A literature review of the promising future of TROP2: a potential drug therapy target
Introduction
Trophoblast cell surface antigen 2 (TROP2), also known as tumor-associated calcium signal transducer 2 (TACSTD2), is a cell surface glycoprotein that acts as a transmembrane transducer of intracellular (IC) calcium signals. It is expressed in many normal tissues but is overexpressed in a variety of tumors, such as pancreatic (1), ovarian (2), prostate (3), and breast (4) cancers. TROP2 plays an important role in tumor cell proliferation, apoptosis, and invasion, thereby impacting the prognosis and treatment of cancer patients (2). The surface TROP2 expression is positively associated with E-cadherin expression and negatively with the mesenchymal gene signature in breast and prostate cancers, suggesting that it correlates with the epithelial phenotype (5). The ability of TROP2 to promote migration and invasion of cancer cells was described in several types of tumors (2). The role of TROP2 in regulating proliferation is a complex and cell type-specific phenomenon. TROP2 stimulates proliferation and cellular growth in human cervical and bladder cancer cells, while the ability of TROP2 to suppress cell proliferation was also reported in cholangiocarcinoma (CHOL) and MCF7 breast cancer cell lines (6,7). In addition, TROP2 appears to have a dual function in the regulation of cancer cell survival and drug resistance. The downregulation of TROP2 in cervical cancer cell lines increases apoptosis in ovarian carcinoma and bladder cancer cells (8,9). Contrary to these findings, cervical cancer cells overexpressing TROP2 were more sensitive to cisplatin induced apoptosis, while cells silenced expressing TROP2 were more resistant (10). TROP2 signals cells via different pathways and is transcriptionally regulated by a complex network of several molecules (11). Since TROP2 plays a critical role in the metastasis and progression of many cancers, agents that target TROP2 have potential as therapies for advanced cancers (12). In this study, based on literature obtained from medical databases, we comprehensively reviewed relevant studies on the role of TROP2 in tumorigenesis and the promising potential of TROP2 as a biomarker and emerging therapeutic target for advanced cancer. We present the following article in accordance with the Narrative Review reporting checklist (available at https://atm.amegroups.com/article/view/10.21037/atm-22-5976/rc).
Methods
A total of 1,376 literatures from PubMed and 614 literatures from EMBASE were retrieved by searching “TROP2” or “Trophoblast cell surface antigen 2”. The search was conducted on December 12, 2020, and updated on November 20, 2022 (Table 1). There were no language, publication date, or publication type restrictions. We also analyzed the expression and mutation of the TROP2 gene using data obtained from TCGA (The Cancer Genome Atlas) through the cBioportal (13) (https://www.cbioportal.org/). Also, GEPIA (Gene Expression Profiling Interactive Analysis) (http://gepia2.cancer-pku.cn/#index) was utilized to evaluate the prognostic value of TROP2 in different types of cancer (14).
Table 1
Items | Specification |
---|---|
Date of search | December 12, 2020 to November 20, 2022 |
Databases and other sources searched | PubMed, EMBASE, cBioportal and GEPIA |
Search terms used | TROP2, Trophoblast cell surface antigen 2 |
Timeframe | From 1980 to 2022 |
Inclusion and exclusion criteria | Inclusion criteria: papers involved the expression, functions, interactions, prognostic values and clinical applications of TROP2 |
Exclusion criteria: non-English language papers | |
Selection process | The selection was conducted by 3 authors independently and was discussed with other 2 authors in the case of any disagreements |
Discussion
TROP2 expression and mutation in cancers
TROP2 was overexpressed in a variety of (but not all) malignant tumors and exhibits differential expression in certain normal tissues (15,16). Patients with bladder urothelial carcinoma (BLCA) expressed the highest level of the TROP2 gene, followed by those with head and neck squamous cell carcinoma (HNSC) and lung squamous cell carcinoma (LUSC) (Figure 1). TROP2 was upregulated in BLCA, CHOL, colon adenocarcinoma (COAD), esophageal carcinoma (ESCA), lung adenocarcinoma (LUAD), LUSC, prostate adenocarcinoma (PRAD), rectum adenocarcinoma (READ), stomach adenocarcinoma (STAD), thyroid carcinoma (THCA), and uterine corpus endometrial carcinoma (UCEC), and downregulated in HNSC, kidney chromophobe (KICH), kidney renal clear cell carcinoma (KIRC), kidney renal papillary cell carcinoma (KIRP), and liver hepatocellular carcinoma (LIHC). The mutation rate of TROP2 gene was different in various tumors. Sarcoma has the highest rate of TROP2 gene mutation, with most of the mutation types being amplified, followed by miscellaneous neuroepithelial and ovarian epithelial tumors (Figure 2). Moreover, congenital mutations in human TROP2 can cause gelatinous drop-like corneal dystrophy (GDLD), which is a rare autosomal recessive genetic disease that can lead to the development of bilateral corneal amyloidosis and eventually blindness (17). Although we observed the presence of TROP2 mutations in some tumors, subsequent studies are needed to further confirm whether TROP2 mutations are directly or indirectly associated with cancer. Therefore, clinical studies targeting TROP2 mutations have not been implemented. Current known reports favor the overexpression of TROP2 in most solid tumor cancers, promoting tumorigenesis and progress of cancer (12).
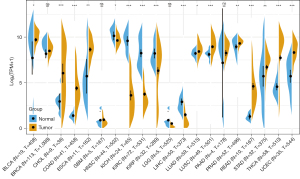
TROP2 structural features
TROP2 is human trophoblast cell surface glycoprotein antigen 2 that belongs to the TACSTD family (18) and is also known as TACSTD2, epidermal glycoprotein 1 (EGP-1), and membrane component chromosome 1 surface marker 1 (M1S1) or gastric antigen 733-1 (GA733-1) (19-21). It is a type I transmembrane cell-surface glycoprotein originally identified in human placental trophoblasts and subsequently found to be highly expressed in most human carcinomas (22). The intronless TROP2 gene is found on the short arm of human chromosome 1 and is located at 1p32.1. It is a type I cell membrane glycoprotein formed by N-terminal glycosylation and posttranslational modification that is composed of 323 amino acids, known as the TROP2 protein, and is approximately 36 kD in size (23). TROP2 is composed of a hydrophobic leader peptide (AA1–26), an extracellular domain (ECD) (AA27–274), a transmembrane domain (AA275–297), and a cytoplasmic tail (AA298–323) (21) (Figure 3).
TROP2 connects the N-terminal ectodomain (EC) to the IC hydrophobic polypeptide short tail through a unidirectional transmembrane helix (TM), thereby immobilizing it on the cell membrane (12). There are highly conserved phosphatidylinositol 4,5-biphosphate (PIP2) binding sequences and tyrosine and serine phosphorylation sites in the cytoplasmic tail, indicating that PIP2 has an important impact on the signal transduction of TROP2. The mutation of serine residue 303 inhibits the ability of TROP2 to stimulate tumor growth. Protein kinase C (PKC) is responsible for its phosphorylation; the phosphorylation of TROP2 IC induces the reorganization of the salt bridge, which causes conformational alteration of the TROP2 functional area. These structural features may have important significance in regulating the activity of TROP2 (12,20,24,25).
