Neuroprotective effects of nobiletin on cerebral ischemia/reperfusion injury rats by inhibiting Rho/ROCK signaling pathway
Highlight box
Key findings
• Our study proves that Nobiletin to reduce the cerebral infarct area, improve neurological deficits, enhance learning and memory ability, and reduce neuron apoptosis and level of inflammatory factors (IL-6, IL-1, TNF).
What is known and what is new?
• Stroke is a common neurological disease characterized by high rates of incidence, death, disability and recurrence.
• Nobiletin had a neuroprotective effect in rats with cerebral I/R injury.
What is the implication, and what should change now?
• Nobiletin is a promising neuroprotective agent for patients with cerebral ischemia.
Introduction
Stroke is a common neurological disease characterized by high rates of incidence, death, disability and recurrence (1). With approximately 80 million stroke cases worldwide, it contributes to the global health economic burden. Stroke is classified as either ischemic or hemorrhagic, of which ischemic stroke is the most common, accounting for »60–80% of all strokes and causing ischemia and hypoxia of brain tissue (2,3). Currently, the effective treatment for ischemic stroke is to reperfuse the ischemic brain tissue by thrombolysis, but outside of the narrow therapeutic time window, it can cause reperfusion damage to the brain tissue (4-6). Numerous preclinical trials have shown that neuroprotective agents can improve the success rate of treatment for ischemic stroke, both when given early in the ischemic stroke episode to prolong the time window of intravenous or intra-arterial treatment and when used during or after partial or complete reperfusion to reduce reperfusion injury and thus reduce the likelihood of disability in stroke patients after treatment (7,8). Therefore, investigating effective neuroprotective agents is crucial to improving the treatment success rate for patients with ischemic stroke.
neuron apoptosis in the ischemic core region is an essential factor affecting neurological recovery in patients with ischemic stroke, and a series of responses such as oxidative stress, excitatory amino acids, Ca2+ overload, and inflammatory responses induced by reperfusion can accelerate neuron apoptosis and further hinder neurological recovery (9,10). Therefore, reducing neuron apoptosis is key to neurological recovery. Previous studies have shown that the Rho/Rho-associated kinase (ROCK) signaling pathway mediated by Rho GTPase and its downstream target effector ROCK are closely related to neuron apoptosis and inhibition of axonal growth. Inhibition of the Rho/ROCK signaling pathway in vivo can promote axonal regeneration, and Rho and ROCK play a key role in the pathogenesis of stroke, they are expressed in all cell types associated with stroke and regulate a series of physiological processes (11,12). So actively exploring blockers of the Rho/ROCK signaling pathway is crucial to improving treatment of ischemic brain injury.
Nobiletin (NOB) is an active natural flavonoid that is mainly distributed in the stems, leaves and peels of Rutaceae and Euphorbiaceae, with a variety of important biological activities that are utilized in Traditional Chinese Medicine, such as anti-inflammatory, antioxidative, antitumor and neuroprotective (13-16). Recent studies have reported on NOB for the treatment of neurological disorders, such as improving cognitive function and reducing the plaque and oxidative stress caused by β-amyloid accumulation in animal models of Alzheimer’s disease, and improvement of motor function and cognitive deficits in animal models of Parkinson’s disease (17,18). In addition, NOB can reduce the production of mitochondrial reactive oxygen species, inhibit apoptotic signaling, enhance ATP production, and improve neuronal cell viability (19). Study of ischemic encephalopathies have shown that NOB prevents ischemia-hypoxia-induced brain injury by activating the Akt/CREB signaling pathway and improving the permeability of the blood-brain barrier (20).
However, the precise pharmacological effect of NOB on ischemic brain injury and its potential mechanism requires further validation. In this study, we simulated cerebral ischemia/reperfusion (I/R) injury by surgically occluding the middle cerebral artery in rats [middle cerebral artery occlusion (MCAO) model], and treated them with NOB. Results showed that NOB significantly decreased the cerebral infarct area, improved neurological deficits, reversed neuron apoptosis and reduced the levels of inflammatory factors interleukin (IL)-6, IL-1β and tumor necrosis factor (TNF)-α in rats with cerebral I/R injury. In terms of the mechanism, the results showed that NOB inhibited the activity of the Rho/ROCK signaling pathway in brain tissue with ischemic injury, and the neuroprotective effect of NOB via inhibiting the Rho/ROCK signaling pathway was confirmed using an oxygen-glucose deprivation PC12 (OGD PC12) cell model. The present study adds reliable data to confirm the neuroprotective effect of NOB and further elucidates its mechanism as a potential treatment for ischemic stroke. We present the following article in accordance with the ARRIVE reporting checklist (available at https://atm.amegroups.com/article/view/10.21037/atm-22-6119/rc).
Methods
Animals and MCAO model
A total of 60 adult male Sprague-Dawley rats (180–200 g, 6–8 weeks) were purchased from Shanghai Jihui Laboratory Animal Care Co., Ltd. [Shanghai, China; Animal Production License No. SCXK (Shanghai) 2017-0012 and Animal Use Certificate No. 20170005053051]. Food and water were provided under specific pathogen-free conditions with a relative humidity of 40–70%, ambient temperature of 20–25 ℃, and a 12/12 h light/darkness cycle. A protocol was prepared before the study without registration. Animal experiments were performed under a project license (No. Med-Eth-Re[2022]734) granted by the Ethics Committee of Hainan General Hospital, in compliance with institutional guidelines for the care and use of animals.
The rats were randomly divided into four groups: Sham group (treated with an equal volume of solvent, N=15), MCAO model group (treated with an equal volume of solvent, N=15), NOB high-dose group (MCAO rats treated with 20 mg/kg NOB, N=15), and NOB low-dose group (MCAO rats treated with 10 mg/kg NOB, N=15) (20). NOB was purchased from Chengdu Herbpurify Co., Ltd. (Chengdu, China), with a purity of 98%. Rats were intragastric administered once a day for 7 days before cerebral ischemia and again after reperfusion.
