BDNF-overexpressing MSCs delivered by hydrogel in acute ischemic stroke treatment
Highlight box
Key findings
• Prolonged survival of transplanted BDNF-overexpressing MSCs with a hydrogel delivery system could promote the recovery of ischemic stroke via the continuous release of BDNF.
What is known and what is new?
• MSCs have been used for ischemic stroke treatment. However, poor survival of MSCs after transplantation limits the therapy efficacy.
• Here, we reported a robust MSC delivery system with hydrogel, which promoted the survival of MSCs in ischemic environment. Hydrogel carrying BDNF-overexpressing MSCs could significantly promoted the ischemic stroke recovery.
What is the implication, and what should change now?
• The hydrogel delivery system we established could be used for stem cell treatment for ischemic stroke. Varieties of stem cells could be detected in further study.
Introduction
Ischemic stroke is a leading cause of mortality and disability (1). The costs associated with ischemic stroke create a significant social burden worldwide (2). Fibrinolytic therapy is often used for stroke treatment but is limited by a narrow therapeutic window (3). Stroke therapy has been developing rapidly, and stem cell-based therapies are emerging as an encouraging approach for promoting long-term recovery after ischemic stroke. Mesenchymal stem cells (MSCs), mainly found in bone marrow, can develop into different kinds of cells, including osteoblasts, chondrocytes, adipocytes, and hepatocytes (4-6). A previous study confirmed that MSCs could promote recovery of ischemic stroke through angiogenesis, neurotrophic factors secretion, apoptosis inhibition and immune system modulation (1). Numerous neurotrophic factors are secreted by MSCs, including nerve growth factor (NGF), brain-derived neurotrophic factor (BDNF), vascular endothelial growth factor (VEGF), insulin-like growth factor 1 (IGF-1), and hepatocyte growth factor (HGF) (7-9). Prior research has confirmed that MSCs could promote ischemic stroke recovery via secretion of neurotrophic factors (10). BDNF has been reported to promote neurogenesis, increase white matter recovery, and enhance angiogenesis (11), and a previous study has found that BDNF-overexpressing MSCs could promote the recovery of ischemic stroke (12). Our previous study also confirmed that BDNF plays an important role in ischemic stroke recovery (3,11). However, MSCs are unable to survive a long time in the ischemic environment, and it has been reported that a small number of stem cells remain at the injected site within days post-injection (13). Biomaterial loading cells have been developed to overcome initial cell loss and increase cell survival ability after transplantation (14,15). Thus, the combination of hydrogel and BDNF-overexpressing MSCs may provide a mechanism for long-term BDNF delivery to infarct brain areas, promoting recovery after ischemic stroke. Therapy effectiveness is improved by a better understanding of stem cell dynamics in vivo following transplantation. Stem cell tracking in vivo is made possible by molecular imaging (16). In our prior work, we effectively observed the complete temporal profile of cell dynamics in vivo by combining magnetic resonance imaging (MRI) and bioluminescence imaging (BLI) (3). In the current study, the aim was to confirm whether prolonged survival of BDNF-overexpressing MSCs delivered by hydrogel could promote the recovery of ischemic stroke via continuous release of BDNF. We present the following article in accordance with the ARRIVE reporting checklist (available at https://atm.amegroups.com/article/view/10.21037/atm-22-5921/rc).
Methods
Hydrogel preparation and characterization
The hydrogel was procured from ESI BIO Stem Cell Solutions (Alameda, CA, USA). The hydrogel was synthesized by thiolated gelatin (Gel-SH), thiolated hyaluronic acid (HA-SH), and polyethylene glycol diacrylate (PEGDA), with a ratio of 4:1:1.25. Swelling/deswelling tests were performed as we described previously (17).