TROP2 transcriptional regulation
Compared with non-cancer cells, the reason why TROP2 is overexpressed in many cancers is not fully understood (24). A possible explanation might be the stem cell-like characteristics of TROP2, which exert an inherent regulatory effect on cell growth, proliferation, regeneration, and transformation (26). TROP2 overexpression has been shown to be necessary and sufficient to drive cancer growth (24), and therefore, will be more representative due to the proliferation of cancer cells (12). Another possible reason is the network of transcription factors that modulate TROP2 expression. TROP2 overexpression in cancer does not arise from structural alterations of the gene itself but rather due to deregulation at the transcriptional and post-transcriptional levels (20). This network includes tumor protein 63 (TP63)/tumor protein 53L (TP53L), Wilms tumor 1 (WT1), ETS-related gene (ERG), T-cell factor (TCF-1)/hepatocyte nuclear factor 1 (HNF1A)/lymphoid enhancer factor (LEF1), autoimmune regulator (AIRE), Glis2, forkhead box protein transcription factor (FOXM1), FOXP3, spleen focus forming virus (SFFV) proviral integration oncogene (SPI1/PU.1), and so on (24,27).
TROP2 signal transduction and function
As a new glycoprotein receptor on the cell membrane surface, TROP2 mainly promotes tumor cell growth, proliferation, and metastasis by regulating the calcium ion signaling pathway and cyclin expression and reducing fibronectin adhesion (27). Figure 4 illustrates the IC signaling network mediated by TROP2 and its involvement in tumorigenesis and development.
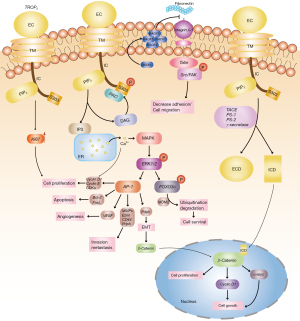
Ca2+ can also further stimulate mitogen-activated protein kinase (MAPK) signal transduction, which increases the levels of phosphorylated ERK1 and ERK2. ERK signal transduction leads to an increase in the transcription factor AP-1, which is a central regulator of tumor-associated target genes during tumorigenesis. AP-1 induces angiogenesis by vascular endothelial growth factor (VEGF); cell proliferation by cyclins and CDKs; apoptosis by Bcl-2 (B-cell lymphoma 2) or FasL (Fas ligand); cell invasion and metastasis by MMPs (matrix metalloproteinases), Pdon (podoplanin), Ezrin, and CD44; and EMT (epithelial to mesenchymal transition) by Pdpn. EMT allows more β-catenin to enter the nucleus and promote cell growth. Enhancement of ERK activity induces phosphorylation of FOXO3a, leading to ubiquitination of MDM2 (mouse double minute 2) and proteasomal degradation. The degradation of FOXO3a may help to promote cancer cell survival. TROP2 increases the expression of Ki-67 (a cell proliferation marker) and further activates cell proliferation.
TROP2 is cleaved into the ECD and intracellular domain (ICD) by the tumor necrosis factor alpha (TNF-α) converting enzyme (TACE), followed by γ-secretase. The cleavage is mediated by two dominant enzymes, presenilin 1 (PS-1) and presenilin 2 (PS-2).
RACK1 (cytoplasmic protein kinase C receptor 1) is enriched on the cell membrane by TROP2, inhibiting the binding of fibronectin to integrin β-1. TROP2 reduces tumor cell adhesion and promotes metastasis through the integrin β-1-RACK1-Src/FAK signal transmission axis.
TROP2 mediates cell cycle progression through the calcium ion signaling pathway
Under the action of PKC, the IC tail serine residue (S303) of the TROP2 protein is phosphorylated, thereby promoting PIP2 hydrolysis for inositol triphosphate (IP3) and diacylglycerol (DAG). IP3 interacts with the IP3 receptor on the endoplasmic reticulum surface and promotes the release of calcium ions stored in the endoplasmic reticulum, activates the MAPK pathway, and promotes the cell cycle process (an increased percentage of cells enter the S phase). Moreover, mTROP2 increases the level of phosphorylated MAPK (ERK1/ERK2), while enhanced ERK activity induces the phosphorylation of FOXO3a at residues S294, S344, and S425. This phenomenon may lead to its ubiquitination by MDM2, which in turn promotes the cytoplasmic localization of FOXO3a and proteasome degradation, thereby contributing to tumor cell survival (24,27,28). The increased concentration of IC calcium may affect cell signal activation and cell cycle progression by activating PKC and/or calcium/calmodulin-dependent protein kinase II (CaMKII). Consequently, the percentage of cells entering the DNA synthesis stage is increased. Moreover, DAG regulates the phosphorylation of TROP2 by activating PKC reciprocally (29).
TROP2 regulates the cell cycle via IC hydrolysis
Under the combined action of the TACE, γ-secretase, and PS-1/2, TROP2 is cleaved into two products, namely, the ECD and the ICD. ICD is released from the membrane and enters the nucleus (although some are found on the membrane). It colocalizes with β-catenin in the Wnt signaling cascade in the nucleus and upregulates the expressions of cyclin D1 and a proto-oncogene (c-myc), thereby playing a role in the transcription of nuclear oncogenes and cell proliferation (30).
TROP2-mediated apoptosis and proliferation signals
TROP2 activates the phosphorylation of p42/p44MAPK (ERK1/2) and further enhances the activity of the downstream transcription factor AP-1, which is the central regulator of tumor-related target genes in the process of carcinogenesis (28). AP-1 induces angiogenesis through VEGF, apoptosis through the pro-apoptotic Bcl-2 or FasL, acceleration of the cell cycle through cyclinD1/cyclinE and CDK, and cell invasion through MMPs, Pdpn, Ezrin, and CD44.
Furthermore, AP-1 can also cause epithelial-to-mesenchymal transition (EMT) via Pdpn; EMT allows for the nuclear translocation of β-catenin, which is conducive to cell proliferation. TROP2 can promote the proliferation of tumor cells by upregulating the expression of the proliferation marker Ki-67, whereas the proliferation of tumor cells is disturbed when the TROP2 gene is knocked down (29,31).
TROP2 promotes tumor invasion and metastasis
TROP2 enriches the RACK1 on the cell membrane, which reduces the binding of fibronectin to integrin β-1. TROP2 forms a complex with integrin β-1 and talin proteins, resulting in the activation of downstream Src and FAK. TROP2 reduces tumor cell adhesion and promotes metastasis through the integrin β-1-RACK1-Src/FAK signal transmission axis (32).
TROP2 as a prognostic biomarker
Through the GEPIA database, we found that a high level of TROP2 is potentially related to better overall survival (OS) in lymphoid neoplasm diffuse large B-cell lymphoma (DLBC), KICH, KIRP, and acute myeloid leukemia (LAML), while a low level of TROP2 is potentially related to better OS in LUAD, ovarian serous cystadenocarcinoma (OV), pancreatic adenocarcinoma (PAAD), and skin cutaneous melanoma (SKCM). Furthermore, high levels of TROP2 are associated with better disease-free survival (DFS) in KICH, KIRP and UCEC, while low levels of TROP2 are associated with better DFS in OV, PAAD, and READ (Figure 5). The obvious correlation between TROP2 levels and cancer aggressiveness and recurrence risk suggests its utility as an independent prognostic indicator for patients (33).