The MCAO model was created according to a previously described protocol (21). Rats were anesthetized by intraperitoneal injection of 3% pentobarbital sodium (30 mg/kg, Sigma-Aldrich, CA, USA), and the neck skin was prepared and disinfected for an incision to separate the right common carotid artery, external carotid artery and internal carotid artery before ligation of the distal external carotid artery as follows. The proximal common carotid artery and internal carotid artery were temporarily clamped with arterial clips, and the external carotid artery was perforated near the bifurcation of the common carotid artery for insertion of a nylon thread with a smooth spherical tip (0.25 mm in diameter). The external carotid artery segment was loosely ligated at the perforation, the arterial clamp on the internal carotid artery was partially released and the thread was gently directed from the external carotid artery into the internal carotid artery for »18.5±0.5 mm. The segment of the external carotid artery and the thread were again ligated together until the beginning of the middle cerebral artery was occluded. Then the clamp on the common carotid artery was released and the neck skin was sutured. The thread was removed to restore blood perfusion after 2 h of ischemia.
Modified neurological severity score (mNSS)
The mNSS was used to assess the neurological deficit in four aspects (motor, sensory, balance and reflexes) 24 h after cerebral ischemia-reperfusion. As reported in our previous research (21), the total score for the mNSS is 18 points, with higher scores representing more severe neurological deficits (22).
Morris water maze (MWM) testing
As per the experimental conditions and environments described in our previous study (20), the MWM was used to examine the learning and memory abilities of the rats in the MCAO model. The rats underwent a positioning navigation experiment before the MCAO by first placing them on the platform for 20 s and then in the water while placing the platform in any quadrant to record the time it took for the rat to find the platform (up to 60 s). The training was performed 4 times a day for 4 consecutive days, with 15 min between each session. After 24 h of reperfusion, the platform was placed 2 cm underwater. The learning ability of the rats was evaluated by calculating the time to find the platform (escape latency) and swimming distance (escape path length) using the video tracking system (SuperMaze Morris water maze experimental analysis system, Shanghai XinRuan Information Technology Co., Ltd., Shanghai, China).
TTC staining
After 24 h of cerebral ischemia–reperfusion the rats were anesthetized again with intraperitoneal pentobarbital sodium. After opening the thorax, the right auricle was incised, and the whole brain was removed after perfusion with phosphate-buffered saline (PBS) from the left ventricle. Each rat brain was frozen (−20 ℃, 30 min) and then sliced into five pieces, placed in 2% TTC solution (Sigma-Aldrich), incubated for 30 min at 37 ℃, and then fixed in 4% formaldehyde for 24 h. The cerebral infarct area was measured using a high-resolution pathological image analysis system (Champion Image HPIAS-1000, Champion Image Engineering Company of Tongji Medical University, Wuhan, China), and the infarct volume was calculated according to the formula V = t (A1+... + An) – (A1+An) t/2, where T is the slice thickness and A is the infarct area.
Enzyme-linked immunosorbent assay (ELISA)
For the ELISA, 100 mg of ischemic brain tissue was washed with PBS, and homogenized with frozen RIPA buffer containing protease and phosphatase inhibitors. The homogenate was centrifuged at 5,000 g at 4 ℃ for 10 min, and then the supernatant was collected to determine levels of IL-6, IL-1β, and TNF-α according to the manufacturer’s instructions (R&D Systems, MN, USA).
Histopathological examination
Ischemic cerebral tissue was fixed with 4% paraformaldehyde (Sigma-Aldrich) for 4 days, trimmed to the appropriate shape and thickness, dehydrated, cleared, and embedded in wax, before sectioning to a thickness of 4 µm, and immersed in hematoxylin staining solution (Sigma-Aldrich) for 5 min at room temperature. Sections were then washed with ultrapure water for 1 min before being dewaxed and rehydrated, immersed in 1% hydrochloric acid alcohol solution for 30 s and rinsed with ultrapure water until the tissue turned blue. Next, the sections were immersed in eosin staining solution (Sigma-Aldrich) for 3–5 min, and excess color was rinsed off with ultrapure water. After the samples were dehydrated, cleared, and sealed with neutral gum, the nuclei of neuron were observed under a microscope (Olympus Co., Ltd., Japan).
Terminal transferase-mediated dUTP nick-end labeling (TUNEL) staining
After being prepared for histopathological examination, the brain tissue sections were routinely dewaxed, treated with Proteinase K working solution, and washed three times with PBS to prepare for the TUNEL reaction mix according to the manufacturer’s instructions (Roche Diagnostics, Shanghai, China). Each section was added to 5 µL of TdT and 45 µL of fluorescein-labeled dUTP mix at 37 ℃ for 60 min and then washed three times with PBS for, before being added to 50 µL of converter-POD at 37 ℃ for 30 min. After washing with PBS, an appropriate amount (full coverage of samples) of DAB substrate was added to control color development under the microscope. The sections were restained with hematoxylin for 30 s, washed with ultrapure water, and sealed with neutral gum. To observe the distribution of positive cells under the microscope, we were randomly selected five fields of view for each sample under a ×200 microscope (Olympus Co., Ltd., Japan), and the number of positive cells in each sample was counted.
Nissl staining
The cortex and hippocampus of each ischemic brain were fixed, embedded and sectioned as described, and then stained according to the manufacturer’s instructions (Beijing Solarbio Science & Technology Co., Ltd., Beijing, China). First, the sections were placed in a crystal violet staining solution, and the dye vat was placed in an incubator at 56 ℃ for 1 h. After being rinsed with deionized water, the sections were removed and placed in Nissl differentiation solution for a few seconds to 2 min, and then dehydrated quickly with absolute ethanol. The sections were cleared and sealed with neutral gum. Nissl bodies are purple under the microscope, and the background was close to colorless or light blue. Five fields of view were randomly selected for each sample under a ×200 microscope, and the total number of Nissl bodies in the cortex and hippocampus of each sample was calculated.
