B and T lymphocyte attenuator as a C-reactive protein and IgA associated auxiliary diagnostic marker for pulmonary tuberculosis: a case-control study
Highlight box
Key findings
• Our study found that the detection of BTLA expression on the surface of specific peripheral blood cells has certain significance for the auxiliary diagnosis of tuberculosis.
What is known and what is new?
• According to literature reports, the expression of BTLA in tuberculosis has been reported, but not systematically enough.
• We systematically analyzed the expression changes of BTLA in peripheral blood and different cell types, and evaluated its auxiliary diagnostic value in the diagnosis of tuberculosis.
What is the implication, and what should change now?
• We verified the role of the hematological molecular marker BTLA in the diagnosis of tuberculosis, and its detection can be increased in the subsequent diagnosis of tuberculosis to improve the auxiliary diagnosis of tuberculosis.
Introduction
Tuberculosis (TB) is an acute and chronic respiratory disease caused by Mycobacterium tuberculosis (MTB) infection (1). Therapeutic strategies for TB have been well established, and most patients achieve recovery after a regular course of treatment. However, in recent years, with the increasing incidence of drug-resistant TB, the cooccurrence of various TB complications, and hypoimmunity of patients themselves, the incidence of TB is showing a resurgence (2). According to the 2021 Global TB Study, the number of new cases in China was 842,000 in 2020, ranking second to India among the 30 countries with high TB burden globally (3).
Studies have found that early detection and timely treatment of TB can effectively promote clinical cures (4). A variety of diagnostic methods for TB have been developed and applied in clinical practice, each with their own advantages and disadvantages. Traditional diagnostic methods for TB etiology include TB smear and TB culture. The advantages of such methods are that TB can be diagnosed once the etiological basis is found. However, there are also many disadvantages, including a low positive rate of etiology, high infectivity of specimens, and dependence on patients’ subjective specimen retention (5,6). Researchers have applied tuberculosis antibody (TB-Ab) in the auxiliary diagnosis of TB, but its false positive rate is high and thus cannot provide clinicians certainty in the diagnosis of TB (7). TB-RNA and TB-DNA have also been applied in the diagnosis of TB, and although they solve the problem of detection time, their infectivity and positive rate of etiology are still important factors impacting TB diagnosis (8,9). With advances in technology, a variety of molecular diagnostic techniques for TB, such as the fluorescence polymerase chain reaction (PCR) solution-curve method and GeneXpert, have become common in clinical practice, but the costs are relatively high, increasing the economic burden of TB patients (10,11). TB infectious T-lymphocyte spot assay (T-SPOT.TB) is a widely used hematological indicator of TB. Although the test is objective, it can only determine MTB infection. Its role in active TB and latent TB infection needs to be further studied (12). Therefore, a new molecular marker based on blood for the clinical diagnosis of TB is urgently needed for early diagnosis, timely treatment, and therapeutic effect monitoring of TB.
B and T lymphocyte attenuator (BTLA), a member of the CD28 superfamily, is mainly involved in immune suppression of the body as a costimulatory signaling molecule (13). The combination of BTLA with its ligand, herpesvirus entry mediator (HVEM) (14), is important in a variety of diseases, including inflammatory diseases, tumors, autoimmune diseases, infectious diseases, and graft rejection (15-17). BTLA has a key role in host T cells, B cells, and dendritic cells (DCs) (18-20). MTB is an intracellular parasitic bacterium in which T cells play a vital part in host immunity (21). After infecting the human body through the respiratory tract, MTB is first engulfed by alveolar macrophages. As the first line of defense in the host body, macrophages phagocytose MTB and initiate specific immune responses through antigen presentation (22). Activated CD4+ T cells can positively regulate macrophage antituberculosis infection by secreting cytokines such as interferon-γ (IFN-γ) and tumor necrosis factor-α (TNF-α). CD8+ T cells kill MTB mainly through the release of cytoplasmic granzyme and Fas/Fas ligand (FasL) to induce apoptosis of target cells (23). The role of BTLA in TB infection has been reported. Researchers have found that BTLA plays a role in CD4+ T cells, CD8+ T cells, mononuclear macrophages, and DCs after TB infection and can reflect the immune function of patients to a certain extent (24). Song et al. found the percentage of BTLA expression on CD4+ T cells or CD8+ T cells was positively correlated with the levels of aspartate aminotransferase (AST) and alanine aminotransaminase (ALT) (25). However, BTLA expression and its diagnostic efficacy in the peripheral blood and various cells of newly treated pulmonary tuberculosis (PTB) patients, along with its correlation with clinical features, has rarely been reported.
Thus, in our study, peripheral blood samples of pulmonary TB patients and healthy controls were collected, and the expression of BTLA on the surface of different types of peripheral blood cells was detected by flow cytometry. We also analyzed the correlation between BTLA and clinical indicators. Our study aimed to explore the diagnostic efficacy of BTLA in pulmonary TB and systematically analyze the value of BTLA in the auxiliary diagnosis of pulmonary TB. We present the following article in accordance with the STARD reporting checklist (available at https://atm.amegroups.com/article/view/10.21037/atm-22-6060/rc).