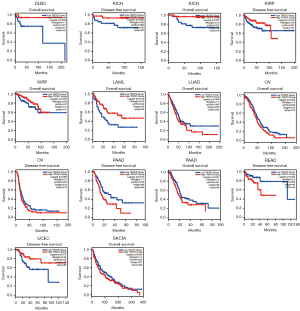
The expression of TROP2 can be used as a unique prognostic biomarker of lymph node metastasis, degree of differentiation, and tumor size (34). TROP2 overexpression is also increased in HNSC and is related to the degree of tissue differentiation and lymph node metastasis (35,36). High expression of TROP2 protein is associated with high aggressiveness in ovarian cancer (37). Elevated levels of TROP2 are also correlated with poor patient outcomes and a more aggressive clinical course in prostate cancer (38,39). In general, TROP2 overexpression often correlates with an unfavorable prognosis and increased risk of metastasis (1,11,40,41), while downregulation is correlated with poor prognosis in some types of cancer (42). Importantly, the hypermethylation of the TACSTD2 gene promoter explains the low expression of TROP2 in this type of cancer (43). Nevertheless, the prognostic value of TROP2 may also depend on its cellular localization within tumors, which requires further research (44).
TROP2 in cancer therapy
As a transmembrane protein whose ECD is overexpressed in a variety of tumors, TROP2 seems to be an exceptionally promising candidate for immunotherapeutic strategies (45). Various forms of drugs have been rapidly developed, including monoclonal antibodies (mAbs), bispecific antibodies, antibody-drug conjugates (ADCs), virus-like particles (VLPs), as well as antibody drugs combined with traditional chemotherapy, immunotherapy, radioimmunotherapy, photoimmunotherapy, and nanoparticles that target TROP2 (46-49).
mAbs
Anti-TROP2 mAbs with high affinity can recognize different regions of the TROP2 molecule and can be used in the treatment and diagnosis of various types of cancer. hRS7 is a humanized IgG1 mAb directed against TROP2, which was originally raised against human non-small cell carcinoma of the lung (50) and is reactive with several cancers. For example, unlike TROP2-negative endometrial endometrioid carcinoma (EEC) cell lines, the EEC primary cell line was found to be highly sensitive to hRS7-mediated antibody-dependent cellular cytotoxicity in vitro (47). TROP2-positive cell lines have also exhibited high sensitivity to hRS7 antibody-dependent cell-mediated cytotoxicity (ADCC) in carcinomas of the uterus, cervix, and ovary (46,51-53). The hRS7 antibody internalizes when bound to TROP2, which enables direct intracellular delivery of cytotoxics (54). In the absence of hRS7 or the presence of rituximab control antibodies, the cytotoxicity of chemotherapy-resistant ovarian cancer is negligible (51).
Bispecific antibodies
TF12 is a trivalent bispecific antibody that consists of two anti-TROP-2 Fab fragments and one antihistamine-succinyl-glycine (HSG) Fab fragment (55). The characteristics and potential for pre-targeted radioimmunoimaging and radioimmunotherapy with TF12 and 111In-IMP288, a radiolabeled hapten-peptide, in mice with human prostate cancer have been investigated. TROP2-expressing prostate cancer can be pre-targeted efficiently with TF12, with a very rapid uptake of 111In-IMP288, sensitive immuno-positron emission tomography (immunoPET), and effective therapy (56). Furthermore, TF12 is sufficiently preserved on the cell surfaces of several epithelial cancers, making it suitable for pre-targeted imaging and treatment of various TROP-2-expressing cancers (57).
(E1)-3s is a T-cell-redirecting trivalent bispecific antibody comprising an anti-CD3 scFv covalently linked to a stabilized dimer of a TROP-2-targeting Fab using Dock-and-Lock (DNL). This covalent combination was designated (X)-3s using the DNL method, in which the code “(X)” denotes a stabilized tumor-associated antigen (TAA)-specific Fab dimer that is fused site-specifically to an scFv of Okt3, indicated as “3s” (58). Bispecific antibody-mediated bidirectional phagocytosis occurs between target cells and T cells and involves immune synapses (59). (E1)-3s effectively induces T-cell-mediated killing of TROP2-expressing pancreatic and gastric cancers (60), validating TROP2 as a candidate tumor-associated antigen for cancer therapy.
Human Fab antibody
The ECD of TROP2 was chosen as the antigen to isolate a human naive Fab antibody from the phage library using phage display technology. TROP2 Fab inhibits proliferation, induces apoptosis, and terminates the migration of MDA-MB-231 cells in a concentration-dependent manner (45). Compared with the control group, Bcl-2 expression was significantly downregulated, while Bax expression was significantly upregulated after treatment with TROP2 Fab in nude mice. Therefore, TROP2 Fab represents a promising chemotherapeutic agent for TROP2-expressing breast cancer (45,61).
ADCs
The utility of anti-TROP2 antibodies coupled with other chemotherapeutic drugs has been confirmed in various preclinical studies. Based on the previous TROP2-Fab antibody, a new TROP2-IgG antibody was constructed via the eukaryotic expression system and then coupled with an SN-38, such as hRS7-CL2A-SN-38, which has been shown to exert significant specific anticancer effects in a variety of tumor cell lines (45). SN-38, the active metabolite of the topoisomerase inhibitor irinotecan, has a derivative, CL2A, which has been successfully conjugated to hRS7.
Sacituzumab govitecan (IMMU-132), hereinafter referred to as sacituzumab, is coupled with the humanized antibody hRS7 as the carrier of TROP2 targeting with SN-38 (27). The United States Food and Drug Administration (FDA) approved the application of sacituzumab on December 26, 2019, for triple-negative breast cancer (TNBC) patients who have received two or more prior therapies (NCT01631552) (4,62-64). Sacituzumab is currently undergoing various clinical trials for different malignancies, including metastatic breast cancer, urothelial cancers, gastric cancer, non-small cell lung cancer, and castration-resistant prostate cancer (44,49,64-68) (Table 2). In April 2022, the Chinese Society of Clinical Oncology Breast Cancer (CSCO BC) guidelines recommended that sacituzumab be included in the advanced rescue treatment of TNBC. The treatment of tumor-bearing mice with hRS7-SN-38 (either with CL2-SN-38 or CL2A-SN-38) significantly inhibited tumor growth in five different tumor models. It is well tolerated in monkeys and worthy of further study in human clinical trials (68). In fact, a smaller dose of SN-38 administered by sacituzumab govitecan is considerably more effective than a larger dose of irinotecan or a combination of hRS7 IgG and SN-38 (68).