Immunohistochemistry
The wax-embedded brain tissue sections were dewaxed in xylene, rehydrated in gradient alcohol, and then incubated with 3% H2O2 at 37 ℃ for 15 min to block and inactivate endogenous peroxidase, then washed in PBS. The antigen was repaired by boiling in 0.01 M citrate buffer (pH 6.0) for 10 min and cooled naturally to room temperature. After washing three times with PBS, Rho, Rac 1, ROCK 1, ROCK 2, rabbit anti-GAPDH (1:200, BIOSS, Beijing, China) were added dropwise to each section before incubating overnight at 4 ℃ in the refrigerator and equilibrating at room temperature for 30 min. Next, the secondary antibody (Zhongshan Golden Bridge Biological Technology Co., Ltd., Beijing, China) was added dropwise before incubating for 30 min at 37 ℃. Microscopic images were obtained after PBS washing, DAB reaction staining, hematoxylin restaining, and neutral gum sealing. Five fields of view were randomly selected for each sample under a ×200 microscope, and the optical density values of relative protein expressions were calculated using ImagePro Plus software.
Real-time polymerase chain reaction (PCR)
Total RNA of the samples was extracted by the Trizol-centrifuge column method according to the instructions of the RNA Rapid Extraction Kit (Generaybiotech Co., Ltd., Beijing, China). After RNA purity determination and quantification, reverse transcription into cDNA was performed according to the manufacturer’s instructions (HiScript-II Q RT SuperMix for qPCR, Vazyme Biotech Co., Ltd., Nanjing, China). Real-time PCR (qPCR) was performed using the CFX Connect Real-Time PCR System (Bio-RAD, CA, USA), and the PCR amplification reaction system was as follows: 10 µL 2× ChamQ SYBR Color qPCR Master Mix, 0.6 µL forward primer (10 µM), 0.6 µL reverse primer (10 µM), 8.8 µL template cDNA, and 20 µL dd H2O. The reaction steps were as follows: 1 min at 95 ℃, 10 s at 95 ℃, 10 s at 58 ℃, and 10 s at 70 ℃, for a total of 40 cycles. The qPCR primers were synthesized and purified by Shanghai Sunny Biotechnology Co., Ltd., China and the primer sequences are as follows Table 1. The results were analyzed by the 2−ΔΔCt method, and the relative RNA expression values were calculated using Actin as an internal reference.
Table 1
Gene | Forward | Reverse |
---|---|---|
Actin | CCCATCTATGAGGGTTACGC | TTTAATGTCACGCACGATTTC |
Rho | GCCAAAATGAAGCAGGAGCC | TTCACAAGATGAGGCACCCC |
Rac 1 | TATGACAGACTGCGTCCCCT | GCTTCGTCCCCACTAGGATG |
ROCK 1 | GGTTGAACTTGCTTTCCGCT | GCATCCAATCCATCCAGCAA |
ROCK 2 | CCAAACAAACCAAGCTAACTGC | TTCAGTCTTGTGGCTGGAAGAA |
Cell culture and oxygen-glucose deprivation (OGD)
PC12 cells were purchased from the Institute of Biochemistry and Cell Biology at the Chinese Academy of Sciences (Shanghai, China) and cultured in DMEM (Gibco, NY, USA) containing 10% fetal bovine serum (Gibco) and 1% penicillin (Gibco) at 37 ℃ in an incubator with 5% CO2.
OGD/R-PC12 cells: the complete culture medium was removed and the PC12 cells cultured for 24 h were washed twice with sugar-free DMEM, then incubated with sugar-free DMEM in a closed anoxic chamber with 5% CO2 and 95% N2 at 37 ℃ for 2 h. The cells were then switched to a complete culture medium, and incubated in an incubator at constant temperature of 37 ℃ with 5% CO2 for 24 h. When PC12 cells were treated with NOB or NOB + pentanoic acid (PA, Med Chem Express, NJ, USA) after oxygen-glucose deprivation, MTT assay, flow cytometry and cell migration assay were performed.
Flow cytometry (FCM)
For FCM, 2×105 PC12 cells were centrifuged, collected, and washed in PBS three times. Annexin V-FITC binding solution was added first to resuspend the cells, followed by Annexin V/FITC and PI (Annexin V-FITC Detection Kit, Beyotime Biotechnology, Shanghai, China). The cells were incubated for 20 min at room temperature and apoptosis was detected by FCM (Accuri C6, Becton-Dickinson, CA, USA) under light-protected conditions.
MTT assay and cell migration assays
MTT assay: PC12 cells were seeded in 96-well plates (5.0×103 cells/well), and treated with NOB for 24 h after OGD. Next, 10 µL of MTT working solution (5 mg/mL, Beyotime Biotechnology) was added and the incubation was continued for 4 h, after which the culture medium was discarded. Next, 150 µL DMSO was added to each well, and the absorbance (OD) value at 490 nm was measured by an enzyme marker (Mini-Protein Tetra System, Bio-RAD).
Migration assays: PC12 cells were collected, washed twice in PBS and resuspended with serum-free DMEM. The cell density was then adjusted to 5×105 cells/mL. Transwell (Sigma-Aldrich) was homogenized with 200 µL of cell suspension in the upper chamber and 600 µL of DMEM containing 10% FBS in the lower chamber. After processing with OGD, the cells were treated with NOB for 24 h. The cells in the upper chamber were removed with cotton swabs, and fixed with paraformaldehyde for 15 min after being rinsed with PBS, and then stained with 0.1% crystal violet staining solution (Sigma-Aldrich) for 10 min. After washing three times with PBS, microscopic images of the migrating cells stained with crystal violet were obtained and the number was counted.