Culture and transfection of the MSCs
The luc-BDNF lentiviral particles were purchase from Jikai company (Shanghai, China). The BDNF gene was amplified with 5'-AGATCTATGACCAT- CCTTTTCCTTAC sense primer and 5'-CTCGAGCTATCTTCCCCTTTTAATGG anti-sense primer. MSCs isolated from femurs of mice (C57BL/6J) were donated by Jiangsu Key Laboratory of Molecular and Functional Imaging, Department of Radiology, Zhongda Hospital. MSCs were grown in Dulbecco’s Modified Eagle Medium/F12 (DMEM/F12) from Cellgro (Manassas, VA, USA), along with 10% fetal bovine serum (FBS) from HyClone (Logan, UT, USA), and penicillin (50 U/mL) and streptomycin (0.05 mg/mL) from Sigma-Aldrich (St Louis, MO, USA). After MSCs were dissociated with 0.25% (w/v) trypsin (Gibco, Life Technologies, China) and pipetted multiple times, they were cultivated in serum-free DMEM/F12 containing sufficient luc-BDNF lentiviral particles with 5 mg/mL polybrene (Sigma-Aldrich). The medium was changed to a fresh full media after 6 hours, and the MSCs were passed 48 hours later. MSCs with BDNF and firefly luciferase genes were used in this study.
Photothrombotic model of focal ischemic stroke
C57BL/6J mice (male, 20–23 g, 8 weeks, Pengyue Experimental Animal Breeding Co., Ltd., Jinan, China) were used in this study. As we previously reported, a photothrombotic model of focal ischemic stroke was created (11). A 50 mg/kg intravenous dose of 1% pentobarbital was used to anesthetize the mice. To reveal the skull, a cut was made across the middle of the scalp. Five minutes following the administration of Rose Bengal (100 mg/kg, 1% in sterile saline, Sigma-Aldrich), a cold light source (KL1500 LCD, Zeiss, USA) was positioned. After 15 minutes of illumination, the mice were placed back inside their cage. Using a heating pad, the body temperature of the mice was sustained between 36.5 and 37.5 ℃ during the experiment.
Cell implantation
Three days after ischemic stroke induction, the cells were implanted. After anesthetized with 1% pentobarbital, mice were placed on a stereotaxic frame. After mixing Gel-SH, HA-SH, and PEGDA with a ratio of 4:1:1.25. 1×106 cells (BDNF-MSCs) or (MSCs) were re-suspended with 100 µL hydrogel. A Hamilton syringe was used to inject 3 µL hydrogel carrying BDNF-MSCs (1×107 cells/mL) or MSCs (1×107 cells/mL) into the brain at a rate of 0.2 µL·min-1 [anteroposterior (AP) =0, mediolateral (ML) =2.0 mm, dorsoventral (DV) =3.0 mm].
Quantitative polymerase chain reaction (qPCR) assay
TRIzol reagents (Promega, Shanghai, China) were used to extract RNA from cellular or cerebral tissue. Reverse transcription was carried out using a SYBR Green PCR kit from Applied Biosystems, China, and the qPCR test was performed using the ABI Prism7600 Fast Real-Time PCR equipment. The melting temperature of the product was determined using melting curve analysis. As the reference gene, glyceraldehyde-3-phosphate dehydrogenase (GAPDH) was consistently examined. We measured the relative messenger RNA (mRNA) expression using the 2−∆∆Ct method.
5-ethynyl-2’-deoxyuridine (EdU) assay
An EdU incorporation assay was carried out according to the manufacturer’s instructions (Abcam, Cambridge, UK).
Enzyme-linked immunosorbent assay (ELISA)
ELISA was carried out per the manufacturer's protocol (Elabscience Biotechnology, Wuhan, China).
Oxygen-glucose deprivation/reperfusion (OGD/R) test
The OGD/R procedure was performed as described previously (18,19). Briefly, complete medium was replaced by medium without glucose and FBS. MSCs and H-MSCs were first placed in an airtight chamber and allowed to acclimate for 10 minutes while continuously fed a gas flow composed of 95% N2 and 5% CO2. For a further 4 hours of OGD, the chamber was placed in an incubator after sealing. A complete medium was then used to reoxygenate cells in an environment that is normally aerobic. Mock cells were added to DMEM/F12 glucose medium that was normally oxygenated.
In vitro BLI
Cells were resuspended in PBS after being dissociated with trypsin (0.25%, w/v). Suspensions with various cell densities added to them were added to 24-well plates. Ten minutes after the addition of D-luciferin (0.15 mg/mL), the BLI of BDNF-MSCs was monitored, as described below.