Table 2
Clinical Trials | Cancers | Phase | Status |
---|---|---|---|
NCT01631552 | Refractory metastatic epithelial cancers | I & II | Completed |
NCT02574455 | Refractory/Relapsed metastatic TNBC | III | Completed |
NCT03337698 | Metastatic NSCLC | I & II | Recruiting |
NCT03424005 | Metastatic or advanced TNBC | I & II | Active, not recruiting |
NCT03547973 | Metastatic urothelial carcinoma | II | Recruiting |
NCT03725761 | Metastatic castration-resistant prostate cancer | II | Recruiting |
NCT03901339 | HR+/HER2− metastatic breast cancer | III | Active, not recruiting |
NCT03964727 | Metastatic NSCLC; head and neck Squamous cell carcinoma; endometrial cancer | II | Recruiting |
NCT03992131 | Ovarian cancer; TNBC; urothelial carcinoma; solid tumor | Ib & II | Terminated |
NCT03995706 | Glioblastoma; metastatic brain tumors | I | Recruiting |
NCT04039230 | Metastatic breast cancer | I & II | Recruiting |
NCT04230109 | Invasive localized TNBC | II | Active, not recruiting |
NCT04251416 | Endometrial carcinoma | II | Recruiting |
NCT04319198 | Metastatic solid tumor | IV | Enrolling by invitation |
NCT04448886 | Metastatic HR+/HER2− breast cancer | II | Recruiting |
NCT04454437 | Metastatic TNBC | II | Active, not recruiting |
NCT04468061 | PD-L1-negative metastatic TNBC | II | Recruiting |
NCT04527991 | Locally advanced or metastatic unresectable urothelial cancer | III | Recruiting |
NCT04559230 | Glioblastoma | II | Recruiting |
TNBC, triple-negative breast cancer; NSCLC, non-small cell lung cancer; HR+, hormone receptor-positive; HER2−, human epidermal growth factor-2 negative; PD-L1, programmed death-ligand 1.
A site-specific TROP2-ADC, RN927C (also known as PF-06664178) is composed of a humanized anti-TROP2 hIgG1 antibody conjugated with a cleavable proprietary microtubule inhibitor (MTI) linker payload, PF-06380101 (22). Upon binding to the extracellular portion of TROP2 on the cell surface, RN927C is internalized and transported to the lysosome, where PF-06380101 is released and induces mitotic arrest, followed by cell death (22). Indeed, RN927C has shown a potent cell-killing effect in a variety of tumor cell lines and patient-derived xenograft tumor models, including pancreatic cancer and TNBC (22).
Datopotamab deruxtecan (Dato-DXd) is another TROP2-directed ADC with a potent DNA topoisomerase I inhibitor (DXd) (3). The topoisomerase I inhibitor is linked to the TROP2 mAb by a tetrapeptide-based linker (69). Dato-DXd is bound specifically to TROP2 and is internalized into tumor cells, where lysosomal enzymes break the linker to release DXd, which induces DNA damage and apoptosis in TROP2-expressing tumor cells. The DNA damage of TROP2-expressing xenograft tumors induced by accumulated DXd is unique, and neither the isotype control IgG-ADC nor the anti-TROP2 antibody displayed this effect (70). Dato-DXd is currently being investigated in a phase I clinical trial in patients with TNBC (NCT03401385) and will be a valuable treatment option for patients with TROP2-expressing tumors (3).
Ranpirnase (Rap) is an amphibian RNase with antitumor activity, minimal toxicity, and negligible immunogenicity (71). A Rap fusion protein constructed through hRS7 has the potential to inhibit chronic lymphocytic leukemia and Raji Burkitt lymphomas (72). The DNL method has been applied to construct a class of novel IgG-Rap immunoRNases, named (Rap)2-E1-(Rap)2 or 2L-Rap(Q)-hRS7, which are made of Rap(Q), a mutant Rap with the putative N-glycosylation site removed, and the anti-TROP2 antibody hRS7 (73). (Rap)2-E1-(Rap)2 has made considerable progress in enhancing the potency of Rap, which significantly suppressed tumor growth in nude mice bearing Calu-3 human non-small cell lung cancer xenografts and improved the survival rate of tumor-bearing mice (71,73).
Combination therapies with ADCs and various compounds
A previous study revealed that the overexpression of one or more efflux pumps in the superfamily of ATP-binding cassettes (ABCs) may induce drug resistance in cancer cells (74). Since SN-38 is susceptible to multiple ABC transporters (75), some patients with metastatic triple-negative breast cancer (mTNBC) showed early disease progression, thus failing IMMU-132. Treatment with known ABCG2 inhibitors (such as fumitremorgin C, Ko143, and YHO-13351) restored the toxicity of SN-38, while the combination of YHO-13351 and IMU-132 improved the therapeutic efficacy and median survival of mice bearing human gastric cancer xenografts (76). ABCG2 plays a key role in inducing SN-38 resistance in various cancer cells, and disturbances in DNA topoisomerase I (Top1) are a potential source of cellular SN-38 resistance (76).
The mutation and degradation of Top1 is the main molecular mechanism of Top1 resistance to camptothecin, especially for SN-38 (77,78). Interestingly, the poly ADP-ribose polymerase (PARP) enzyme was found to catalyze binding between the ADP-ribose polymer and Top1, which reduced the efficacy of camptothecin (79). The combination treatment of sacituzumab govitecan and PARP inhibitors (olaparib, rucaparib, or talazoparib) showed synergistic growth inhibition and antitumor effects in mice bearing human TNBC tumor xenografts (80). A phase I/II clinical trial (NCT04039230) involving patients with mTNBC is currently ongoing.
Immunotherapy, radioimmunotherapy, and photoimmunotherapy
The adoptive transfer of antigen-specific cytotoxic T lymphocytes (CTLs) is a promising anticancer immunotherapy (81). TROP2 has been verified as a potential target molecule for presentation to human CTLs, and TROP2-specific CTLs exhibit highly specific cytotoxicity to transfected target cells (82).
Radioimmunotherapy (RAIT) is a therapeutic modality that is based on selectively targeting ionizing radiation to tumor sites using tumor-targeting mAbs tagged with radionuclides (83). 131I-IMP-R4-hRS7 is an hRS7 monoclonal antibody labeled with radioactive iodine, and 131I-IMP-R4 was evaluated for preclinical RAIT in breast cancer (84,85). 131I-IMP-R4 is an improved residual form of 131I, which has the advantage of a longer residence time in tumors compared to conventional radioiodinated mAbs (86). Radiolabeled hRS7 has been demonstrated to have a tumor-targeting ability and significant antitumor efficacy in animal models. Compared to direct conventional 131I-hRS7, 131I-IMP-R4-hRS7 exhibited considerably better tumor growth control in athymic mice bearing human breast cancer xenografts (86), which provides a significant therapeutic advantage. RAIT has been combined with the humanized antibody 90Y-hPAM4 targeted antigen in pancreatic cancer, while the conjugate hRS7-SN-38 has been shown to target TROP2 in cancer cells (72). This combination therapy was found to be significantly more effective than using RAIT alone (72). In addition, radiolabeled 111In-HRS7 and 89Zr-HRS7 preferentially and specifically accumulated in prostate cancer xenografts and were clearly visualized through immunoPET and immunoSPECT; thus, they will be beneficial for guiding clinical cancer therapy (87).
Photoimmunotherapy (PIT) is a new type of tumor-specific treatment utilizing mAb-photosensitizer conjugates and near-infrared (NIR) light irradiation (88). A newly developed human anti-TROP2 mAb conjugated with photosensitizer IR700 (TROP2-IR700) has been successfully used to treat pancreatic cancer and cholangiocarcinoma cells under near-infrared irradiation (89). TROP2-IR700 could cause rapid and selective cell death when exposed to NIR light. TROP2-targeted PIT significantly inhibited the growth of xenograft tumors in pancreatic cancer and cholangiocarcinoma and is expected to be an attractive cancer-specific treatment (89).
