Prospective and therapeutic screening value of non-coding RNA as biomarkers in cardiovascular disease
Introduction
According to data from the World Health Organization, an estimated 17.5 million people die annually from cardiovascular diseases (CVD), representing 31% of all global deaths. The vast majority dies from stroke and coronary artery disease (CAD).
Atherosclerosis impairs vascular functional integrity, and causes serious morbidity long before these “combined endpoints”. One quarter of the Western population suffers from some form of CAD or the consequences of stroke (1,2). This is compounded by aneurysm, peripheral arterial occlusive disease (PAOD), vascular dementia, pulmonary arterial hypertension (PAH), and venous disease. Treatment strategies are focused on prevention by controlling risk factors and early states in the epidemiologic—and sepcific diagnosis and treatment in the individualized setting, as well as specific diagnosis and treatment in the individualized setting.
For the latter, disease biomarkers are of utmost utility and importance, for instance, cardiac troponin T/I (TnT/I) for the diagnosis of myocardial infarction (MI), and NT-proBNP (BNP) for the diagnosis and monitoring of heart failure due to left ventricular remodeling (LVM) (3). A biomarker is a measurable and quantifiable biological parameter, and might as such be used for screening, identifying, categorizing, monitoring or predicting disease, risk and therapy (4). Classic protein biomarkers, however, are only available and clinically useful for a limited number of diseases. Their utility is compromised by inter- and intra-individual heterogeneity of diseases, specific genetics and proteomics, as well as the influence of lifestyle (5). In contrast, due to their generalized role in pathologic conditions, non-coding RNAs (ncRNAs) have great potential for future biomarker approaches.
Non-coding RNA (ncRNA)
Over the last few years, it has been established that only 3% of the human genome codes for protein genes. Approximately 80% of the genome is, however, transcribed regularly (6). RNA and genomic deep sequencing have revealed that the ever growing number of non-coding transcripts by far exceeds protein-coding mRNA. This is believed to shape complexity in species, since the proportion of ncRNA increases with higher rank in evolutionary development (7,8).
The first microRNA (miRNA) was described in 1993, and since then, approximately 1,800 of these 16–22 nucleotide (nt) long transcripts have been annotated, with great uncertainty about the final number (9,10). Some of them have been well investigated in various diseases, and the first candidates have now entered clinical trials, both for diagnostic and therapeutic applications (11). They constitute the vast majority of small ncRNA (<200 nt), whereas long ncRNA (lncRNA) includes transcripts >200 nt. This number is believed to be around 9,000 (6). miRNAs act mainly at the post-transcriptional level by regulating mRNA decay or inhibition.
LncRNAs multiply the challenge to the central dogma of nuclear DNA-transcription and subsequent cytoplasmic mRNA-translation via multiple mechanisms. These include histone modification, transcript regulation, alternate splicing, mRNA fragmentation, endo-sponge activity and direct protein interaction (Figure 1). miRNAs are most often located in the promoter regions of distinct genes, either singly or in clusters. One molecule can have hundreds of (often functionally related) mRNA targets, thus miRNAs constitute dense regulatory networks for approximately two thirds of all genes (12). LncRNAs have a more heterogeneous distribution in the genome, with nested and overlapping, sense and antisense transcripts (13). Although their structure is not as evolutionary conserved as that for miRNAs, their function within the regulatory network is (14,15).
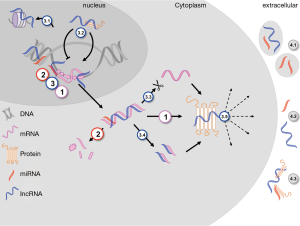
Additionally, there is growing evidence suggesting numerous interactions between the different classes of RNAs due to complementary binding sites. miRNAs might thus directly control action and transcription of lncRNAs, whereas these in return might smother miRNA effects, i.e., by endo-sponge-activity (16,17). These closely interwoven relationships emphasize the likely involvement of a network, rather than a single gene, when investigating ncRNA changes in disease (18). A genome-wide shift of corresponding miRNA-mRNA expressions in CVD patients was demonstrated in the Framingham population, and a fast dynamically regulated transcriptome of the myocardium, after mechanical support in a small patient cohort, showed significant changes in lncRNA expression (19-21). Whereas the mechanistic role of ncRNA in CVD has been reviewed extensively before, their potential as novel biomarkers in CVDs appears as an intriguing topic, especially since different expression levels might represent different stages of a disease.
Circulating non-coding RNA (ncRNA)
For use as classic biomarkers, ncRNAs must be easily accessible by routine diagnostic methods, suggesting extracellular circulating candidates as valuable targets. Their abundance in plasma, urine, saliva and cerebrospinal fluid (CSF) suggests a specific role in inter-cellular signaling, rather than just an intracellular function. Their high stability in body fluids compared to mRNA or genomic DNA is due to smaller size, and compartmentalization into exosomes, microparticles, apoptotic bodies, lipoprotein and protein complexes [Figure 1; reviewed in detail by (18)].