In vivo BLI
BLI was performed using the Berthold LB 983 NC100 imaging system (Berthold Technologies, Bad Wildbad, Germany) after BDNF-overexpressing MSCs by hydrogel (H-B-MSCs) transplantation. Briefly, the BLI signal of mice was recorded 10 minutes after intraperitoneal injection of 100 mL D-luciferin (30 mg/mL). The radiance was calculated after drawing the region of interest (ROI) of each mouse.
Animal experiments were performed under a project license (No. AHQU-MAL20220805) granted by Ethical Review Committee of Animal Experimental Center of the Affiliated Hospital of Qingdao University, in compliance with Chinese guidelines for the care and use of animals. A protocol was prepared before the study without registration.
In vitro cell survival and necrosis assay
To test the vitality of the cells, the Cell Counting Kit-8 (CCK-8) assay (Dojindo Laboratories, Kumamoto, Japan) was used. At 450 nm, the CCK optical density (OD) was noted. A 2-step enzymatic reaction lactate dehydrogenase (LDH) assay kit (Takara, Tokyo, Japan) was used to examine the release of LDH to the conditioned medium in order to determine whether cell necrosis had occurred. The medium’s LDH concentration was adjusted to total LDH (medium LDH plus cellular LDH).
Western blot analysis
Radioimmunoprecipitation assay (RIPA) buffer (KeyGEN BioTECH, Nanjing, China) with 1% protease inhibitor cocktail (Roche Applied Sciences, Mannheim, Germany) was used to homogenize cells or brain tissues. Supernatants were collected, and the bicinchoninic acid (BCA) protein quantification kit (KeyGEN BioTECH, China) was used to calculate the protein content. After sodium dodecyl sulphate-polyacrylamide gel electrophoresis (SDS-PAGE) separation, the proteins were transferred to a polyvinylidene difluoride (PVDF) membrane (Bio-Rad Laboratories, Shanghai, China). After blocking with 5% milk at room temperature, the PVDF membrane was incubated with primary antibodies against BDNF (1:500 dilution, Abcam), cleaved PARP [Poly (ADP-ribose) polymerase] (1:1,000 dilution, Abcam), cleaved caspase 3 (1:2,000 dilution, Abcam), VEGF (1:500 dilution, Abcam), IGF-1 (0.2 mg/mL dilution, Abcam), β-actin (1:5,000 dilution, Abcam), and tubulin (1:10000 dilution, Abcam) overnight at 4 ℃. A horseradish peroxidase (HRP)-conjugated secondary antibody was then incubated with the PVDF membrane (1:5,000, Wuhan, China). The membrane was then exposed and examined using the Image-Pro Plus program (NIH, USA).
Immunohistochemical and immunofluorescence staining
Paraffin blocks were created after fixation in 4% paraformaldehyde at 4 ℃. Slices of the brain were taken from the block. The sections were treated with primary antibodies directed against myelin basic protein (MBP) (1:200 dilution, Abcam) and cluster of differentiation 31 (CD 31) (1:60 dilution, Abcam) for 2 hours at 37 ℃, followed by biotinylated secondary antibodies (1:200, Abcam) at room temperature for an additional 1 hour. The sections were then exposed to an avidin-biotin-peroxidase kit for 10 minutes at room temperature. A nickel-enhanced 3,3'-diaminobenzidine (DAB) peroxidase substrate kit was used to observe the HRP reaction result. For immunofluorescence staining, the sections were first treated with a primary antibody directed against neuronal nuclei (NeuN) (1:1,000 dilution; Abcam) for 2 hours at 37 ℃, followed by an hour at room temperature in the presence of a secondary antibody (1:1,000 dilution; Invitrogen, Darmstadt, Germany). The experimental groups were concealed from the observer who examined the data.
Behavioral tests
A modified neurological severity score (mNSS) was given, along with the foot fault test, 1 day prior to the production of a stroke and 3, 7, 14, and 21 days post-cell-transplantation. The mice were put on a makeshift wire grid and recorded for 5 minutes with a camera placed underneath the grid as part of the foot fault test. A foot fault was noted once the paw passed through a grid hole. The experimental groups were hidden from the observer who recorded the scores. The mNSS scores were collected as previously described (3).