VLPs
VLPs are highly immunogenic and versatile immunostimulants that can be used as cancer vaccines by binding exogenous proteins to their cell membranes (90). VLPs can induce the production of mTROP2-specific cytotoxic T lymphocytes and antibodies without the induction of autoimmunity. The combination of VLP immunization with gemcitabine treatment has shown an improved effect, significantly increasing the survival of tumor-bearing mice. Chimeric TROP2 VLPs will be a novel immunotherapeutic approach that could potentially be used as an alternative treatment option (90).
Anti-TROP2 antigen-conjugated nanoparticles (ST-NPs)
TROP2-targeted therapy also includes nanoparticles bound to anti-TROP2 antibodies. ST-NPs are potential nanocarriers consisting of carboxymethyl glucan (CMD) derivatives and bioreducible disulfide bonds loaded with doxorubicin (DOX), called DOX-ST-NPs, which are utilized for the targeted delivery of anticancer drugs to TNBC (91). After binding to TROP2, the nanoparticles enter cells via endocytosis and release DOX. Compared to the control nanoparticles, which lack disulfide bonds or anti-TROP2 antibodies, TROP2-expressing TNBC cell lines have higher selectivity and toxicity to DOX-ST-NPs. Therefore, ST-NPs might be a promising nanocarrier for targeted TNBC therapy (91).
Furthermore, the delivery of apoptotic activator 2 nanoparticles via liposomes targeting the TROP2 antigen is a possible approach for the intelligent killing of human gastric adenocarcinoma cells (AGS) (92). AGS cells have been treated with apoptosis activator 2-loaded liposomes that targeted the cell surface TROP2 antigen in cancer cells and significantly increased cell apoptosis (92).
Chimeric antigen receptor T (CAR-T) cell therapy
CAR-T cell therapy, a type of adoptive cell therapy, has been successfully used when treating lymphoma malignancies, but not nearly as successful in treating solid tumors. One study showed that a novel bispecific TROP2/PD-L1 CAR-T cell can target TROP2/PD-L1 and show a killing effect on gastric cancer, thus improving the killing effect of CAR-T cells in solid tumors (93). The CD27-based TROP2 CAR-T cells showed a higher antitumor activity in mouse tumor models (94). There is no denying that TROP2 is a promising target for CAR-T cell therapy. We are still waiting for further clinical trials, TROP2-CAR-T therapy is just around the corner (95).
Conclusions
In recent years, research has been conducted to identify cancer-specific proteins that can be used for targeted therapy (96-100). The TROP2 oncogene represents an ideal target for the development of ADC drugs. In this review, we found that TROP2 is highly expressed in a variety of malignant tumors and plays roles in cell proliferation, adhesion, migration, EMT, apoptosis, and targeted therapy by interacting with or binding to several molecules. TROP2 is involved in survival signaling and is a prognostic predictor in patients with several types of cancer. Briefly, TROP2 can be used as a new target for tumor-targeted therapies and has considerable research value and development prospects for research into new antitumor drugs. The development of novel TROP2-targeting therapies for advanced cancers that do not pose a risk of toxicity to normal tissues is essential. Combination therapies, such as agents targeting TROP2 coupled with conventional chemotherapy, immunotherapy, radioimmunotherapy, and nanoparticles, have substantial potential and are worthy of further study in preclinical and clinical studies.
Acknowledgments
Funding: This work was supported by grants from the National Natural Science Foundation of China (No. 81873640), the Science and Technology Innovation Program of Hunan Province (No. 2021SK2026) and the Fundamental Research Funds for the Central Universities of Central South University (Grant No. 202ZTS0950).
Footnote
Reporting Checklist: The authors have completed the Narrative Review reporting checklist. Available at https://atm.amegroups.com/article/view/10.21037/atm-22-5976/rc
Conflicts of Interest: All authors have completed the ICMJE uniform disclosure form (available at https://atm.amegroups.com/article/view/10.21037/atm-22-5976/coif). All authors report that this work was supported by grants from the National Natural Science Foundation of China (No. 81873640), the Science and Technology Innovation Program of Hunan Province (No. 2021SK2026) and the Fundamental Research Funds for the Central Universities of Central South University (Grant No. 202ZTS0950). The authors have no other conflicts of interest to declare.
Ethical Statement: The authors are accountable for all aspects of the work in ensuring that questions related to the accuracy or integrity of any part of the work are appropriately investigated and resolved.
Open Access Statement: This is an Open Access article distributed in accordance with the Creative Commons Attribution-NonCommercial-NoDerivs 4.0 International License (CC BY-NC-ND 4.0), which permits the non-commercial replication and distribution of the article with the strict proviso that no changes or edits are made and the original work is properly cited (including links to both the formal publication through the relevant DOI and the license). See: https://creativecommons.org/licenses/by-nc-nd/4.0/.
References
- Fong D, Moser P, Krammel C, et al. High expression of TROP2 correlates with poor prognosis in pancreatic cancer. Br J Cancer 2008;99:1290-5. [Crossref] [PubMed]
- Wu B, Yu C, Zhou B, et al. Overexpression of TROP2 promotes proliferation and invasion of ovarian cancer cells. Exp Ther Med 2017;14:1947-52. [Crossref] [PubMed]
- Shen M, Liu S, Stoyanova T. The role of Trop2 in prostate cancer: an oncogene, biomarker, and therapeutic target. Am J Clin Exp Urol 2021;9:73-87.
- Bardia A, Mayer IA, Vahdat LT, et al. Sacituzumab Govitecan-hziy in Refractory Metastatic Triple-Negative Breast Cancer. N Engl J Med 2019;380:741-51. [Crossref] [PubMed]
- Remšík J, Binó L, Kahounová Z, et al. Trop-2 plasticity is controlled by epithelial-to-mesenchymal transition. Carcinogenesis 2018;39:1411-8. [Crossref] [PubMed]
- Sawanyawisuth K, Tantapotinan N, Wongkham C, et al. Suppression of trophoblast cell surface antigen 2 enhances proliferation and migration in liver fluke-associated cholangiocarcinoma. Ann Hepatol 2016;15:71-81. [Crossref] [PubMed]
- Zimmers SM, Browne EP, Williams KE, et al. TROP2 methylation and expression in tamoxifen-resistant breast cancer. Cancer Cell Int 2018;18:94. [Crossref] [PubMed]
- Liu M B, Shi X F, Liu K, et al. The role and mechanism of human trophoblastic cell surface antigen 2 in the invasion and metastasis of ovarian cancer. Zhonghua Zhong Liu Za Zhi 2021;43:299-305. [Crossref] [PubMed]
- Zhang L, Yang G, Zhang R, et al. Curcumin inhibits cell proliferation and motility via suppression of TROP2 in bladder cancer cells. Int J Oncol 2018;53:515-26. [Crossref] [PubMed]
- Sin STK, Li Y, Liu M, et al. TROP-2 exhibits tumor suppressive functions in cervical cancer by dual inhibition of IGF-1R and ALK signaling. Gynecol Oncol 2019;152:185-93. [Crossref] [PubMed]
- Zhao W, Kuai X, Zhou X, et al. Trop2 is a potential biomarker for the promotion of EMT in human breast cancer. Oncol Rep 2018;40:759-66. [Crossref] [PubMed]
- Shvartsur A, Bonavida B. Trop2 and its overexpression in cancers: regulation and clinical/therapeutic implications. Genes Cancer 2015;6:84-105. [Crossref] [PubMed]
- Ustjanzew A, Desuki A, Ritzel C, et al. cbpManager: a web application to streamline the integration of clinical and genomic data in cBioPortal to support the Molecular Tumor Board. BMC Med Inform Decis Mak 2021;21:358. [Crossref] [PubMed]
- Tang Z, Li C, Kang B, et al. GEPIA: a web server for cancer and normal gene expression profiling and interactive analyses. Nucleic Acids Res 2017;45:W98-W102. [Crossref] [PubMed]
- Stepan LP, Trueblood ES, Hale K, et al. Expression of Trop2 cell surface glycoprotein in normal and tumor tissues: potential implications as a cancer therapeutic target. J Histochem Cytochem 2011;59:701-10. [Crossref] [PubMed]
- Wang J, Day R, Dong Y, et al. Identification of Trop-2 as an oncogene and an attractive therapeutic target in colon cancers. Mol Cancer Ther 2008;7:280-5. [Crossref] [PubMed]
- Tsujikawa M, Kurahashi H, Tanaka T, et al. Identification of the gene responsible for gelatinous drop-like corneal dystrophy. Nat Genet 1999;21:420-3. [Crossref] [PubMed]
- Calabrese G, Crescenzi C, Morizio E, et al. Assignment of TACSTD1 (alias TROP1, M4S1) to human chromosome 2p21 and refinement of mapping of TACSTD2 (alias TROP2, M1S1) to human chromosome 1p32 by in situ hybridization. Cytogenet Cell Genet 2001;92:164-5. [Crossref] [PubMed]
- Li X, Teng S, Zhang Y, et al. TROP2 promotes proliferation, migration and metastasis of gallbladder cancer cells by regulating PI3K/AKT pathway and inducing EMT. Oncotarget 2017;8:47052-63. [Crossref] [PubMed]
- Lenárt S, Lenárt P, Šmarda J, et al. Trop2: Jack of All Trades, Master of None. Cancers (Basel) 2020.
