplementation of CoQ10 before oocyte retrieval | ||||||
Yes | −0.769 | 0.264 | 0.004 | 0.464 | 0.276 | 0.777 |
No | – | – | – | – | – | – |
Total dose of gonadotrophins | 0.000 | 0.000 | 0.046 | 1.000 | 0.999 | 1.000 |
Number of top-quality embryo transferred 1 | 0.638 | 0.311 | 0.041 | 1.892 | 1.028 | 3.484 |
Number of top-quality embryo transferred 2 | −0.322 | 0.310 | 0.299 | 0.725 | 0.395 | 1.330 |
Serum β-HCG | −0.003 | 0.001 | 0.001 | 0.997 | 0.996 | 0.999 |
Infertility type | ||||||
Primary | 0.057 | 0.265 | 0.828 | 1.059 | 0.630 | 1.779 |
Secondary | – | – | – | – | – | – |
Paternal factors | ||||||
Azoospermia | −0.531 | 0.453 | 0.241 | 0.588 | 0.242 | 1.429 |
Oligozoospermia | −0.551 | 0.487 | 0.258 | 0.576 | 0.222 | 1.497 |
None | – | – | – | – | – | – |
Polycystic ovary syndrome | ||||||
Yes | −0.299 | 0.460 | 0.515 | 0.741 | 0.301 | 1.826 |
No | – | – | – | – | – | – |
Endometriosis | ||||||
Yes | 0.144 | 0.256 | 0.572 | 1.155 | 0.700 | 1.906 |
Unknown | 0.229 | 0.449 | 0.611 | 1.257 | 0.521 | 3.029 |
No | – | – | – | – | – | – |
Previous miscarriage | ||||||
Yes | −0.443 | 0.394 | 0.260 | 0.642 | 0.297 | 1.388 |
No | – | – | – | – | – | – |
Different stage of embryo | ||||||
Blastocyst | 1.261 | 0.437 | 0.004 | 3.528 | 1.497 | 8.317 |
Cleavage | – | – | – | – | – | – |
1, embryo quality was evaluated on day 3 of the relevant ovum pick-up cycle; 2, embryo quality was evaluated before transfer. NLB, normal live birth; BMI, body mass index; FSH, follicle stimulating hormone; β-HCG, beta human chorionic gonadotropin; CoQ10, coenzyme Q10; EE, early pregnancy loss with embryos.
Karyotype analysis
Karyotype results were collected from 99 AP and 229 EE patients. The chromosomal normality rate was significantly higher in the AP group (50.5% vs. 32.3%, P=0.003), with a far greater difference observed between patients younger than 35 years old (34.3% vs. 62.1%, P<0.001). Trisomy 16 and trisomy 22 were the most common abnormalities in both groups. Viable aneuploidies were more frequently found in the EE group, including monosomy X, trisomy 21, trisomy 13, and trisomy 18. Interestingly, not a single case of monosomy X that could be detected in the AP group, which is consistent with previous reports (9,10) (Table S1).
Discussion
Main findings
To the best of our knowledge, this study is the first to demonstrate that blastocyst culture of non-top-quality embryos is associated with almost a doubling in the risk of AP when compared with cleavage stage embryo transfers. Advanced maternal age might be another risk factor, while deceased serum β-HCG level could reasonably be considered as one of the characteristics of AP due to the deficiency of chorionic villous vascularization, as previously reported (5,6). One-way ANOVA demonstrated statistical differences in female BMI, infertility duration, and infertility type between AP, EE, and NLB. However, multivariable logistic regression analysis showed that there was no obvious association between these variables and AP. Embryo cryopreservation might not increase the incidence of AP, since no statistical difference could be observed between fresh and frozen cycles.
The chromosomal abnormality rate was significantly lower in the AP group compared with the EE group, confirming data from several other recent studies (9-11). Viable aneuploidies were more frequently found in the EE group, and not a single case of monosomy X could be observed in the AP group.
The occurrence of AP is possibly due to epigenetic changes
Here this current study, patients were excluded if they had any indications of autoimmune or anatomic abnormalities, and they were routinely asked to avoid any possible pollutants or toxicants three months before and during IVF cycles to minimize environmental influences as far as possible. Under such circumstances, we observed an increase in AP when blastocyst culture was used in non-top-quality originated embryos compared with transfer of cleavage stage embryos, with a significantly lower aneuploidy rate in AP. Therefore, we speculate that non-genomic factors, such as epigenetic abnormalities, could be a potential cause of AP.