Statistical analysis
The data are given as mean ± standard deviation (SD). All analyses were carried out at least 3 times. To examine the statistical differences between groups, two-tailed Student’s t-tests or one-way analysis of variance (ANOVA) with an post hoc least significant difference (LSD) test were used. Statistics were deemed significant at P<0.05.
Results
Rheological properties of hydrogel
Strain (Figure 1A) and frequency (Figure 1B) sweep tests were performed to understand the hydrogel’s characteristics better. The complex viscosity was in a high linear relationship with shear rate, and an increasing shear rate decreased the viscosity (Figure 1C). Following the addition of PEGDA, the hydrogel began to gel (Figure 1D).
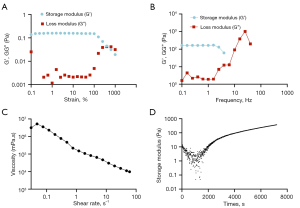
BDNF detection and in vitro BLI
To investigate BDNF expression of MSCs after transfection, western blot, qPCR, and ELISA assays were carried out. The results showed that BDNF expression was significantly increased after lentivirus transfection (Figure 2A-2C). In addition, EdU-positive cells were significantly increased after lentivirus transfection (Figure 2D,2E). We performed BLI of MSCs after lentivirus transfection in vitro to verify whether we could track MSCs with BLI after transfection and whether the BLI intensity directly represented the number of MSCs. The results indicated that there was a nearly linear relationship between the BLI intensity and the number of MSCs (R2=0.99) (Figure 3A,3B).
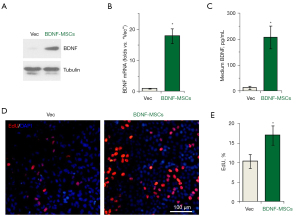
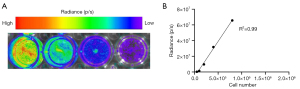
Hydrogel promotes the survival ability of MSCs in the OGD/R model
To confirm whether hydrogel could promote the survival of MSCs in the ischemic environment, OGD/R assay was performed. MSCs and H-MSCs were compared in the OGD/R model. Results demonstrated that there was a statistical difference between the 2 groups in the number of cells that survived in the OGD model (P<0.05) (Figure 4A). Additionally, the release of lactate dehydrogenase was substantially increased in the MSC group (P<0.05) (Figure 4B).
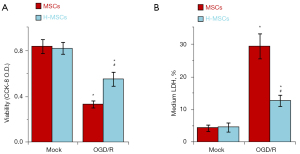
In vivo tracking of MSCs
To validate our ability to track BDNF-MSCs using BLI. We initially performed BLI in vivo at various time intervals. Three days following cell transplantation, we were able to detect a considerably greater BLI signal in the H-B-MSCs group, and the signal progressively diminished over time. The signal could still be observed at day 14 (Figure 5A,5B).
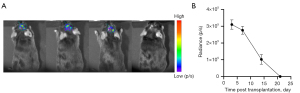
BDNF expression, inflammation, and necrosis on 3rd day of cell transplantation
On the 3rd day of cell transplantation, BDNF expression in the brain was detected. Results revealed that the level of BDNF in the H-B-MSCs group was substantially greater than in the other 2 groups (P<0.05) (Figure 6A-6C). H-B-MSCs transplantation significantly inhibited TNF-α and IL-1 expression (P<0.05) (Figure 6D,6E). In addition, cleaved PARP and cleaved caspase-3 decreased in the H-B-MSCs group (P<0.05) (Figure 6F-6H).
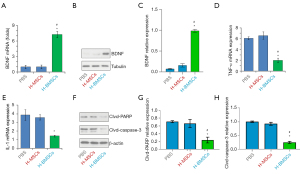
Increase in neurogenesis, angiogenesis, and white matter recovery by H-B-MSCs after ischemic stroke
On the 7th day post-cell-transplantation, there was a higher VEGF level (Figure 7A,7B), and IGF-1 (Figure 7C,7D) was detected in the H-B-MSCs group (P<0.05). H-B-MSCs significantly increased the expression of NeuN (Figure 8A,8B). The H-B-MSCs group showed more MBP expression than the other groups did (Figure 8C,8D). Additionally, we used the marker CD 31 to assess angiogenesis at day 7, and the results revealed that H-B-MSCs therapy greatly increased the microvessel density (Figure 8E,8F).