Methods
Research objects
Patients with clinically confirmed pulmonary TB between January 2020 and December 2021 at The Sixth People’s Hospital of Nantong and The Third People’s Hospital of Nantong were selected as the research objects of this study. Patients’ fresh heparin anticoagulated peripheral blood was collected. At the same time, a healthy population at The Sixth People’s Hospital of Nantong was used as the control group. For the TB case group (TB group), inclusion was based on the clinician’s disease diagnosis. All TB patients included in this study were newly treated for pulmonary TB and had a clear etiological diagnosis basis and obvious tuberculous chest imaging changes. For the healthy control group (control group), the criteria were as follows: T-SPOT or purified protein derivative (PPD) test was negative, and the chief complaint was no history of TB or TB exposure, with no abnormality in chest computed tomography (CT) or X-ray imaging examination. The gender and age ranges of the included subjects were matched with those of the experimental group and they were all Han nationality. The study was conducted in accordance with the Declaration of Helsinki (as revised in 2013). The study was approved by Ethics Committee of Affiliated Nantong Hospital of Shanghai University (The Sixth People’s Hospital of Nantong) and The Third People’s Hospital of Nantong (Nos. NTLYLL2019008 and EL2019021), and informed consent was taken from all the participants. Some other diseases were excluded, in addition to immune suppression-related diseases, tumors, and other infectious diseases.
Flow cytometry assay
Briefly, for flow cytometry detection, 4 groups were established: set blank group, isotype control group, fluorescent single staining group, and experimental group. Heparin anticoagulant fresh whole blood (100 µL) was taken, added to the flow tube, and mixed with corresponding fluorescent antibody for staining in the dark After 30 minutes, 2 mL of red blood cell lysate (Mindray Biotech Co., Ltd., Shenzhen, China) was added to each tube and lysed for 20 minutes. The supernatant was carefully absorbed and discarded after being centrifugated at 1,500 rpm for 5 minutes. Next, 1 mL phosphate-buffered saline (PBS) was added to each tube to wash the cells. Finally, 200 µL PBS was added to the tubes, mixed well, and testing was performed using a FC500 flow cytometry analysis system (Beckman Coulter, Brea, CA, USA). The blank group, isotype control group, and fluorescence single staining group were used to adjust the staining channel and baseline. The original experimental drawings were saved and the experimental results were analyzed with Kaluza Analysis Software (Beckman Coulter). All fluorescent antibodies were purchased from BD Biosciences (San Jose, CA, USA).
Real-time PCR (RT-PCR) analysis
Total RNA was obtained by Trizol reagent (Invitrogen, Carlsbad, CA, USA) according to the manufacturer’s instruction and then reverse transcribed into complementary DNA (cDNA) using the Revert Aid First Strand cDNA Synthesis Kit (Thermo Fisher Scientific, Waltham, MA, USA). RT-PCR was performed according to the protocol of SYBR Premix Ex Taq RT-PCR Kit (Takara, Kusatsu, Shiga, Japan) in an Eco Real-time PCR system (Illumina, San Diego, CA, USA). Based on the known BTLA and HVEM gene sequences provided by GeneBank, primers were designed using Primer5.0 software and synthesized by Shanghai Jikai Biotechnology Co., LTD. Primer sequence: the human BTLA-F: 5'-CATCTTAGCAGGAGATCCCTTTG-3', The Human BTLA-R: 5'-GACCCATTGTCATTAGGAAGCA-3'; The Human HVEM-F: 5'-CTTGAGGCTGGTGCTGTATC-3', the human HVEM-R: 5'-CTTCACACGATAACCTGGAC-3'. The following components were mixed into the real-time quantitative PCR plate: upstream primer (10 µM) 0.4 µL, downstream primer (10 µm) 0.4 µL, 2.0 µL cDNA, SYBR®Premix Ex TaqTM (2×) 10.0 µL, double-distilled water (ddH2O) to 20 µL. The reaction conditions were: 95 ℃ for 30 seconds; 95 ℃ for 5 seconds, 56 ℃ for 30 seconds, 72 ℃ for 30 seconds, with 40 cycles, The dissolution curves were analyzed at 95 ℃ for 15 seconds, 56 ℃ for 30 seconds, and 95 ℃ for 15 seconds. The target gene values were normalized to glyceraldehyde 3-phosphate dehydrogenase (GAPDH) values and expressed as relative fold increases 2(−ΔΔCt).
Statistical analysis
An Excel table was used to sort the data of all subjects included in the study. All clinical characteristics were got from the patients clinical laboratory. Numerical statistics were calculated by means ± standard deviation (SD) and percentages. SPSS 17.0 software (SPSS Inc., Chicago, IL, USA) and GraphPad Prism version 5.0 (GraphPad Software, San Diego, CA, USA) were used for analysis and processing. SPSS was used for receiver operating characteristic (ROC) analysis [95% confidence interval (CI)], area under the curve (AUC), sensitivity, and specificity of the diagnosis. GraphPad was used to analyze paired and unpaired data using t-test, chi-square test, Pearson or Spearman correlation analysis. A two-sided statistical threshold of P<0.05 was used to determine the statistical difference in the analysis.