- Cubas R, Li M, Chen C, et al. Trop2: a possible therapeutic target for late stage epithelial carcinomas. Biochim Biophys Acta 2009;1796:309-14. [Crossref] [PubMed]
- Strop P, Tran TT, Dorywalska M, et al. RN927C, a Site-Specific Trop-2 Antibody-Drug Conjugate (ADC) with Enhanced Stability, Is Highly Efficacious in Preclinical Solid Tumor Models. Mol Cancer Ther 2016;15:2698-708. [Crossref] [PubMed]
- Ciccarelli FD, Acciarito A, Alberti S. Large and diverse numbers of human diseases with HIKE mutations. Hum Mol Genet 2000;9:1001-7. [Crossref] [PubMed]
- Zaman S, Jadid H, Denson AC, et al. Targeting Trop-2 in solid tumors: future prospects. Onco Targets Ther 2019;12:1781-90. [Crossref] [PubMed]
- Goldstein AS, Lawson DA, Cheng D, et al. Trop2 identifies a subpopulation of murine and human prostate basal cells with stem cell characteristics. Proc Natl Acad Sci U S A 2008;105:20882-7. [Crossref] [PubMed]
- Vidmar T, Pavšič M, Lenarčič B. Biochemical and preliminary X-ray characterization of the tumor-associated calcium signal transducer 2 (Trop2) ectodomain. Protein Expr Purif 2013;91:69-76. [Crossref] [PubMed]
- Guerra E, Trerotola M, Aloisi AL, et al. The Trop-2 signalling network in cancer growth. Oncogene 2013;32:1594-600. [Crossref] [PubMed]
- Cubas R, Zhang S, Li M, et al. Trop2 expression contributes to tumor pathogenesis by activating the ERK MAPK pathway. Mol Cancer 2010;9:253. [Crossref] [PubMed]
- Liu T, Liu Y, Bao X, et al. Overexpression of TROP2 predicts poor prognosis of patients with cervical cancer and promotes the proliferation and invasion of cervical cancer cells by regulating ERK signaling pathway. PLoS One 2013;8:e75864. [Crossref] [PubMed]
- Stoyanova T, Goldstein AS, Cai H, et al. Regulated proteolysis of Trop2 drives epithelial hyperplasia and stem cell self-renewal via β-catenin signaling. Genes Dev 2012;26:2271-85. [Crossref] [PubMed]
- Trerotola M, Cantanelli P, Guerra E, et al. Upregulation of Trop-2 quantitatively stimulates human cancer growth. Oncogene 2013;32:222-33. [Crossref] [PubMed]
- Trerotola M, Li J, Alberti S, et al. Trop-2 inhibits prostate cancer cell adhesion to fibronectin through the β1 integrin-RACK1 axis. J Cell Physiol 2012;227:3670-7. [Crossref] [PubMed]
- Goldenberg DM, Stein R, Sharkey RM. The emergence of trophoblast cell-surface antigen 2 (TROP-2) as a novel cancer target. Oncotarget 2018;9:28989-9006. [Crossref] [PubMed]
- Wu H, Xu H, Zhang S, et al. Potential therapeutic target and independent prognostic marker of TROP2 in laryngeal squamous cell carcinoma. Head Neck 2013;35:1373-8. [Crossref] [PubMed]
- Redlich N, Robinson AM, Nickel KP, et al. Anti-Trop2 blockade enhances the therapeutic efficacy of ErbB3 inhibition in head and neck squamous cell carcinoma. Cell Death Dis 2018;9:5. [Crossref] [PubMed]
- Tang G, Tang Q, Jia L, et al. High expression of TROP2 is correlated with poor prognosis of oral squamous cell carcinoma. Pathol Res Pract 2018;214:1606-12. [Crossref] [PubMed]
- Bignotti E, Zanotti L, Calza S, et al. Trop-2 protein overexpression is an independent marker for predicting disease recurrence in endometrioid endometrial carcinoma. BMC Clin Pathol 2012;12:22. [Crossref] [PubMed]
- Calvo A, Xiao N, Kang J, et al. Alterations in gene expression profiles during prostate cancer progression: functional correlations to tumorigenicity and down-regulation of selenoprotein-P in mouse and human tumors. Cancer Res 2002;62:5325-35.