Western blot analysis
PC12 cells were collected and lysed with RAPI lysis solution (Beyotime Biotechnology). The samples were centrifuged at 25,000 g for 30 min at 4 ℃, and the supernatant was collected for measurement of the total protein by BCA kit (Beyotime Biotechnology). Protein samples were denatured and electrophoresed on a 10% SDS-PAGE gel, then transferred to a PVDF membrane (Millipore, MA, USA). The samples were sealed with 5% skim milk for 2 h and washed three times with TBST buffer solution. Next, Rho A, Rac 1, ROCK 1, ROCK 2 and rabbit anti-GAPDH (1:1,000, BIOSS ) were added according to the corresponding protein size (kDa) and incubated overnight at 4 ℃. The secondary antibody (goat anti-mouse IgG, 1:5,000, MultiSciences, Shanghai, China) was added after washing with TBST and the samples were incubated for 2 h at room temperature. After TBST washing, ECL luminescent solution (Beyotime Biotechnology) was added and a gel imager (ChemiDoc XRS+ System, Bio-RAD) was used to develop images. The relative protein expressions of Rho A, Rac 1, ROCK 1 and ROCK 2 were calculated using GAPDH as an internal reference.
Statistical analysis
The statistical analysis was performed using GraphPad Prism 8.0 (Graphpad Software Inc., San Diego, CA, USA). Data are expressed as the mean ± standard deviation. One-way analysis (one-way ANOVA) was used for comparison between groups, followed by the least significant difference method test. A P value <0.05 was considered statistically significant.
Results
NOB reduced cerebral infarct area and promoted neurological recovery in rats with cerebral I/R injury
We used TTC staining to observe the infarct area in cerebral I/R injured rats to investigate whether NOB has a neuroprotective effect. As shown in Figure 1A,1B, large cerebral infarcts occurred in rats of the MCAO groups, but were reduced in area in the NOB high-dose treatment group (20 mg/kg) although the NOB low-dose treatment group (10 mg/kg) showed no statistical difference (P>0.05). Regarding the mNSS results, the mNSS score was significantly higher in the untreated MCAO group but lower in the NOB-treated group, indicating an improvement with NOB (Figure 1C). To further confirm that NOB improved neurological function in cerebral I/R injury rats, we conducted the MWM experiment. The results showed that the swimming distance and latency time to find the platform of rats in the MCAO group were higher than those in the Sham group, the swimming distance of rats in the NOB high-dose treatment group was shorter than that in the untreated MCAO group, and the latency time of the NOB low-dose treatment group was shorter than that of the MCAO group (Figure 1D,1E). These results suggested that NOB had improved the learning and memory ability of cerebral I/R injury rats. We also explored the effect of NOB on inflammatory factors in the brain tissue of cerebral I/R injured rats. As shown in Figure 1F, the levels of IL-6, IL-1β, and TNF-α were significantly increased after cerebral I/R in rats, but when treated with NOB, the levels were significantly decreased.
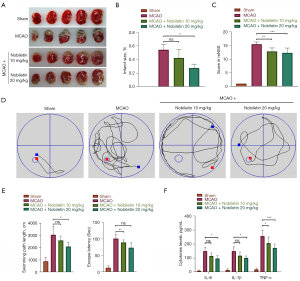
NOB reduced neuron apoptosis in cerebral I/R injury rats
neuron apoptosis is an essential manifestation of ischemic stroke, affecting the neurological recovery and prognosis of patients (23), so we examined it in cerebral I/R injury rats. First, we performed histopathological HE staining, which showed that compared with the Sham group, karyopyknosis, degeneration and apoptosis of a large number of neurons occurred in rats of the MCAO group, and were also seen in the NOB-treated group, but significantly less than in the MCAO group (Figure 2A). The same results were also obtained in the TUNEL staining experiment, and the degree of neuron apoptosis in the NOB high- and low-dose treatment groups was significantly less than that of the MCAO group (Figure 2B,2C). This finding demonstrated that NOB significantly reduced neuron apoptosis. To further verify these results, Nissl staining was used to observe the numbers of Nissl bodies in the cerebral cortex and hippocampus of cerebral I/R injury rats. The total number of Nissl bodies in the cortex and hippocampus was higher in the NOB-treated group than in the MCAO group (Figure 2D,2E), indicating an increase in the number of surviving neurons in the cortex and hippocampus after NOB treatment and suggesting that NOB could reduce the apoptosis of neurons in cerebral I/R injury rats.
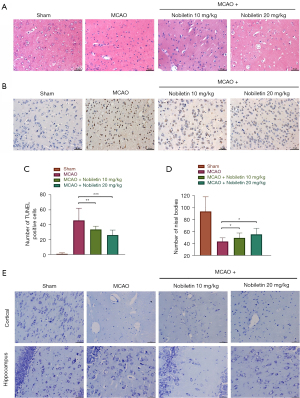
NOB inhibited the expression of the Rho/ROCK signaling pathway in the brain tissue of cerebral I/R injury rats
Because activation of Rho and its effector ROCK is a critical factor in inhibiting axonal growth, and blocking the Rho/ROCK pathway can promote neuron regeneration (11), we explored whether NOB could inhibit the Rho/ROCK signaling pathway in cerebral I/R injury rats. The expression levels of Rho A, Rac 1, ROCK 1, and ROCK 2, proteins related to the Rho/ROCK signaling pathway, were first analyzed using immunohistochemistry, and the results are shown in Figure 3A,3B. Compared with the Sham group, the expression levels of Rho A, Rac 1, ROCK 1, and ROCK 2 were elevated in the ischemic reperfused brain tissue of rats in the MCAO group, but were significantly decreased after treatment with NOB. To validate these results, we extracted the RNA in brain tissue, and the RNA expression levels of Rho A, Rac 1, ROCK 1, and ROCK 2 in the brain tissue of rats in the MCAO group were higher than those in the Sham group, while the expression levels were reversed in the NOB high- and low-dose treatment groups (Figure 3C). Thus, the results suggested that NOB inhibited the activity of the Rho/ROCK signaling pathway in cerebral I/R injury rats.