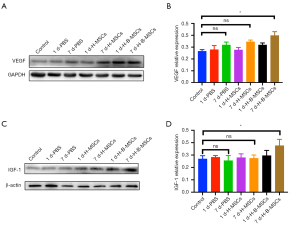
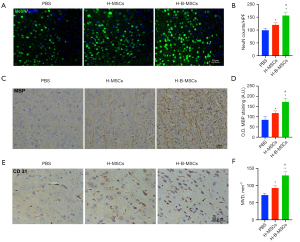
Functional recovery improvement by H-B-MSCs treatment after ischemic stroke
According to the mNSS and foot fault tests, mice treated with H-B-MSCs had significantly fewer neurological functional abnormalities than the other 2 groups (Figure 9A,9B).
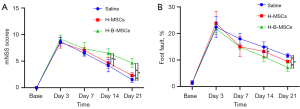
Discussion
Our results showed that we had successfully constructed an MSC delivery system with hydrogel, and that prolonged survival of transplanted BDNF-overexpressing MSCs delivered by the hydrogel could promote ischemic stroke recovery.
Stem-cell-based therapies bring considerable hope for ischemic stroke recovery. Unfortunately, acute cell death posttransplantation limits the clinical application of stem cell therapy (15,20). Biomaterials offer a suitable environment for cells after transplantation (21). In this study, we explored the feasibility of hydrogel carrying BDNF-overexpression MSC for ischemic stroke treatment.
BLI has been widely used to dynamically track cell fate after transplantation as the signal cannot be detected in dead cells (22,23). In the present study, the BLI signal could be detected in the H-B-MSCs group even at 14 days after cell transplantation, indicating a long survival period for stem cells. Our lab had synthesized a biocompatible hydrogel during previous work (24). In the present study, our results suggested that the hydrogel achieved good biocompatibility in MSC delivery. In addition, luc-BDNF lentivirus transfection could promote the proliferation of MSCs. To confirm whether hydrogel could promote the survival of MSCs in the ischemic environment, the OGD/R test was carried out. The results indicated that hydrogel could significantly promote the survival of the MSCs and suggested that MSCs delivered by hydrogel may have, to a large extent, overcome the acute cell death after cell transplantation into the ischemic area. As previous studies have reported, MSCs may promote the recovery of ischemic stroke via neuroprotection, neurogenesis, angiogenesis, and white matter recovery (25,26). Three days after cell transplantation, less inflammation response was found in the H-B-MSCs group, as indicated by the expression levels of interleukin-1 (IL-1) and tumor necrosis factor-α (TNF-α). Further, H-B-MSCs decreased the necrosis of the brain at day 3 after cell transplantation, as indicated by the expression levels of cleaved PARP and cleaved caspase-3. A previous study reported that MSCs could increase the BDNF level of the brain after transplantation (8). However, in our study, no statistically significant was found in BDNF expression between H-MSCs and PBS group at day 3 after transplantation. The photothrombotic model of ischemic stroke and the detection time point at day 3 after cell transplantation may be the reason that accounting for the differences.
Seven days after transplantation, significantly increased expression of VEGF and IGF-1 were observed in the H-B-MSCs group. The level of these factors has been reported to positively correlate with recovery after ischemic stroke (27,28). To further confirm the therapeutic efficacy of H-B-MSCs, neurogenesis, white matter recovery, angiogenesis, and function behavior were detected at different times. NeuN expression was significantly higher in the H-B-MSCs group on day 14 after cell transplantation, indicating that H-B-MSCs could significantly increase neurogenesis compared with the other 2 groups. We also detected the expression of CD 31, with the results indicating that H-B-MSCs could also significantly promote angiogenesis compared with the other 2 groups. White matter is considered important for sensory, motor, and cognitive functions and constitutes almost 50% of the brain (29,30). A previous study have reported that white matter repair is important for recovery after ischemic stroke (31). We found that MBP expression was higher in the H-B-MSCs group, indicating that myelinated axons significantly increased after H-B-MSCs transplantation, reflecting improved remyelination after the stroke.