Results
General characteristics of the included subjects
Based on the inclusion criteria, 56 patients with pulmonary TB and 52 healthy controls were included in the study. The median age of pulmonary TB patients was 46.36±13.24 years old, and there were 38 males (67.56%) and 18 females (32.14%). In the healthy control group, the median age was 47.56±14.70 years old, and there were 35 males (67.31%) and 17 females (32.69%), with no statistical difference (P>0.05). All 56 cases of pulmonary TB patients had clear TB test results and imaging evidence. Among the TB cases, 52 were MTB smear positive (92.86%), 54 were MTB culture positive (96.43%), and 49 were TB-RNA positive (87.50%). There were 48 (85.71%) GeneXpert positive patients and 55 (98.21%) T-SPOT positive patients. In the imaging diagnosis, 10 cases (17.86%) had pulmonary cavity, and 46 cases (82.14%) had other nodules, plaques, and miliary lesions. The general characteristics of the patients are shown in Table 1.
Table 1
Characteristics | Control (n=52) | TB (n=56) | P |
---|---|---|---|
Age (years) | 47.56±14.70 | 46.36±13.24 | 0.8151 |
Sex (male/female) | 35/17 | 38/18 | 0.9546 |
MTB smear positive (%) | 0/52 (0) | 52/56 (92.86) | <0.0001*** |
MTB culture positive (%) | 0/52 (0) | 54/56 (96.43) | <0.0001*** |
TB-RNA positive (%) | 0/52 (0) | 49/56 (87.50) | <0.0001*** |
GeneXpert positive (%) | 0/52 (0) | 48/56 (85.71) | <0.0001*** |
T-SPOT positive (%) | 0/52 (0) | 55/56 (98.21) | <0.0001*** |
Pulmonary cavity (%) | 0/52 (0) | 10/56 (17.86) | <0.0001*** |
Other imaging changes (%) | 0/52 (0) | 46/56 (82.14) | <0.0001*** |
Numerical statistics were calculated by means ± SD and percentages (%). ***, P<0.0001. TB, tuberculosis; MTB, Mycobacterium tuberculosis; T-SPOT, T-lymphocyte spot assay; SD, standard deviation.
Expression of BTLA and HVEM in peripheral blood
In order to explore the role of BTLA and HVEM in pulmonary TB, we detected expression levels in 52 healthy controls and 56 pulmonary TB patients from whole blood RNA extraction, reverse transcription, and RT-PCR. As shown in Figure 1, the expression of BTLA gene was significantly different in the two groups (P=0.0187), while the expression of HVEM was not statistically different (P=0.4718). The results suggested that BTLA, but not its ligand, may have played a certain role in the diagnosis of pulmonary TB.
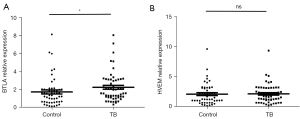
Expression of BTLA in alveolar macrophages
Since BTLA was highly expressed in the peripheral blood of patients with pulmonary TB, we needed to further confirm in which or which kinds of cells it was highly expressed. As the first line of defense in MTB-infected hosts, alveolar macrophages were the first cell type to be identified. BTLA was contained with molecular markers of macrophages, and the expression of BTLA cells was analyzed by flow cytometry. As shown in Figure 2, the proportion of BTLA+CD68+ cells in pulmonary TB patients was significantly higher than that in the control group (P=0.0004).
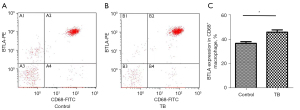
Changes of BTLA expression in specific antigen-presenting cells (APCs)
As reported in a previous study (26), BTLA is highly expressed in CD11c APCs. To confirm the expression of BTLA in CD11c, we also analyzed the expression of CD11c in peripheral blood mononuclear cells (PBMCs) and BTLA in CD11c. Our results showed that CD11c was highly expressed in PBMCs of TB patients (Figure 3A,3B; P<0.0001), and the proportion of BTLA+CD11c+ cells was also significantly higher in TB patients than in healthy controls (Figure 3C,3D; P<0.0001).
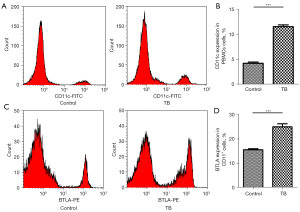
Expression changes of BTLA in CD4 T and CD8 T cells
To further confirm whether the expression of BTLA changes in effector T lymphocytes, we examined the expression of BTLA in CD4 T cells and CD8 T cells. As shown in Figure 4, there was no significant difference in BTLA+CD4+ and BTLA+CD8+ between the two groups (P=0.0792, P=0.8706, Figure 4).