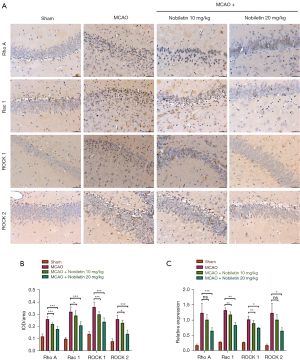
NOB protected OGD PC12 cells by inhibiting the Rho/ROCK signaling pathway
To further elucidate whether the mechanism of the neuroprotective effect of NOB is through inhibition of the Rho/ROCK signaling pathway, we established an OGD model of PC12 cells in vitro, examining the apoptosis, migration, cell viability and expression levels of related proteins after treatment of OGD PC12 cells with NOB, PA (an Rho/ROCK pathway agonist) (24,25) or NOB + PA. As shown in Figure 4, the results revealed that the rate of apoptosis of PC12 cells in the NOB-treated group was less than that in the OGD model group. When treated with PA, apoptosis of OGD PC12 cells was accelerated, whereas treatment with NOB reduced PA-induced apoptosis. Similarly, in the cell migration and MTT assays, we also observed that the number of migrating PC12 cells and cell viability were higher in the NOB-treated group than in the OGD model group, and also higher in the NOB combined with PA-treated group than in the PA-treated group (Figure 4B,4D,4E). In addition, the western blotting results (Figure 4F,4G) showed that the expression levels of Rho A, Rac 1, ROCK 1, and ROCK 2 in the NOB-treated group were lower than those in the OGD model group, and the expression levels were also lower in the NOB combined with PA-treated group than in the PA group. Hence, our experiments demonstrated that NOB could reduce apoptosis and improve the migration ability and viability of OGD PC12 cells by inhibiting the Rho/ROCK signaling pathway.
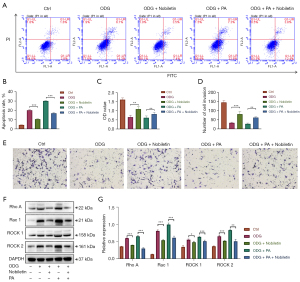
Discussion
Ischemic stroke obstructs the blood supply to local brain tissue, leading to an ischemic and hypoxic lesion and necrosis of brain tissue. Restoring blood flow with thrombolytic therapy within an effective time window reduces the extent of brain tissue damage, but when ischemia is prolonged, restoring blood flow can cause cerebral I/R injury (26). Tissue plasminogen activator (t-PA) is the only FDA-approved drug for thrombolytic therapy in ischemic stroke, but its narrow time window, contraindication restrictions, risk of complications, and low revascularization rates result in a low overall effective treatment rate (27,28). Study have shown that neuroprotective agents can significantly improve the treatment success rate of ischemic stroke, by prolonging the time window of thrombolytic therapy, protecting the blood-brain barrier, promoting revascularization, reducing free radicals, and preventing oxidation and inflammation (7). Neuroprotective agents are essential to improve the treatment success rate of ischemic stroke by intervening in a series of pathophysiological processes to reduce I/R injury (29). The present study’s histopathological results showed that NOB could reduce the cerebral infarction area, and the mNSS and MWM test results confirmed that NOB improved the neurological deficit and learning and memory ability in cerebral I/R injury rats. These findings are consistent with previous research (30).
After cerebral I/R, infiltration of inflammatory cells and release of inflammatory factors cause cerebral reperfusion injury (31). The release of inflammatory factors exacerbates neuron apoptosis, which leads to neurological dysfunction, causing clinical symptoms such as hemiplegia, hemianopia, aphasia, sensory and consciousness impairment in patients with cerebral ischemia. IL-6, IL-1β and TNF-α are key inflammatory factors involved in cerebral ischemia and cerebral reperfusion injury. Study have shown that TNF-α can damage vascular endothelial cells after cerebral ischemia, alter their permeability, increase the adhesion of leukocytes to vascular endothelial cells through various ways and interact with endothelial cells, leading to vascular dysfunction and inducing the coagulation process (32). IL-6 can promote the release of TNF-α to further aggravate the inflammatory response of tissue, leading to stenosis of local blood vessels. At the same time, IL-1β can enhance the adhesion between leukocytes and vascular endothelial cells and the process of leukocytes penetrating the vascular wall, thus stimulating the inflammatory response (33,34). Therefore, inhibiting the release of IL-6, IL-1β and TNF-α can effectively reduce neuron apoptosis, improve neurological deficits, reduce the disability rate and improve the quality of life of patients with cerebral ischemia. The results of the present study showed that NOB reduced the levels of IL-6, IL-1β, and TNF-α in the serum of cerebral I/R injured rats, and the results of both histopathological and Nissl staining confirmed that NOB reduced neuron apoptosis in ischemic brain tissue. Likewise, several studies have indicated that NOB reduces the expressions of IL-6, IL-1β, and TNF-α in animal models of acute liver and kidney injury, aging, and osteoarthritis (35-37), and also decreases apoptosis in ischemic cardiomyocytes, hepatocytes, and neuron (14,38,39). All of this evidence demonstrates the great potential of NOB to be developed as a neuroprotective agent.