- Hsu EC, Rice MA, Bermudez A, et al. Trop2 is a driver of metastatic prostate cancer with neuroendocrine phenotype via PARP1. Proc Natl Acad Sci U S A 2020;117:2032-42. [Crossref] [PubMed]
- Mühlmann G, Spizzo G, Gostner J, et al. TROP2 expression as prognostic marker for gastric carcinoma. J Clin Pathol 2009;62:152-8. [Crossref] [PubMed]
- Fong D, Spizzo G, Gostner JM, et al. TROP2: a novel prognostic marker in squamous cell carcinoma of the oral cavity. Mod Pathol 2008;21:186-91. [Crossref] [PubMed]
- Sin STK, Li Y, Liu M, et al. Down-regulation of TROP-2 Predicts Poor Prognosis of Hepatocellular Carcinoma Patients. Hepatol Commun 2018;2:1408-14. [Crossref] [PubMed]
- Lin JC, Wu YY, Wu JY, et al. TROP2 is epigenetically inactivated and modulates IGF-1R signalling in lung adenocarcinoma. EMBO Mol Med 2012;4:472-85. [Crossref] [PubMed]
- Ambrogi F, Fornili M, Boracchi P, et al. Trop-2 is a determinant of breast cancer survival. PLoS One 2014;9:e96993. [Crossref] [PubMed]
- Lin H, Zhang H, Wang J, et al. A novel human Fab antibody for Trop2 inhibits breast cancer growth in vitro and in vivo. Int J Cancer 2014;134:1239-49. [Crossref] [PubMed]
- Varughese J, Cocco E, Bellone S, et al. Uterine serous papillary carcinomas overexpress human trophoblast-cell-surface marker (Trop-2) and are highly sensitive to immunotherapy with hRS7, a humanized anti-Trop-2 monoclonal antibody. Cancer 2011;117:3163-72. [Crossref] [PubMed]
- Bignotti E, Ravaggi A, Romani C, et al. Trop-2 overexpression in poorly differentiated endometrial endometrioid carcinoma: implications for immunotherapy with hRS7, a humanized anti-trop-2 monoclonal antibody. Int J Gynecol Cancer 2011;21:1613-21. [Crossref] [PubMed]
- Kaplon H, Muralidharan M, Schneider Z, et al. Antibodies to watch in 2020. MAbs 2020;12:1703531. [Crossref] [PubMed]
- Sahota S, Vahdat LT. Sacituzumab govitecan: an antibody-drug conjugate. Expert Opin Biol Ther 2017;17:1027-31. [Crossref] [PubMed]
- Stein R, Basu A, Chen S, et al. Specificity and properties of MAb RS7-3G11 and the antigen defined by this pancarcinoma monoclonal antibody. Int J Cancer 1993;55:938-46. [Crossref] [PubMed]
- Varughese J, Cocco E, Bellone S, et al. High-grade, chemotherapy-resistant primary ovarian carcinoma cell lines overexpress human trophoblast cell-surface marker (Trop-2) and are highly sensitive to immunotherapy with hRS7, a humanized monoclonal anti-Trop-2 antibody. Gynecol Oncol 2011;122:171-7. [Crossref] [PubMed]
- Varughese J, Cocco E, Bellone S, et al. Cervical carcinomas overexpress human trophoblast cell-surface marker (Trop-2) and are highly sensitive to immunotherapy with hRS7, a humanized monoclonal anti-Trop-2 antibody. Am J Obstet Gynecol 2011;205:567.e1-7. [Crossref] [PubMed]
- Raji R, Guzzo F, Carrara L, et al. Uterine and ovarian carcinosarcomas overexpressing Trop-2 are sensitive to hRS7, a humanized anti-Trop-2 antibody. J Exp Clin Cancer Res 2011;30:106. [Crossref] [PubMed]
- Shih LB, Xuan H, Aninipot R, et al. In vitro and in vivo reactivity of an internalizing antibody, RS7, with human breast cancer. Cancer Res 1995;55:5857s-63s.
- van Rij CM, Frielink C, Goldenberg DM, et al. Pretargeted immunoPET of prostate cancer with an anti-TROP-2 x anti-HSG bispecific antibody in mice with PC3 xenografts. Mol Imaging Biol 2015;17:94-101. [Crossref] [PubMed]
- van Rij CM, Lütje S, Frielink C, et al. Pretargeted immuno-PET and radioimmunotherapy of prostate cancer with an anti-TROP-2 x anti-HSG bispecific antibody. Eur J Nucl Med Mol Imaging 2013;40:1377-83. [Crossref] [PubMed]
- Sharkey RM, van Rij CM, Karacay H, et al. A new Tri-Fab bispecific antibody for pretargeting Trop-2-expressing epithelial cancers. J Nucl Med 2012;53:1625-32. [Crossref] [PubMed]
- Rossi EA, Rossi DL, Cardillo TM, et al. Redirected T-cell killing of solid cancers targeted with an anti-CD3/Trop-2-bispecific antibody is enhanced in combination with interferon-α. Mol Cancer Ther 2014;13:2341-51. [Crossref] [PubMed]
- Ahmed KA, Munegowda MA, Xie Y, et al. Intercellular trogocytosis plays an important role in modulation of immune responses. Cell Mol Immunol 2008;5:261-9. [Crossref] [PubMed]
- Rossi DL, Rossi EA, Cardillo TM, et al. A new class of bispecific antibodies to redirect T cells for cancer immunotherapy. MAbs 2014;6:381-91. [Crossref] [PubMed]
- Liu J, Yang D, Yin Z, et al. A novel human monoclonal Trop2-IgG antibody inhibits ovarian cancer growth in vitro and in vivo. Biochem Biophys Res Commun 2019;512:276-82. [Crossref] [PubMed]
- Seligson JM, Patron AM, Berger MJ, et al. Sacituzumab Govitecan-hziy: An Antibody-Drug Conjugate for the Treatment of Refractory, Metastatic, Triple-Negative Breast Cancer. Ann Pharmacother 2021;55:921-31. [Crossref] [PubMed]
- Bardia A, Mayer IA, Diamond JR, et al. Efficacy and Safety of Anti-Trop-2 Antibody Drug Conjugate Sacituzumab Govitecan (IMMU-132) in Heavily Pretreated Patients With Metastatic Triple-Negative Breast Cancer. J Clin Oncol 2017;35:2141-8. [Crossref] [PubMed]
- Weiss J, Glode A, Messersmith WA, et al. Sacituzumab govitecan: breakthrough targeted therapy for triple-negative breast cancer. Expert Rev Anticancer Ther 2019;19:673-9. [Crossref] [PubMed]
- Starodub AN, Ocean AJ, Shah MA, et al. First-in-Human Trial of a Novel Anti-Trop-2 Antibody-SN-38 Conjugate, Sacituzumab Govitecan, for the Treatment of Diverse Metastatic Solid Tumors. Clin Cancer Res 2015;21:3870-8. [Crossref] [PubMed]
- Lopez S, Perrone E, Bellone S, et al. Preclinical activity of sacituzumab govitecan (IMMU-132) in uterine and ovarian carcinosarcomas. Oncotarget 2020;11:560-70. [Crossref] [PubMed]
- Cardillo TM, Govindan SV, Sharkey RM, et al. Sacituzumab Govitecan (IMMU-132), an Anti-Trop-2/SN-38 Antibody-Drug Conjugate: Characterization and Efficacy in Pancreatic, Gastric, and Other Cancers. Bioconjug Chem 2015;26:919-31. [Crossref] [PubMed]
- Cardillo TM, Govindan SV, Sharkey RM, et al. Humanized anti-Trop-2 IgG-SN-38 conjugate for effective treatment of diverse epithelial cancers: preclinical studies in human cancer xenograft models and monkeys. Clin Cancer Res 2011;17:3157-69. [Crossref] [PubMed]
- Shastry M, Jacob S, Rugo HS, et al. Antibody-drug conjugates targeting TROP-2: Clinical development in metastatic breast cancer. Breast 2022;66:169-77. [Crossref] [PubMed]
- Okajima D, Yasuda S, Maejima T, et al. Datopotamab Deruxtecan, a Novel TROP2-directed Antibody-drug Conjugate, Demonstrates Potent Antitumor Activity by Efficient Drug Delivery to Tumor Cells. Mol Cancer Ther 2021;20:2329-40. [Crossref] [PubMed]
- Liu D, Cardillo TM, Wang Y, et al. Trop-2-targeting tetrakis-ranpirnase has potent antitumor activity against triple-negative breast cancer. Mol Cancer 2014;13:53. [Crossref] [PubMed]
- Govindan SV, Goldenberg DM. New antibody conjugates in cancer therapy. ScientificWorldJournal 2010;10:2070-89. [Crossref] [PubMed]
- Chang CH, Gupta P, Michel R, et al. Ranpirnase (frog RNase) targeted with a humanized, internalizing, anti-Trop-2 antibody has potent cytotoxicity against diverse epithelial cancer cells. Mol Cancer Ther 2010;9:2276-86. [Crossref] [PubMed]
- Gottesman MM, Fojo T, Bates SE. Multidrug resistance in cancer: role of ATP-dependent transporters. Nat Rev Cancer 2002;2:48-58. [Crossref] [PubMed]
- Szakács G, Paterson JK, Ludwig JA, et al. Targeting multidrug resistance in cancer. Nat Rev Drug Discov 2006;5:219-34. [Crossref] [PubMed]
- Chang CH, Wang Y, Zalath M, et al. Combining ABCG2 Inhibitors with IMMU-132, an Anti-Trop-2 Antibody Conjugate of SN-38, Overcomes Resistance to SN-38 in Breast and Gastric Cancers. Mol Cancer Ther 2016;15:1910-9. [Crossref] [PubMed]
- Urasaki Y, Laco GS, Pourquier P, et al. Characterization of a novel topoisomerase I mutation from a camptothecin-resistant human prostate cancer cell line. Cancer Res 2001;61:1964-9.