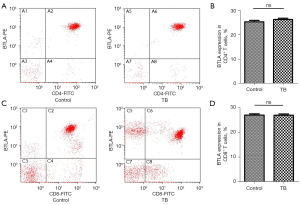
Correlation analysis between BTLA expression and clinical indicators
In order to further analyze the diagnostic efficacy of BTLA in pulmonary TB, we conducted a correlation analysis between the expression of BTLA and clinical indicators. Our results showed that the expression of CD11c + BTLA+ was negatively correlated with the expression of immunoglobulin A (IgA) (r=−0.2934, P=0.0282, Figure 5A) and positively correlated with high sensitivity C-reaction protein (CRP) (r=0.3277, P=0.0137, Figure 5B). There was no significant difference in other statistical indexes (P<0.05, Table 2). These findings suggested that BTLA played an auxiliary role in the diagnosis of pulmonary TB, especially in alveolar macrophages and specific APCs, and there was a certain correlation between the expression of BTLA and clinical indicators.
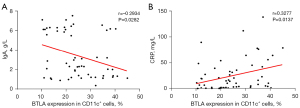
Table 2
Characteristics | BTLA (RT-PCR) | BTLA+CD68+ | BTLA+CD11c+ | |||||
---|---|---|---|---|---|---|---|---|
r | P | r | P | r | P | |||
Neu | −0.1375 | 0.3122 | 0.04079 | 0.7653 | −0.01569 | 0.9086 | ||
Mon | −0.05512 | 0.6866 | −0.009592 | 0.9441 | −0.1515 | 0.2652 | ||
Lym | −0.1225 | 0.3684 | 0.07902 | 0.5627 | −0.1726 | 0.2032 | ||
PLT | −0.1045 | 0.4435 | −0.0432 | 0.7519 | −0.1453 | 0.2852 | ||
RBC | 0.01937 | 0.8873 | 0.103 | 0.4502 | −0.2543 | 0.0586 | ||
HGB | 0.03373 | 0.8051 | 0.04513 | 0.7412 | −0.1638 | 0.2277 | ||
ESR | −0.008724 | 0.9491 | −0.01431 | 0.9166 | −0.1646 | 0.2254 | ||
CRP | 0.1565 | 0.2494 | −0.1073 | 0.4314 | 0.3277 | 0.0137* | ||
PT | 0.1618 | 0.2334 | −0.004086 | 0.9762 | −0.006112 | 0.9643 | ||
APTT | 0.0487 | 0.7215 | −0.06598 | 0.629 | −0.06896 | 0.6136 | ||
FIB | −0.115 | 0.3986 | 0.02364 | 0.8627 | −0.07288 | 0.5935 | ||
D-D | −0.1313 | 0.3348 | −0.04755 | 0.7278 | −0.02612 | 0.8485 | ||
IgA | −0.1093 | 0.4226 | 0.02181 | 0.8732 | −0.2934 | 0.0282* | ||
IgM | 0.05823 | 0.6699 | 0.02046 | 0.881 | 0.1769 | 0.1922 | ||
IgG | −0.05415 | 0.6919 | 0.0954 | 0.4843 | 0.2143 | 0.1128 | ||
IgE | 0.2594 | 0.0536 | −0.08165 | 0.5497 | −0.171 | 0.2076 | ||
C3 | 0.03146 | 0.8179 | 0.02745 | 0.8408 | −0.2391 | 0.0760 | ||
C4 | 0.06191 | 0.6503 | −0.03419 | 0.8025 | −0.2611 | 0.0520 |
*, P<0.05. BTLA, B and T lymphocyte attenuator; RT-PCR, real-time polymerase chain reaction; Neu, neutrophil count; Mon, monocyte count; Lym, lymphocyte count; PLT, platelet count; RBC, red blood cells; HGB, hemoglobin; ESR, erythrocyte sedimentation rate; CRP, C-reaction protein; PT, prothrombin time; APTT, partially activated prothrombin time; FIB, fibrinogen; D-D, D-D dimer; IgA, immunoglobulin A; IgM, immunoglobulin M; IgG, immunoglobulin G; IgE, immunoglobulin E; C3, complement 3; C4, complement 4.
Analysis of detection efficiency of 3 detection methods for BTLA
In order to further express the diagnostic efficacy of RT-PCR, flow cytometry to detect the proportion of BTLA+CD68+ cells, and flow cytometry to detect the proportion of BTLA+CD11c+ cells in pulmonary TB, ROC curves were used to analyze the sensitivity, specificity, 95% CI, and AUC of the 3 methods. Our study found that the AUC of RT-PCR of BTLA for the detection of pulmonary TB was 0.6260, sensitivity was 56.36%, specificity was 52.94%, and the 95% CI was 0.5188–0.7333 (P=0.02545). The AUC of the BTLA+CD11c+ method was 0.7563, sensitivity was 70.00%, specificity was 82.61%, and the 95% CI was 0.6391–0.8734 (P<0.0001). The AUC of the BTLA+CD68+ method for detecting TB was 0.6883, sensitivity was 62.50%, specificity was 86.96%, and the 95% CI was 0.5656–0.8110 (P=0.0027) (Figure 6). These results indicated that flow cytometry had high diagnostic efficiency in detecting BTLA on specific cells and could be used as an auxiliary detection index for pulmonary TB.