A number of studies have established that mNSS and foot fault tests are robust for evaluating functional recovery after stroke (11,32,33). Our study also conducted these tests, and the results revealed that H-B-MSCs could significantly promote functional recovery compared with the other 2 groups at day 14 and day 21 after cell transplantation. All of the results above indicated that H-B-MSCs could significantly promote recovery after ischemic stroke.
Conclusions
We effectively established a robust a hydrogel delivery system. Transplanted BDNF-MSCs with hydrogel offered a mechanism for continuous BDNF release, which promoted recovery after ischemic stroke.
Acknowledgments
Funding: This work was supported by the National Science Foundation for Youths of China (No. 81901800), Foundation of Taishan Scholars Project. China Postdoctoral Science Foundation (No. 2021M701812), Science and Technology Plan of Shinan District of Qingdao (No. 2022-2-001-YY), and Qingdao Postdoctoral Applied Research Project.
Footnote
Reporting Checklist: The authors have completed the ARRIVE reporting checklist. Available at https://atm.amegroups.com/article/view/10.21037/atm-22-5921/rc
Data Sharing Statement: Available at https://atm.amegroups.com/article/view/10.21037/atm-22-5921/dss
Conflicts of Interest: All authors have completed the ICMJE uniform disclosure form (available at https://atm.amegroups.com/article/view/10.21037/atm-22-5921/coif). The authors have no conflicts of interest to declare.
Ethical Statement: The authors are accountable for all aspects of the work in ensuring that questions related to the accuracy or integrity of any part of the work are appropriately investigated and resolved. Animal experiments were performed under a project license (No. AHQU-MAL20220805) granted by Ethics Review Committee of Animal Experimental Center of the Affiliated Hospital of Qingdao University, in compliance with Chinese guidelines for the care and use of animals.
Open Access Statement: This is an Open Access article distributed in accordance with the Creative Commons Attribution-NonCommercial-NoDerivs 4.0 International License (CC BY-NC-ND 4.0), which permits the non-commercial replication and distribution of the article with the strict proviso that no changes or edits are made and the original work is properly cited (including links to both the formal publication through the relevant DOI and the license). See: https://creativecommons.org/licenses/by-nc-nd/4.0/.
References
- Li J, Zhang Q, Wang W, et al. Mesenchymal stem cell therapy for ischemic stroke: A look into treatment mechanism and therapeutic potential. J Neurol 2021;268:4095-107.
- Go AS, Mozaffarian D, Roger VL, et al. Heart disease and stroke statistics--2014 update: a report from the American Heart Association. Circulation 2014;129:e28-e292.
- Wang C, Lin G, Luan Y, et al. HIF-prolyl hydroxylase 2 silencing using siRNA delivered by MRI-visible nanoparticles improves therapy efficacy of transplanted EPCs for ischemic stroke. Biomaterials 2019;197:229-43.
- Pittenger MF, Mackay AM, Beck SC, et al. Multilineage potential of adult human mesenchymal stem cells. Science 1999;284:143-7.
- Prockop DJ. Marrow stromal cells as stem cells for nonhematopoietic tissues. Science 1997;276:71-4.
- Petersen BE, Bowen WC, Patrene KD, et al. Bone marrow as a potential source of hepatic oval cells. Science 1999;284:1168-70.
- Li X, Huang M, Zhao R, et al. Intravenously Delivered Allogeneic Mesenchymal Stem Cells Bidirectionally Regulate Inflammation and Induce Neurotrophic Effects in Distal Middle Cerebral Artery Occlusion Rats Within the First 7 Days After Stroke. Cell Physiol Biochem 2018;46:1951-70.
- Wakabayashi K, Nagai A, Sheikh AM, et al. Transplantation of human mesenchymal stem cells promotes functional improvement and increased expression of neurotrophic factors in a rat focal cerebral ischemia model. J Neurosci Res 2010;88:1017-25.
- Mukai T, Tojo A, Nagamura-Inoue T. Umbilical Cord-Derived Mesenchymal Stromal Cells Contribute to Neuroprotection in Neonatal Cortical Neurons Damaged by Oxygen-Glucose Deprivation. Front Neurol 2018;9:466.
- Chen X, Li Y, Wang L, et al. Ischemic rat brain extracts induce human marrow stromal cell growth factor production. Neuropathology 2002;22:275-9.