The Rho/ROCK signaling pathway mainly consists of Rho GTPase, Rho-associated kinase and their downstream acting substrates (40). Rho GTPases belong to the Ras superfamily, of which Rho (A, B, C) and Rac (1, 2, 3) are the prominent members. The physiological roles of Rho are to promote stress fiber formation and elongation, actin bundle contraction and directed adhesion, while that of Rac is to induce lamellar pseudopod and filopod formation as well as to promote protrusive activity, with Rho A and Rac 1 being the most critical molecules (41-43). ROCK, a serine/threonine protein kinase, is the most functionally well-studied downstream target effector molecule of Rho, with various biological functions, including regulating cell proliferation, migration, contraction, and adhesion (44). There are two subtypes of ROCK, ROCK 1 and ROCK 2, which have biological functions such as cytophagy and contraction (45). During the resting state, the Rho protein structural domain of ROCK interacts with the PH structural domain and the catalytic structural domain of kinase, inhibiting its activity (46). Rho A is activated when stimulated by various factors and mechanical actions. The interaction between Rho A and the structural domain of Rho proteins changes the biological conformation of ROCK, thus relieving the inhibitory effect of the PH structural domain and the catalytic domain of kinase, and activating ROCK. ROCK then undergoes directional translocation close to the myosin light chain (MLC), which acidifies and undergoes myofilament contraction, and also inactivates MLC phosphatase (MLCP) by phosphorylating, preventing the dephosphorylation and inactivation of phosphorylated MLC and promoting myofilament contraction, thereby affecting actomyosin polymerization and causing growth cone atrophy and axon retraction (47). In addition, Rho-kinase downregulates endothelial nitric oxide synthase (eNOS) activity and increases the contractile tension of endothelial cells and vascular smooth muscle cells. Smooth muscle cell tension depends on the dynamic balance of interconversion between intracytoplasmic phosphorylated and dephosphorylated MLC, while the level of MLC phosphorylation is regulated by both Ca2+/calmodulin (CaM)-dependent MLC kinase and Ca2+-independent MLCP (48). In contrast, the Rho/ROCK signaling pathway is a non-Ca2+-dependent signaling pathway. Therefore, phosphorylation directly activates MLC and inhibits MLCP activity to increase the phosphorylation level of MLC and enhance vasoconstriction, leading to cerebral ischemia (49), so the Rho/ROCK signaling pathway is closely linked to the progress of ischemic reperfusion.
The Rho/ROCK signaling pathway inhibitor, fasudil, attenuates chemical-induced nephrotoxicity, inhibits inflammatory factors, apoptotic factors, fibrogenic factors, schistin-activated proteases, and nitrogen oxidation pathways, and improves nephrotic conditions (50). The novel, specific ROCK2 inhibitor, FSD-C10, induces axon growth, BV-2 microglial cell network formation, and promotes the production of brain-derived and glial cell lineage neurotrophic factors (51). DL0805-2 blocks Ang II-induced the increase in MLC phosphorylation and inhibition of p-MLC dephosphorylation, which promotes the diastole of isolated arterioles (52). In addition, Rho-kinase inhibitors can inhibit ROCK activation to suppress inflammatory responses, promote neural regeneration and repair, and improve brain tissue damage after ischemia (43,53). The results of the present study showed that the mRNA and protein expression levels of Rho A, Rac 1, ROCK 1, ROCK 2 were significantly higher in the MCAO group than in the Sham group, suggesting that the Rho/ROCK signaling pathway was involved in the cerebral I/R injury. After being treated with NOB, the mRNA and protein expression levels of Rho A, Rac 1, ROCK 1, and ROCK 2 were significantly reduced,
Similarly, consistent results were observed in the OGD PC12 cell study, indicating that NOB inhibited the Rho/ROCK signaling activity pathway in rats with cerebral I/R injury.
Conclusions
In summary, the ability of NOB to reduce the cerebral infarct area, improve neurological deficits, enhance learning and memory ability, and reduce neuron apoptosis and level of inflammatory factors (IL-6, IL-1, TNF) were confirmed in this study. We also found that the Rho/ROCK signaling pathway was involved in the cerebral I/R injury process in both in vitro and in vivo studies, and that NOB exerted its neuroprotective effects by inhibiting the Rho/ROCK pathway. In conclusion, NOB is believed to be a promising neuroprotective agent. Nevertheless, there are some limitations to our study, such as the regulatory relationship between the reduction in neuron apoptosis by NOB through the Rho/ROCK pathway and the apoptotic pathway, NOB’s relationship with the apoptotic protein Bcl-2 and the apoptotic cascade reaction of caspase, the manner of the Rho/ROCK pathway involvement in cerebral ischemic perfusion injury, how to promote NOB in preclinical studies, and the safety and efficacy of non-human primate studies.
Acknowledgments
We thank the Hainan Province Clinical Medical Center for providing support of experimental research platform.
Funding: This work was supported by grants from the Hainan Province Key R&D Program (No. ZDYF2020165) and National Natural Science Foundation of China (No. 82060851).
Footnote
Reporting Checklist: The authors have completed the ARRIVE reporting checklist. Available at https://atm.amegroups.com/article/view/10.21037/atm-22-6119/rc
Data Sharing Statement: Available at https://atm.amegroups.com/article/view/10.21037/atm-22-6119/dss
Conflicts of Interest: All authors have completed the ICMJE uniform disclosure form (available at https://atm.amegroups.com/article/view/10.21037/atm-22-6119/coif). The authors have no conflicts of interest to declare.
Ethical Statement: The authors are accountable for all aspects of the work in ensuring that questions related to the accuracy or integrity of any part of the work are appropriately investigated and resolved. Animal experiments were performed under a project license (No. Med-Eth-Re[2022]734) granted by the Ethics Committee of Hainan General Hospital, in compliance with institutional guidelines for the care and use of animals.
Open Access Statement: This is an Open Access article distributed in accordance with the Creative Commons Attribution-NonCommercial-NoDerivs 4.0 International License (CC BY-NC-ND 4.0), which permits the non-commercial replication and distribution of the article with the strict proviso that no changes or edits are made and the original work is properly cited (including links to both the formal publication through the relevant DOI and the license). See: https://creativecommons.org/licenses/by-nc-nd/4.0/.