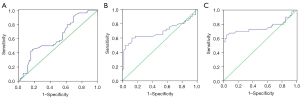
Discussion
TB, as an infectious disease with a long history, has certain clinical characteristics. One of the main manifestations is the low positive rate of etiology, resulting in difficulty with clinical diagnosis (27). The second manifestation is that there is no obvious hematologic diagnostic index for active pulmonary TB. For example, the routinely used T-SPOT test cannot distinguish between latent and active TB infection (28). Thirdly, due to the increase of drug resistance in TB, a certain treatment dilemma exists (29). The multiple complications of other manifestations of TB, such as AIDS infection and diabetes, have a great impact on the treatment and prognosis of TB (30). As a result, domestic and international researchers have been focusing on diagnosis based on the molecular marker of TB.
As an important molecule that can exert an immunosuppressive effect on the host through the activation of B and T cells, BTLA has been shown to play an important role in a variety of diseases, including some inflammatory diseases and immunosuppressive diseases, as well as in TB (13). At present, research on the role of BTLA in TB has mainly been on its expression in various specific cells (31). However, to date, few studies have comprehensively evaluated the role and diagnostic efficacy of BTLA in peripheral blood and different types of cells of TB. Through RT-PCR, our study found that the expression of BTLA was highly expressed in peripheral blood of patients with pulmonary TB, while there was no significant difference in HVEM between the TB and control groups. After MTB infects the host, it successively experiences alveolar macrophages, APCs, CD4 T cells, and CD8 T cells, among others, which play a role in vivo and determine the outcome of MTB infection (32). Our study comprehensively evaluated the changes in BTLA expression in these cell types. We found that BTLA was highly expressed on the surface of CD68 cells and CD11c APCs, while there was no significant difference in the expression of BTLA on CD4 T and CD8 T cells. These results indicated that BTLA played an important role through CD68 cells and CD11c APCs. Previous study had shown that BTLA expression levels in peripheral blood CD4+ and CD8+ T cells of smear-positive pulmonary TB patients were higher than those of controls. At the same time, the expression levels of BTLA in CD4+ and CD8+ T cells of retreated patients were higher than those of newly treated patients and were gradually upregulated with the increase of sputum bacterial load. In addition, the expression levels of BTLA on CD4+ and CD8+ T cells in patients with lung cavities were higher than those in patients without lung cavities (26). This may have been due to the different inclusion specimens of patients, and our study mainly focused on patients with newly diagnosed TB. Our study showed that changes in BTLA expression on the surface of CD68 cells and CD11c APCs had a certain auxiliary diagnostic efficacy in pulmonary TB, rather than directly detecting the expression of BTLA in peripheral blood.
In the primary stage of infection, MTB is engulfed by alveolar macrophages, and the phagocytic macrophages can directly kill MTB and produce an anti-TB effect (33). In addition, MTB phagocytic macrophages can also secrete some cytokines, including IFN-γ, TGF-β, and interleukin-10 (IL-10), to play an immune role (34). The process of MTB infection in the host body must be accompanied by changes in the expression of mononuclear macrophages, neutrophils, and some inflammatory factors. CRP is an acute phase reaction protein and reflects inflammatory changes in the body (35). Studies have found that CRP is closely related to the occurrence and development of TB, and it is currently commonly used as a blood marker for TB and infection surveillance. Ding et al. found that linezolid could kill bacteria by increasing serum procalcitonin (PCT), erythrocyte sedimentation rate (ESR), and CRP levels in the treatment of TB and pneumonia (36). Jiang et al. reported that elevated serum hs-CRP levels would increase the incidence of lung cancer in male patients with pulmonary TB (37). Goto et al. found that serum CRP level was closely related to whether TB patients had tuberculous cavities and dominant lung distribution (38). Researchers have also found that hsCRP could be used in pediatric TB diagnostic algorithms for TB exclusion. Further study had found that hsCRP has a certain correlation with prognosis and treatment in children (39). Our study found that the expression of CD11c+ BTLA was positively correlated with CRP, which may have been related to inflammatory changes in patients.
Study had reported that TB susceptibility is driven by immune dysfunction in both acute and chronic latency periods. Control of MTB infection after TB infection requires immune-mediated clearance of MTB (40). Therefore, it is thought that any kind of immune dysfunction that reverses the balance may contribute to the progression of the disease. Studies have shown that the biggest risk factors for TB include HIV-1 infection, malnutrition, diabetes, smoking, vitamin D deficiency, drug/alcohol abuse, male sex, and age, among others (41). In the process of clinical testing, immune-related indicators are used to evaluate the immune status of TB patients, including IgA, IgG, IgM, IgE, CD3, CD4, and CD8, etc. (42). Since MTB infection is mainly mediated through the respiratory tract, it has been demonstrated that MTB-specific IgA derived from mucosal immunity plays an important role in the defense against MTB (43). Studies have found that IgA-deficient mice are more sensitive to Mycobacterium Bovis infection, have higher bacterial loads in the lungs and bronchoalveolar lavage fluid, and have lower IFN-γ and TNF-α production in the lungs than wild-type mice (44). A recent study showed that MTB-specific IgA antibodies inhibited mycobacterial infection in epithelial cell lines, whereas IgG antibodies promoted MTB infection (45). In addition, secreted IgA purified from human colostrum mediated protection against MTB infection in mice (46). In addition to demonstrating protective effects, elevated IgA responses against MTB antigens have been evaluated for their diagnostic potential. Bezerra et al. reported that TB patients showed a specific IgA response to glycolipid antigens with a detection sensitivity of 88% and specificity of 89% compared with healthy controls (47). Legesse et al. described increased serum IgA levels against ESAT-6/CFP-10 and Rv2031 in culture-confirmed pulmonary tuberuculosis patients compared with healthy MTB-infected and non-infected individuals (48). IgA targeting other MTB antigens, such as LAM, PE35, P-90, PstS3, and MPT83, can also be detected in MTB infection (49-51).