Blastocyst transfer has been previously reported to result in better implantation and live birth rate by enabling self-selection of viable embryos and improving embryo-uterine synchronicity (19,20). However, it has been well documented that epigenetic reprogramming is initiated in primordial germline cells and finishes during embryo implantation (21,22), coinciding with the time-frame of ART-related manipulations including blastocyst culture. Many studies have identified alterations in human DNA methylation resulting from ART (23-28). In vitro culture induces changes in the DNA methylation landscape of the embryo, and increased length of culture results in a greater number of epigenetic changes at the blastocyst stage (29-31). In addition, as has been reported, maternal age also influences the epigenetic modifications of the oocyte genome, which could be transmitted to the next generation (32). In a recent study using uniparental embryos, researchers found that the paternal genome is more likely to contribute to the extra-embryonic trophectoderm lineage, while the maternal component is essential for the development of the inner cell mass, and epigenetic regulation is responsible for these parent-specific expressions (33). Therefore, we hypothesized that maternal epigenetic changes, due to advanced maternal age or in vitro embryo culture, might be responsible for the formation of AP, and those non-top-quality embryos, which might have originated from impaired-quality oocytes, are possibly more susceptible to extended in vitro culture compared to top-quality embryos during the epigenetic reprogramming process. However, further research is needed to fully elucidate the pathogenesis of AP and to re-evaluate the safety of extended embryo culture in ART treatments.
Presently, preimplantation genetic testing (PGT) on extended cultures and blastocyst biopsies facilitates the optimal selection of euploid embryos. However, based on our study, embryo quality on day 3 of the relevant ovum pickup cycle should also be considered, since a non-top-quality originated euploid blastocyst might have a higher possibility of resulting in AP compared with top-quality originated embryos.
CoQ10 might be a protective factor of AP
CoQ10 is a redox-active, lipophilic substance that is present in the inner mitochondrial membrane. It functions as an electron carrier for the production of cellular energy and stabilizes the cell membrane as an antioxidant (34). CoQ10 supplementation has long been used to ameliorate infertility outcomes and is associated with improved embryo quality and increased clinical pregnancy rates (35). The data herein suggested that oral supplementation CoQ10 before oocyte retrieval might decrease the incidence of AP. This is possibly due to its antioxidative effects in the oocytes and the embryos during the in vitro embryo culture. However, further research is warranted to fully understand the mechanisms involved.
Strengths and limitations
To the best of our knowledge, this is the first report examining the association of blastocyst culture of non-top-quality cleavage embryos and the increased risk of AP. Safety of ART has always been of primary concern, and although blastocyst culture has proven successful, its safety requires continual monitoring. One potential limitation of this study is that data from a single IVF center were analyzed retrospectively, and future multi-center prospective cohort studies should be conducted to verify these results.
Conclusions
Advanced maternal age and extended culture of non-top-quality embryos may increase the risk of AP due to possible maternal epigenetic abnormalities. Embryo quality on day 3 of the relevant ovum pickup cycle should be considered when making a decision on the PGT cycle, since a non-top-quality originated euploid blastocyst might lead to an increased incidence of AP. However, CoQ10 supplementation may be a potential method for preventing AP.
Acknowledgments
Funding: This work was supported by The National Natural Science Foundation of China (81901566) and the Natural Science Foundation of Guangdong Province (2022A1515011732).
Footnote
Reporting Checklist: The authors have completed the STROBE reporting checklist. Available at https://atm.amegroups.com/article/view/10.21037/atm-22-5809/rc
Data Sharing Statement: Available at https://atm.amegroups.com/article/view/10.21037/atm-22-5809/dss
Conflicts of Interest: All authors have completed the ICMJE uniform disclosure form (available at https://atm.amegroups.com/article/view/10.21037/atm-22-5809/coif). The authors have no conflicts of interest to declare.