References
- Ahsan A, Liu M, Zheng Y, et al. Natural compounds modulate the autophagy with potential implication of stroke. Acta Pharm Sin B 2021;11:1708-20. [Crossref] [PubMed]
- Ma Q, Li R, Wang L, et al. Temporal trend and attributable risk factors of stroke burden in China, 1990-2019: an analysis for the Global Burden of Disease Study 2019. Lancet Public Health 2021;6:e897-906. [Crossref] [PubMed]
- GBD 2016 Stroke Collaborators. Global, regional, and national burden of stroke, 1990-2016: a systematic analysis for the Global Burden of Disease Study 2016. Lancet Neurol 2019;18:439-58. [Crossref] [PubMed]
- Ye Y, Zhu YT, Xin XY, et al. Efficacy of Chinese herbal medicine for tPA thrombolysis in experimental stroke: A systematic review and meta-analysis. Phytomedicine 2022;100:154072. [Crossref] [PubMed]
- Shah SR. Interventional closure vs. medical therapy of patent foramen ovale for secondary prevention of stroke: updated meta-analysis. Clin Res Cardiol 2019;108:452. [Crossref] [PubMed]
- Knecht T, Story J, Liu J, et al. Adjunctive Therapy Approaches for Ischemic Stroke: Innovations to Expand Time Window of Treatment. Int J Mol Sci 2017;18:2756. [Crossref] [PubMed]
- Luo Y, Tang H, Li H, et al. Recent advances in the development of neuroprotective agents and therapeutic targets in the treatment of cerebral ischemia. Eur J Med Chem 2019;162:132-46. [Crossref] [PubMed]
- Savitz SI, Baron JC, Yenari MA, et al. Reconsidering Neuroprotection in the Reperfusion Era. Stroke 2017;48:3413-9. [Crossref] [PubMed]
- Thirugnanachandran T, Ma H, Singhal S, et al. Refining the is-chemic penumbra with topography. Int J Stroke 2018;13:277-84. [Crossref] [PubMed]
- Paul S, Candelario-Jalil E. Emerging neuroprotective strategies for the treatment of ischemic stroke: An overview of clinical and preclinical studies. Exp Neurol 2021;335:113518. [Crossref] [PubMed]
- Kubo T, Hata K, Yamaguchi A, et al. Rho-ROCK inhibitors as emerging strategies to promote nerve regeneration. Curr Pharm Des 2007;13:2493-9. [Crossref] [PubMed]
- Shin HK, Salomone S, Ayata C. Targeting cerebrovascular Rho-kinase in stroke. Expert Opin Ther Targets 2008;12:1547-64. [Crossref] [PubMed]
- Wu Y, Cheng CS, Li Q, et al. The Application of Citrus folium in Breast Cancer and the Mechanism of Its Main Component Nobiletin: A Systematic Review. Evid Based Complement Alternat Med 2021;2021:2847466. [Crossref] [PubMed]
- Li W, Zhao R, Wang X, et al. Nobiletin-Ameliorated Lipopolysaccharide-Induced Inflammation in Acute Lung Injury by Suppression of NF-κB Pathway In Vivo and Vitro. Inflammation 2018;41:996-1007. [Crossref] [PubMed]
- Cajas YN, Cañón-Beltrán K, Ladrón de Guevara M, et al. Antioxidant Nobiletin Enhances Oocyte Maturation and Subsequent Embryo Development and Quality. Int J Mol Sci 2020;21:5340. [Crossref] [PubMed]
- Ashrafizadeh M, Zarrabi A, Saberifar S, et al. Nobiletin in Cancer Therapy: How This Plant Derived-Natural Compound Targets Various Oncogene and Onco-Suppressor Pathways. Biomedicines 2020;8:110. [Crossref] [PubMed]
- Nakajima A, Ohizumi Y. Potential Benefits of Nobiletin, A Citrus Flavonoid, against Alzheimer's Disease and Parkinson's Disease. Int J Mol Sci 2019;20:3380. [Crossref] [PubMed]
- Braidy N, Behzad S, Habtemariam S, et al. Neuroprotective Effects of Citrus Fruit-Derived Flavonoids, Nobiletin and Tangeretin in Alzheimer's and Parkinson's Disease. CNS Neurol Disord Drug Targets 2017;16:387-97. [Crossref] [PubMed]
- Amarsanaa K, Kim HJ, Ko EA, et al. Nobiletin Exhibits Neuroprotective Effects against Mitochondrial Complex I Inhibition via Regulating Apoptotic Signaling. Exp Neurobiol 2021;30:73-86. [Crossref] [PubMed]
- Zhang L, Zhao H, Zhang X, et al. Nobiletin protects against cerebral ischemia via activating the p-Akt, p-CREB, BDNF and Bcl-2 pathway and ameliorating BBB permeability in rat. Brain Res Bull 2013;96:45-53. [Crossref] [PubMed]
- Huang D, Zhou J, Li W, et al. Casticin protected against neuronal injury and inhibited the TLR4/NF-κB pathway after middle cerebral artery occlusion in rats. Pharmacol Res Perspect 2021;9:e00752. [Crossref] [PubMed]
- Shen JD, Ma LG, Hu CY, et al. Berberine up-regulates the BDNF expression in hippocampus and attenuates corticosterone-induced depressive-like behavior in mice. Neurosci Lett 2016;614:77-82. [Crossref] [PubMed]
- Tuo QZ, Zhang ST, Lei P. Mechanisms of neuronal cell death in ischemic stroke and their therapeutic implications. Med Res Rev 2022;42:259-305. [Crossref] [PubMed]
- Zhang W, Zhao W, Li Q, et al. 3D-printing magnesium-polycaprolactone loaded with melatonin inhibits the development of osteosarcoma by regulating cell-in-cell structures. J Nanobiotechnology 2021;19:263. [Crossref] [PubMed]
- Mizuno N, Abe K, Morishita Y, et al. Pentanoic acid induces thymic stromal lymphopoietin production through Gq/11 and Rho-associated protein kinase signaling pathway in keratinocytes. Int Immunopharmacol 2017;50:216-23. [Crossref] [PubMed]