Through our study, we found that the expression of BTLA in peripheral blood, macrophages, and specific APCs in the pulmonary TB group were significantly increased, and the expression of BTLA in CD68 and CD11c cells had high sensitivity and specificity, indicating that BTLA may have certain auxiliary diagnostic value in pulmonary TB. In addition, the expression of BTLA in CD11c cells had a certain correlation with clinical indicators, which could effectively reflect the response state of the body. However, due to the limited number of enrolled patients, the diagnostic role of BTLA in pulmonary TB needs to be further studied and confirmed.
Conclusions
In our study, BTLA is highly expressed in peripheral blood and specific cell types of patients with PTB and is correlated with specific clinical indicators. Thus, BTLA clinical hematological tests might serve an important method for the auxiliary diagnosis of PTB.
Acknowledgments
Funding: This work was supported financially by grants from the Foundation of Nantong Science and Technology Program (Grant No. JC12022005) and Nantong Sanitation and Health Committee Program (Nos. QA2020038 and JCZ20013).
Footnote
Reporting Checklist: The authors have completed the STARD reporting checklist. Available at https://atm.amegroups.com/article/view/10.21037/atm-22-6060/rc
Data Sharing Statement: Available at https://atm.amegroups.com/article/view/10.21037/atm-22-6060/dss
Conflicts of Interest: All authors have completed the ICMJE uniform disclosure form (available at https://atm.amegroups.com/article/view/10.21037/atm-22-6060/coif). The authors have no conflicts of interest to declare.
Ethical Statement: The authors are accountable for all aspects of the work in ensuring that questions related to the accuracy or integrity of any part of the work are appropriately investigated and resolved. The study was conducted in accordance with the Declaration of Helsinki (as revised in 2013). The study was approved by Ethics Committee of Affiliated Nantong Hospital of Shanghai University (The Sixth People’s Hospital of Nantong) and The Third People’s Hospital of Nantong (Nos. NTLYLL2019008 and EL2019021), and informed consent was taken from all the participants.
Open Access Statement: This is an Open Access article distributed in accordance with the Creative Commons Attribution-NonCommercial-NoDerivs 4.0 International License (CC BY-NC-ND 4.0), which permits the non-commercial replication and distribution of the article with the strict proviso that no changes or edits are made and the original work is properly cited (including links to both the formal publication through the relevant DOI and the license). See: https://creativecommons.org/licenses/by-nc-nd/4.0/.
References
- Su VY, Pan SW, Yen YF, et al. Statin use and impact on tuberculosis risk. Expert Rev Anti Infect Ther 2021;19:1093-8. [Crossref] [PubMed]
- Starshinova A, Malkova A, Kudryavtsev I, et al. Tuberculosis and autoimmunity: Common features. Tuberculosis (Edinb) 2022;134:102202. [Crossref] [PubMed]
- Bouzeyen R, Javid B. Therapeutic Vaccines for Tuberculosis: An Overview. Front Immunol 2022;13:878471. [Crossref] [PubMed]
- Dhali A, Das K, Dhali GK, et al. Abdominal tuberculosis: Clinical profile and outcome. Int J Mycobacteriol 2021;10:414-20. [Crossref] [PubMed]
- Li Y, Wang Y, Wang G, et al. Effectiveness of a case management model in newly treated smear-positive pulmonary tuberculosis patients. J Infect Dev Ctries 2021;15:1670-6. [Crossref] [PubMed]
- Anand E, Biswal N, Joseph NM. Effect of Neutralization of Gastric Aspirate on culture yield of mycobacterium tuberculosis in children with pulmonary tuberculosis. Indian J Tuberc 2021;68:431-6. [Crossref] [PubMed]
- Yu R, Hu S, Wang C, et al. Clinical diagnostic algorithm in defining tuberculous unilateral pleural effusion in high tuberculosis burden areas short of diagnostic tools. J Thorac Dis 2022;14:866-76. [Crossref] [PubMed]
- Qiu X, Zheng S, Yang J, et al. Comparing Mycobacterium tuberculosis RNA Accuracy in Various Respiratory Specimens for the Rapid Diagnosis of Pulmonary Tuberculosis. Infect Drug Resist 2022;15:4195-202. [Crossref] [PubMed]