Ethical Statement: The authors are accountable for all aspects of the work, including ensuring that any questions related to the accuracy or integrity of any part of the work have been appropriately investigated and resolved. This study was approved by the Institutional Review Board for Nanfang Hospital (No. NFEC-2017-055). The study was conducted in accordance with the Declaration of Helsinki (as revised in 2013). Due to the retrospective nature of the study, the requirement of written informed consent was waived.
Open Access Statement: This is an Open Access article distributed in accordance with the Creative Commons Attribution-NonCommercial-NoDerivs 4.0 International License (CC BY-NC-ND 4.0), which permits the non-commercial replication and distribution of the article with the strict proviso that no changes or edits are made and the original work is properly cited (including links to both the formal publication through the relevant DOI and the license). See: https://creativecommons.org/licenses/by-nc-nd/4.0/.
References
- Jurkovic D, Overton C, Bender-Atik R. Diagnosis and management of first trimester miscarriage. BMJ 2013;346:f3676. [Crossref] [PubMed]
- Jackson T, Watkins E. Early pregnancy loss. JAAPA 2021;34:22-7. [Crossref] [PubMed]
- ChaudhryK, Tafti D, Siccardi MA.Anembryonic Pregnancy. Treasure Island (FL): StatPearls Publishing; 2022.
- Andersen LB, Dechend R, Karumanchi SA, et al. Early pregnancy angiogenic markers and spontaneous abortion: an Odense Child Cohort study. Am J Obstet Gynecol 2016;215:594.e1-594.e11. [Crossref] [PubMed]
- Aydin S, Ozeren M, Yenilmez E, et al. Morphologic alterations and immunohistochemical analysis of alpha-fetoprotein and CD34 in chorionic villi of anembryonic pregnancy. Saudi Med J 2006;27:154-60.
- Lisman BA, Boer K, Bleker OP, et al. Abnormal development of the vasculosyncytial membrane in early pregnancy failure. Fertil Steril 2004;82:654-60. [Crossref] [PubMed]
- Lathi RB, Mark SD, Westphal LM, et al. Cytogenetic testing of anembryonic pregnancies compared to embryonic missed abortions. J Assist Reprod Genet 2007;24:521-4. [Crossref] [PubMed]
- Muñoz M, Arigita M, Bennasar M, et al. Chromosomal anomaly spectrum in early pregnancy loss in relation to presence or absence of an embryonic pole. Fertil Steril 2010;94:2564-8. [Crossref] [PubMed]
- Cheng HH, Ou CY, Tsai CC, et al. Chromosome distribution of early miscarriages with present or absent embryos: female predominance. J Assist Reprod Genet 2014;31:1059-64. [Crossref] [PubMed]
- Ouyang Y, Tan Y, Yi Y, et al. Correlation between chromosomal distribution and embryonic findings on ultrasound in early pregnancy loss after IVF-embryo transfer. Hum Reprod 2016;31:2212-8. [Crossref] [PubMed]
- Romero ST, Geiersbach KB, Paxton CN, et al. Differentiation of genetic abnormalities in early pregnancy loss. Ultrasound Obstet Gynecol 2015;45:89-94. [Crossref] [PubMed]
- Yin Y, Zhang T, Dai Y, et al. The effect of plasma lead on anembryonic pregnancy. Ann N Y Acad Sci 2008;1140:184-9. [Crossref] [PubMed]
- Yin Y, Zhang T, Dai Y, et al. Pilot study of association of anembryonic pregnancy with 55 elements in the urine, and serum level of folate, homocysteine and S-adenosylhomocysteine in Shanxi Province, China. J Am Coll Nutr 2009;28:50-5. [Crossref] [PubMed]
- Hipp H, Crawford S, Kawwass JF, et al. First trimester pregnancy loss after fresh and frozen in vitro fertilization cycles. Fertil Steril 2016;105:722-8. [Crossref] [PubMed]
- Emami F, Eftekhar M, Jalaliani S. Correlation between clinical and laboratory parameters and early pregnancy loss in assisted reproductive technology cycles: A cross-sectional study. Int J Reprod Biomed 2022;20:683-90. [Crossref] [PubMed]
- Wu T, Yin B, Zhu Y, et al. Molecular cytogenetic analysis of early spontaneous abortions conceived from varying assisted reproductive technology procedures. Mol Cytogenet 2016;9:79. [Crossref] [PubMed]
- The Istanbul consensus workshop on embryo assessment: proceedings of an expert meeting. Hum Reprod 2011;26:1270-83. [Crossref] [PubMed]
- WHO. WHO laboratory manual for the Examination and processing of human semen. 5 ed.Geneva, Switzerland: WHO Press; 2010.