- Khoshnam SE, Winlow W, Farzaneh M, et al. Pathogenic mechanisms following ischemic stroke. Neurol Sci 2017;38:1167-86. [Crossref] [PubMed]
- Hacke W, Kaste M, Bluhmki E, et al. Thrombolysis with alteplase 3 to 4.5 hours after acute ischemic stroke. N Engl J Med 2008;359:1317-29. [Crossref] [PubMed]
- Chamorro Á, Dirnagl U, Urra X, et al. Neuroprotection in acute stroke: targeting excitotoxicity, oxidative and nitrosative stress, and inflammation. Lancet Neurol 2016;15:869-81. [Crossref] [PubMed]
- Rahman AA, Amruta N, Pinteaux E, et al. Neurogenesis After Stroke: A Therapeutic Perspective. Transl Stroke Res 2021;12:1-14. [Crossref] [PubMed]
- Yasuda N, Ishii T, Oyama D, et al. Neuroprotective effect of nobiletin on cerebral ischemia-reperfusion injury in transient middle cerebral artery-occluded rats. Brain Res 2014;1559:46-54. [Crossref] [PubMed]
- Przykaza Ł. Understanding the Connection Between Common Stroke Comorbidities, Their Associated Inflammation, and the Course of the Cerebral Ischemia/Reperfusion Cascade. Front Immunol 2021;12:782569. [Crossref] [PubMed]
- Maddahi A, Kruse LS, Chen QW, et al. The role of tumor necrosis factor-α and TNF-α receptors in cerebral arteries following cerebral ischemia in rat. J Neuroinflammation 2011;8:107. [Crossref] [PubMed]
- Tułowiecka N, Kotlęga D, Bohatyrewicz A, et al. Could Lipoxins Represent a New Standard in Ischemic Stroke Treatment? Int J Mol Sci 2021;22:4207. [Crossref] [PubMed]
- Saito K, Suyama K, Nishida K, et al. Early increases in TNF-alpha, IL-6 and IL-1 beta levels following transient cerebral ischemia in gerbil brain. Neurosci Lett 1996;206:149-52. [Crossref] [PubMed]
- Güvenç M, Cellat M, Gökçek İ, et al. Nobiletin attenuates acetaminophen-induced hepatorenal toxicity in rats. J Biochem Mol Toxicol 2020;34:e22427. [Crossref] [PubMed]
- Bi J, Zhang H, Lu J, et al. Nobiletin ameliorates isoflurane-induced cognitive impairment via antioxidant, anti-inflammatory and anti-apoptotic effects in aging rats. Mol Med Rep 2016;14:5408-14. [Crossref] [PubMed]
- Yang G, Li S, Yuan L, et al. Effect of nobiletin on the MAPK/NF-κB signaling pathway in the synovial membrane of rats with arthritis induced by collagen. Food Funct 2017;8:4668-74. [Crossref] [PubMed]
- Liu F, Zhang H, Li Y, et al. Nobiletin suppresses oxidative stress and apoptosis in H9c2 cardiomyocytes following hypoxia/reoxygenation injury. Eur J Pharmacol 2019;854:48-53. [Crossref] [PubMed]
- Wang T, Wang F, Yu L, et al. Nobiletin alleviates cerebral ischemic-reperfusion injury via MAPK signaling pathway. Am J Transl Res 2019;11:5967-77.
- Etienne-Manneville S, Hall A. Rho GTPases in cell biology. Nature 2002;420:629-35. [Crossref] [PubMed]
- Zhou Q, Gensch C, Liao JK. Rho-associated coiled-coil-forming kinases (ROCKs): potential targets for the treatment of atherosclerosis and vascular disease. Trends Pharmacol Sci 2011;32:167-73. [Crossref] [PubMed]
- Hall A, Lalli G. Rho and Ras GTPases in axon growth, guidance, and branching. Cold Spring Harb Perspect Biol 2010;2:a001818. [Crossref] [PubMed]
- Kimura T, Horikoshi Y, Kuriyagawa C, et al. Rho/ROCK Pathway and Noncoding RNAs: Implications in Ischemic Stroke and Spinal Cord Injury. Int J Mol Sci 2021;22:11573. [Crossref] [PubMed]
- Murakoshi H, Wang H, Yasuda R. Local, persistent activation of Rho GTPases during plasticity of single dendritic spines. Nature 2011;472:100-4. [Crossref] [PubMed]
- Syed ZA, Rapuano CJ. Rho kinase (ROCK) inhibitors in the management of corneal endothelial disease. Curr Opin Ophthalmol 2021;32:268-74. [Crossref] [PubMed]
- Yamamoto T, Ugawa Y, Kawamura M, et al. Modulation of microenvironment for controlling the fate of periodontal ligament cells: the role of Rho/ROCK signaling and cytoskeletal dynamics. J Cell Commun Signal 2018;12:369-78. [Crossref] [PubMed]
- Arita R, Hata Y, Ishibashi T. ROCK as a Therapeutic Target of Diabetic Retinopathy. J Ophthalmol 2010;2010:175163. [Crossref] [PubMed]
- Shi J, Wei L. Rho Kinases in Embryonic Development and Stem Cell Research. Arch Immunol Ther Exp (Warsz) 2022;70:4. [Crossref] [PubMed]
- Fernández DI, Kuijpers MJE, Heemskerk JWM. Platelet calcium signaling by G-protein coupled and ITAM-linked receptors regulating anoctamin-6 and procoagulant activity. Platelets 2021;32:863-71. [Crossref] [PubMed]
- Mateos-Olivares M, García-Onrubia L, Valentín-Bravo FJ, et al. Rho-Kinase Inhibitors for the Treatment of Refractory Diabetic Macular Oedema. Cells 2021;10:1683. [Crossref] [PubMed]
- Xin YL, Yu JZ, Yang XW, et al. FSD-C10: A more promising novel ROCK inhibitor than Fasudil for treatment of CNS autoimmunity. Biosci Rep 2015;35:00247. [Crossref] [PubMed]
- Yuan TY, Yan Y, Wu YJ, et al. Vasodilatory effect of a novel Rho-kinase inhibitor, DL0805-2, on the rat mesenteric artery and its potential mechanisms. Cardiovasc Drugs Ther 2014;28:415-24. [Crossref] [PubMed]
- Kubo T, Yamaguchi A, Iwata N, et al. The therapeutic effects of Rho-ROCK inhibitors on CNS disorders. Ther Clin Risk Manag 2008;4:605-15. [Crossref] [PubMed]
(English Language Editor: K. Brown)