- Bai W, Liu L, Wu L, et al. Assessing the utility of the Xpert Mycobacterium tuberculosis/rifampin assay for analysis of bronchoalveolar lavage fluid in patients with suspected pulmonary tuberculosis. J Clin Lab Anal 2022;36:e24154. [Crossref] [PubMed]
- Liang J, An H, Zhou J, et al. Exploratory development of PCR-fluorescent probes in rapid detection of mutations associated with extensively drug-resistant tuberculosis. Eur J Clin Microbiol Infect Dis 2021;40:1851-61. [Crossref] [PubMed]
- Brown S, Leavy JE, Jancey J. Implementation of GeneXpert for TB Testing in Low- and Middle-Income Countries: A Systematic Review. Glob Health Sci Pract 2021;9:698-710. [Crossref] [PubMed]
- Haas MK, Belknap RW. Diagnostic Tests for Latent Tuberculosis Infection. Clin Chest Med 2019;40:829-37. [Crossref] [PubMed]
- Ning Z, Liu K, Xiong H. Roles of BTLA in Immunity and Immune Disorders. Front Immunol 2021;12:654960. [Crossref] [PubMed]
- Bourque J, Hawiger D. The BTLA-HVEM-CD5 Immunoregulatory Axis-An Instructive Mechanism Governing pTreg Cell Differentiation. Front Immunol 2019;10:1163. [Crossref] [PubMed]
- Lee JB, Ha SJ, Kim HR. Clinical Insights Into Novel Immune Checkpoint Inhibitors. Front Pharmacol 2021;12:681320. [Crossref] [PubMed]
- Fang J, Chen F, Liu D, et al. Prognostic value of immune checkpoint molecules in breast cancer. Biosci Rep 2020;40:BSR20201054. [Crossref] [PubMed]
- Karabon L, Andrzejczak A, Ciszak L, et al. BTLA Expression in CLL: Epigenetic Regulation and Impact on CLL B Cell Proliferation and Ability to IL-4 Production. Cells 2021;10:3009. [Crossref] [PubMed]
- Ritthipichai K, Haymaker CL, Martinez M, et al. Multifaceted Role of BTLA in the Control of CD8+ T-cell Fate after Antigen Encounter. Clin Cancer Res 2017;23:6151-64. [Crossref] [PubMed]
- Axelsson S, Magnuson A, Lange A, et al. A combination of the activation marker CD86 and the immune checkpoint marker B and T lymphocyte attenuator (BTLA) indicates a putative permissive activation state of B cell subtypes in healthy blood donors independent of age and sex. BMC Immunol 2020;21:14. [Crossref] [PubMed]
- Zhang JA, Lu YB, Wang WD, et al. BTLA-Expressing Dendritic Cells in Patients With Tuberculosis Exhibit Reduced Production of IL-12/IFN-α and Increased Production of IL-4 and TGF-β, Favoring Th2 and Foxp3+ Treg Polarization. Front Immunol 2020;11:518. [Crossref] [PubMed]
- Lu YJ, Barreira-Silva P, Boyce S, et al. CD4 T cell help prevents CD8 T cell exhaustion and promotes control of Mycobacterium tuberculosis infection. Cell Rep 2021;36:109696. [Crossref] [PubMed]
- Counoupas C, Triccas JA. The generation of T-cell memory to protect against tuberculosis. Immunol Cell Biol 2019;97:656-63. [Crossref] [PubMed]
- Sharan R, Singh DK, Rengarajan J, et al. Characterizing Early T Cell Responses in Nonhuman Primate Model of Tuberculosis. Front Immunol 2021;12:706723. [Crossref] [PubMed]
- Ogongo P, Tezera LB, Ardain A, et al. Tissue-resident-like CD4+ T cells secreting IL-17 control Mycobacterium tuberculosis in the human lung. J Clin Invest 2021;131:142014. [Crossref] [PubMed]
- Song HF, Chen XJ, Tang PJ, et al. Clinical Significance of BTLA and HVEM Expression on Circulating CD4+ T and CD8+ T Cells in Chronic Hepatitis B Virus Infection. Viral Immunol 2022;35:291-302. [Crossref] [PubMed]
- Wang WD, Gao YC, Lu YB, et al. BTLA-expressing CD11c antigen presenting cells in patients with active tuberculosis exhibit low capacity to stimulate T cell proliferation. Cell Immunol 2017;311:28-35. [Crossref] [PubMed]
- Suárez I, Fünger SM, Kröger S, et al. The Diagnosis and Treatment of Tuberculosis. Dtsch Arztebl Int 2019;116:729-35. [Crossref] [PubMed]
- Luo Y, Xue Y, Mao L, et al. Diagnostic Value of T-SPOT.TB Assay for Tuberculous Peritonitis: A Meta-Analysis. Front Med (Lausanne) 2020;7:585180. [Crossref] [PubMed]
- Migliori GB, Tiberi S, Zumla A, et al. MDR/XDR-TB management of patients and contacts: Challenges facing the new decade. The 2020 clinical update by the Global Tuberculosis Network. Int J Infect Dis 2020;92S:S15-25. [Crossref] [PubMed]