- Wang C, Zhang Y, Ding J, et al. Nicotinamide Administration Improves Remyelination after Stroke. Neural Plast 2017;2017:7019803.
- Kurozumi K, Nakamura K, Tamiya T, et al. BDNF gene-modified mesenchymal stem cells promote functional recovery and reduce infarct size in the rat middle cerebral artery occlusion model. Mol Ther 2004;9:189-97.
- Burdick JA, Mauck RL, Gerecht S. To Serve and Protect: Hydrogels to Improve Stem Cell-Based Therapies. Cell Stem Cell 2016;18:13-5.
- Orive G, Anitua E, Pedraz JL, et al. Biomaterials for promoting brain protection, repair and regeneration. Nat Rev Neurosci 2009;10:682-92.
- Lee AS, Inayathullah M, Lijkwan MA, et al. Prolonged survival of transplanted stem cells after ischaemic injury via the slow release of pro-survival peptides from a collagen matrix. Nat Biomed Eng 2018;2:104-13.
- Doerks T, Copley RR, Schultz J, et al. Systematic identification of novel protein domain families associated with nuclear functions. Genome Res 2002;12:47-56.
- Qi X, Xiang Y, Cai E, et al. All-in-one: Harnessing multifunctional injectable natural hydrogels for ordered therapy of bacteria-infected diabetic wounds. Chemical Engineering Journal 2022;439:135691.
- Ye XC, Hao Q, Ma WJ, et al. Dectin-1/Syk signaling triggers neuroinflammation after ischemic stroke in mice. J Neuroinflammation 2020;17:17.
- Shan Y, Tan S, Lin Y, et al. The glucagon-like peptide-1 receptor agonist reduces inflammation and blood-brain barrier breakdown in an astrocyte-dependent manner in experimental stroke. J Neuroinflammation 2019;16:242.
- Nguyen PK, Neofytou E, Rhee JW, et al. Potential Strategies to Address the Major Clinical Barriers Facing Stem Cell Regenerative Therapy for Cardiovascular Disease: A Review. JAMA Cardiol 2016;1:953-62.
- Discher DE, Mooney DJ, Zandstra PW. Growth factors, matrices, and forces combine and control stem cells. Science 2009;324:1673-7.
- Li S, Ruan Z, Zhang H, et al. Recent achievements of bioluminescence imaging based on firefly luciferin-luciferase system. Eur J Med Chem 2021;211:113111.
- Mezzanotte L, van 't Root M, Karatas H, et al. In Vivo Molecular Bioluminescence Imaging: New Tools and Applications. Trends Biotechnol 2017;35:640-52.
- Liang Y, Bar-Shir A, Song X, et al. Label-free imaging of gelatin-containing hydrogel scaffolds. Biomaterials 2015;42:144-50.
- Guo Y, Peng Y, Zeng H, et al. Progress in Mesenchymal Stem Cell Therapy for Ischemic Stroke. Stem Cells Int 2021;2021:9923566.
- Li W, Shi L, Hu B, et al. Mesenchymal Stem Cell-Based Therapy for Stroke: Current Understanding and Challenges. Front Cell Neurosci 2021;15:628940.
- Sohrabji F, Williams M. Stroke neuroprotection: oestrogen and insulin-like growth factor-1 interactions and the role of microglia. J Neuroendocrinol 2013;25:1173-81.
- Huang L, Zhang L. Neural stem cell therapies and hypoxic-ischemic brain injury. Prog Neurobiol 2019;173:1-17.
- Nave KA. Myelination and support of axonal integrity by glia. Nature 2010;468:244-52.
- Bercury KK, Macklin WB. Dynamics and mechanisms of CNS myelination. Dev Cell 2015;32:447-58.
- Goldberg MP, Ransom BR. New light on white matter. Stroke 2003;34:330-2.
- Bai YY, Gao X, Wang YC, et al. Image-guided pro-angiogenic therapy in diabetic stroke mouse models using a multi-modal nanoprobe. Theranostics 2014;4:787-97.
- HoWangYin KY. HIF-prolyl hydroxylase 2 inhibition enhances the efficiency of mesenchymal stem cell-based therapies for the treatment of critical limb ischemia. Stem Cells 2014;32:231-43.
(English Language Editor: A. Muijlwijk)