- Tomicic MT, Kaina B. Topoisomerase degradation, DSB repair, p53 and IAPs in cancer cell resistance to camptothecin-like topoisomerase I inhibitors. Biochim Biophys Acta 2013;1835:11-27. [Crossref] [PubMed]
- Das SK, Rehman I, Ghosh A, et al. Poly(ADP-ribose) polymers regulate DNA topoisomerase I (Top1) nuclear dynamics and camptothecin sensitivity in living cells. Nucleic Acids Res 2016;44:8363-75. [Crossref] [PubMed]
- Cardillo TM, Sharkey RM, Rossi DL, et al. Synthetic Lethality Exploitation by an Anti-Trop-2-SN-38 Antibody-Drug Conjugate, IMMU-132, Plus PARP Inhibitors in BRCA1/2-wild-type Triple-Negative Breast Cancer. Clin Cancer Res 2017;23:3405-15. [Crossref] [PubMed]
- Aki M, Shimbara N, Takashina M, et al. Interferon-gamma induces different subunit organizations and functional diversity of proteasomes. J Biochem 1994;115:257-69. [Crossref] [PubMed]
- Mangino G, Grazia Capri M, Barnaba V, et al. Presentation of native TROP-2 tumor antigens to human cytotoxic T lymphocytes by engineered antigen-presenting cells. Int J Cancer 2002;101:353-9. [Crossref] [PubMed]
- Goldenberg DM. Targeted therapy of cancer with radiolabeled antibodies. J Nucl Med 2002;43:693-713.
- Stein R, Govindan SV, Chen S, et al. Successful therapy of a human lung cancer xenograft using MAb RS7 labeled with residualizing radioiodine. Crit Rev Oncol Hematol 2001;39:173-80. [Crossref] [PubMed]
- Stein R, Blumenthal R, Sharkey RM, et al. Comparative biodistribution and radioimmunotherapy of monoclonal antibody RS7 and its F(ab')2 in nude mice bearing human tumor xenografts. Cancer 1994;73:816-23. [Crossref] [PubMed]
- Govindan SV, Stein R, Qu Z, et al. Preclinical therapy of breast cancer with a radioiodinated humanized anti-EGP-1 monoclonal antibody: advantage of a residualizing iodine radiolabel. Breast Cancer Res Treat 2004;84:173-82. [Crossref] [PubMed]
- van Rij CM, Sharkey RM, Goldenberg DM, et al. Imaging of prostate cancer with immuno-PET and immuno-SPECT using a radiolabeled anti-EGP-1 monoclonal antibody. J Nucl Med 2011;52:1601-7. [Crossref] [PubMed]
- Mitsunaga M, Ogawa M, Kosaka N, et al. Cancer cell-selective in vivo near infrared photoimmunotherapy targeting specific membrane molecules. Nat Med 2011;17:1685-91. [Crossref] [PubMed]
- Nishimura T, Mitsunaga M, Sawada R, et al. Photoimmunotherapy targeting biliary-pancreatic cancer with humanized anti-TROP2 antibody. Cancer Med 2019;8:7781-92. [Crossref] [PubMed]
- Cubas R, Zhang S, Li M, et al. Chimeric Trop2 virus-like particles: a potential immunotherapeutic approach against pancreatic cancer. J Immunother 2011;34:251-63. [Crossref] [PubMed]
- Son S, Shin S, Rao NV, et al. Anti-Trop2 antibody-conjugated bioreducible nanoparticles for targeted triple negative breast cancer therapy. Int J Biol Macromol 2018;110:406-15. [Crossref] [PubMed]
- Farivar TN, Najafipour R, Johari P. Nano - drug Delivery of Apoptosis Activator 2 to AGS Cells by Liposomes Conjugated with Anti-TROP2 Antibody. N Am J Med Sci 2012;4:582-5. [Crossref] [PubMed]
- Zhao W, Jia L, Zhang M, et al. The killing effect of novel bi-specific Trop2/PD-L1 CAR-T cell targeted gastric cancer. Am J Cancer Res 2019;9:1846-56.
- Chen H, Wei F, Yin M, et al. CD27 enhances the killing effect of CAR T cells targeting trophoblast cell surface antigen 2 in the treatment of solid tumors. Cancer Immunol Immunother 2021;70:2059-71. [Crossref] [PubMed]
- Corti C, Venetis K, Sajjadi E, et al. CAR-T cell therapy for triple-negative breast cancer and other solid tumors: preclinical and clinical progress. Expert Opin Investig Drugs 2022;31:593-605. [Crossref] [PubMed]
- Li G, Guo X, Tang L, et al. Analysis of BRCA1/2 mutation spectrum and prevalence in unselected Chinese breast cancer patients by next-generation sequencing. J Cancer Res Clin Oncol 2017;143:2011-24. [Crossref] [PubMed]
- Huang P, Ouyang DJ, Chang S, et al. Chemotherapy-driven increases in the CDKN1A/PTN/PTPRZ1 axis promote chemoresistance by activating the NF-κB pathway in breast cancer cells. Cell Commun Signal 2018;16:92. [Crossref] [PubMed]
- Li L, Han D, Wang X, et al. Prognostic values of Ki-67 in neoadjuvant setting for breast cancer: a systematic review and meta-analysis. Future Oncol 2017;13:1021-34. [Crossref] [PubMed]
- Yao J, Xu F, Zhang D, et al. TP73-AS1 promotes breast cancer cell proliferation through miR-200a-mediated TFAM inhibition. J Cell Biochem 2018;119:680-90. [Crossref] [PubMed]
- Zou Q, Zhou E, Xu F, et al. A TP73-AS1/miR-200a/ZEB1 regulating loop promotes breast cancer cell invasion and migration. J Cell Biochem 2018;119:2189-99. [Crossref] [PubMed]
(English Language Editor: A. Kassem)