- Meintjes G, Brust JCM, Nuttall J, et al. Management of active tuberculosis in adults with HIV. Lancet HIV 2019;6:e463-74. [Crossref] [PubMed]
- Barham MS, Abrahams DA, Khayumbi J, et al. HIV Infection Is Associated With Downregulation of BTLA Expression on Mycobacterium tuberculosis-Specific CD4 T Cells in Active Tuberculosis Disease. Front Immunol 2019;10:1983. [Crossref] [PubMed]
- Bussi C, Gutierrez MG. Mycobacterium tuberculosis infection of host cells in space and time. FEMS Microbiol Rev 2019;43:341-61. [Crossref] [PubMed]
- Furin J, Cox H, Pai M. Tuberculosis. Lancet 2019;393:1642-56. [Crossref] [PubMed]
- Dheda K, Barry CE 3rd, Maartens G. Tuberculosis. Lancet 2016;387:1211-26. [Crossref] [PubMed]
- El Amrani M, Asserraji M, Bahadi A, et al. Tuberculosis in hemodialysis. Med Sante Trop 2016;26:262-6. [Crossref] [PubMed]
- Ding RD, Zhang HJ. Effect of linezolid on serum PCT, ESR, and CRP in patients with pulmonary tuberculosis and pneumonia. Medicine (Baltimore) 2018;97:e12177. [Crossref] [PubMed]
- Jiang Y, Ni K, Fang M, et al. The Effects of Serum hs-CRP on the Incidence of Lung Cancer in Male Patients with Pulmonary Tuberculosis. Iran J Public Health 2019;48:1265-9.
- Goto A, Komiya K, Kan T, et al. Factors associated with atypical radiological findings of pulmonary tuberculosis. PLoS One 2019;14:e0220346. [Crossref] [PubMed]
- Jaganath D, Reza TF, Wambi P, et al. The Role of C-Reactive Protein as a Triage Tool for Pulmonary Tuberculosis in Children. J Pediatric Infect Dis Soc 2022;11:316-21. [Crossref] [PubMed]
- Cardona PJ. Pathogenesis of tuberculosis and other mycobacteriosis. Enferm Infecc Microbiol Clin 2018;36:38-46. (Engl Ed). [Crossref] [PubMed]
- Khader SA, Divangahi M, Hanekom W, et al. Targeting innate immunity for tuberculosis vaccination. J Clin Invest 2019;129:3482-91. [Crossref] [PubMed]
- Venketaraman V, Kaushal D, Saviola B. Mycobacterium tuberculosis. J Immunol Res 2015;2015:857598. [Crossref] [PubMed]
- Casadevall A. Antibodies to Mycobacterium tuberculosis. N Engl J Med 2017;376:283-5. [Crossref] [PubMed]
- Rodríguez A, Tjärnlund A, Ivanji J, et al. Role of IgA in the defense against respiratory infections IgA deficient mice exhibited increased susceptibility to intranasal infection with Mycobacterium bovis BCG. Vaccine 2005;23:2565-72. [Crossref] [PubMed]
- Zimmermann N, Thormann V, Hu B, et al. Human isotype-dependent inhibitory antibody responses against Mycobacterium tuberculosis. EMBO Mol Med 2016;8:1325-39. [Crossref] [PubMed]
- Alvarez N, Otero O, Camacho F, et al. Passive administration of purified secretory IgA from human colostrum induces protection against Mycobacterium tuberculosis in a murine model of progressive pulmonary infection. BMC Immunol 2013;14:S3. [Crossref] [PubMed]
- Bezerra JM, Beck ST, Kanunfre KA, et al. A study of IgA antibody response to different mycobacterium tuberculosis antigens in the diagnosis and monitoring of pulmonary tuberculosis. Braz J Infect Dis 2009;13:53-8. [Crossref] [PubMed]
- Legesse M, Ameni G, Medhin G, et al. IgA response to ESAT-6/CFP-10 and Rv2031 antigens varies in patients with culture-confirmed pulmonary tuberculosis, healthy Mycobacterium tuberculosis-infected and non-infected individuals in a tuberculosis endemic setting, Ethiopia. Scand J Immunol 2013;78:266-74. [Crossref] [PubMed]
- Baumann R, Kaempfer S, Chegou NN, et al. Serologic diagnosis of tuberculosis by combining Ig classes against selected mycobacterial targets. J Infect 2014;69:581-9. [Crossref] [PubMed]
- Conde MB, Suffys P, Lapa E, Silva JR, et al. Immunoglobulin A (IgA) and IgG immune responses against P-90 antigen for diagnosis of pulmonary tuberculosis and screening for Mycobacterium tuberculosis infection. Clin Diagn Lab Immunol 2004;11:94-7. [Crossref] [PubMed]
- Baumann R, Kaempfer S, Chegou NN, et al. A Subgroup of Latently Mycobacterium tuberculosis Infected Individuals Is Characterized by Consistently Elevated IgA Responses to Several Mycobacterial Antigens. Mediators Inflamm 2015;2015:364758. [Crossref] [PubMed]
(English Language Editor: A. Muijlwijk)