- Glujovsky D, Farquhar C, Quinteiro Retamar AM, et al. Cleavage stage versus blastocyst stage embryo transfer in assisted reproductive technology. Cochrane Database Syst Rev 2016;CD002118. [Crossref] [PubMed]
- Papanikolaou EG, Camus M, Kolibianakis EM, et al. In vitro fertilization with single blastocyst-stage versus single cleavage-stage embryos. N Engl J Med 2006;354:1139-46. [Crossref] [PubMed]
- Ishida M, Moore GE. The role of imprinted genes in humans. Mol Aspects Med 2013;34:826-40. [Crossref] [PubMed]
- Reik W, Dean W, Walter J. Epigenetic reprogramming in mammalian development. Science 2001;293:1089-93. [Crossref] [PubMed]
- Cox GF, Bürger J, Lip V, et al. Intracytoplasmic sperm injection may increase the risk of imprinting defects. Am J Hum Genet 2002;71:162-4. [Crossref] [PubMed]
- Maher ER, Brueton LA, Bowdin SC, et al. Beckwith-Wiedemann syndrome and assisted reproduction technology (ART). J Med Genet 2003;40:62-4. [Crossref] [PubMed]
- Ørstavik KH, Eiklid K, van der Hagen CB, et al. Another case of imprinting defect in a girl with Angelman syndrome who was conceived by intracytoplasmic semen injection. Am J Hum Genet 2003;72:218-9. [Crossref] [PubMed]
- Shi X, Chen S, Zheng H, et al. Abnormal DNA Methylation of Imprinted Loci in Human Preimplantation Embryos. Reprod Sci 2014;21:978-83. [Crossref] [PubMed]
- White CR, Denomme MM, Tekpetey FR, et al. High Frequency of Imprinted Methylation Errors in Human Preimplantation Embryos. Sci Rep 2015;5:17311. [Crossref] [PubMed]
- Whitelaw N, Bhattacharya S, Hoad G, et al. Epigenetic status in the offspring of spontaneous and assisted conception. Hum Reprod 2014;29:1452-8. [Crossref] [PubMed]
- Gad A, Besenfelder U, Rings F, et al. Effect of reproductive tract environment following controlled ovarian hyperstimulation treatment on embryo development and global transcriptome profile of blastocysts: implications for animal breeding and human assisted reproduction. Hum Reprod 2011;26:1693-707. [Crossref] [PubMed]
- Salilew-Wondim D, Fournier E, Hoelker M, et al. Genome-Wide DNA Methylation Patterns of Bovine Blastocysts Developed In Vivo from Embryos Completed Different Stages of Development In Vitro. PLoS One 2015;10:e0140467. [Crossref] [PubMed]
- Sirard MA. The influence of in vitro fertilization and embryo culture on the embryo epigenetic constituents and the possible consequences in the bovine model. J Dev Orig Health Dis 2017;8:411-7. [Crossref] [PubMed]
- Kawai K, Harada T, Ishikawa T, et al. Parental age and gene expression profiles in individual human blastocysts. Sci Rep 2018;8:2380. [Crossref] [PubMed]
- Leng L, Sun J, Huang J, et al. Single-Cell Transcriptome Analysis of Uniparental Embryos Reveals Parent-of-Origin Effects on Human Preimplantation Development. Cell Stem Cell 2019;25:697-712.e6. [Crossref] [PubMed]
- Barshop BA, Gangoiti JA. Analysis of coenzyme Q in human blood and tissues. Mitochondrion 2007;7:S89-93. [Crossref] [PubMed]
- Florou P, Anagnostis P, Theocharis P, et al. Does coenzyme Q(10) supplementation improve fertility outcomes in women undergoing assisted reproductive technology procedures? A systematic review and meta-analysis of randomized-controlled trials. J Assist Reprod Genet 2020;37:2377-87. [Crossref] [PubMed]