Blood is the most promising compartment for biomarker investigations in the context of CVD, due to its close relationship with the affected tissues, easy accessibility and the possibility of testing multiple targets with only one probe. There have been confounding reports with inconsistent results for various miRNAs regarding CVD for other body fluids; urine analysis might have a role for investigating kidney disease (22-24). Most studies report analysis from “serum”, “plasma” or “whole blood”, with, unfortunately, heterogeneous definitions of these terms. Only very few studies address the cellular and acellular fractions, and their respective role, in ncRNA transport in circulation (Table S1) (25).
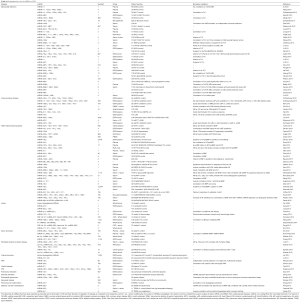
Full table
Nevertheless, the use of circulating ncRNA as biomarkers is persuasive, especially in the context of signature and network analysis for disease detection and progression. Therefore, our aim here is to summarize and evaluate the existing evidence for the analytic and predictive potential of different ncRNAs in CVD.
microRNA (miRNA) in cardiovascular diseases (CVDs)
A large number of miRNAs has been studied in the context of acute MI and its concluding remodeling processes within the heart. Nevertheless, a variety of other CVDs, both on the arterial and the venous side, have followed.
We therefore performed a complete literature review on the terms “miRNA”, “ncRNA” and “lncRNA” in combination with “biomarker” and the respective CVDs discussed below. The results from over 100 studies are shown in detail in the supplementary material (Table S1). From these, miRNAs reported more than once in two independent studies, and all lncRNAs are reviewed in detail in this manuscript (Tables 1,2).
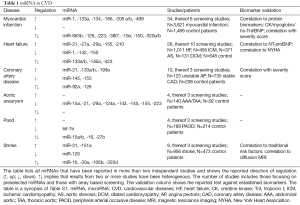
Full table
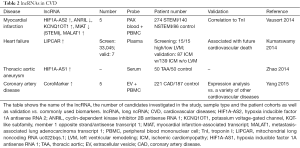
Full table
Myocardial infarction (MI)
Since 2010, more than 30 studies have assessed the use of miRNAs as biomarkers for acute MI diagnosis, and their predictive values for post-MI outcomes, in a combined total of over 3,500 patients. Unfortunately, most of them were identified using a retrospective approach, with however, fairly large and often well diagnosed cohorts. Protein biomarkers, based on cellular decay, such as TnI/T and creatine kinase MB (CK-MB), have been very well established in clinical use, so the evaluation versus this current standard is of utmost importance.
Most studies were focused on the group miRNA-1, -133a/b, -208a, -499. These so-called myomirs are heart specific, due to their regulatory interaction with the transcripts of different cardiac muscle myosin chains, similar to CK-MB (Table 1) (26). Changes in the circulatory levels of these miRNAs specifically represent heart tissue. A small number of screening studies did not lead to further validated MI specific candidates, and only miRNA-134 and miRNA-186 were detected in more than two separate studies (27-29). While there appears to be no heart specific context for miRNA-186, miRNA-134 has been suggested as a promoter of cardiac progenitor cells in vitro (30). All studies report higher abundance of those candidates in blood of MI patients, however receiver-operator curve (ROC) analysis of the predictive power of miRNA changes did not outperform classic TnI/T or CK-MB in most studies, and was shown to be inferior in the biggest cohort with 1,155 prospective patients with acute onset of chest pain (Table S1) (31). In addition, this cohort attributed a great predictive power for MI lifetime risk to circulating miRs-126, -223 and -197 after ten years follow-up (32).
Release kinetics have been closely studied by Liebetrau et al. in an experimental setting, revealing significant elevation after 15 minutes and a peak at 85 minutes for miRNA-1 and -133, in close correlation with TnI/T, but without additional benefit. Other studies showed earlier peaks for miRNA-133a/b, -208 and -499 (33-36). Similar results were also demonstrated for heart specific enzymes and miRNAs in marathon runners, before and after aerobic exercise (37). miRNA-499 levels may, however, be predictive of one-year mortality after MI, whereas miRNA-208a levels may be predictive of 30-day mortality (31,38). Additionally, miRNA-133a, -499 levels were shown to correlate with CAD severity, based on percentage and number of occluded coronary vessels (Gensini score) (39,40).
In summary, these results demonstrate very well the utility of circulating miRNAs as specific biomarkers for mechanistically well-defined candidates. The myomirs, however, are currently not superior to protein biomarkers for diagnosing MI. Their predictive power for specific “after-effects” requires further evaluation and research.
Coronary artery disease (CAD)
For discriminating unstable angina pectoris (AP) and stable CAD, higher levels of miRNA-21, -133a/b, -199a, and lower levels of -145, -155 were suggested (Table 1). Until now, only a small number of 125 patients with unstable AP, combined in ten studies, have been analyzed, requiring confirmation in larger cohorts for more reliable findings when facing clinical decision making.
Most studies failed to show discriminatory power for either a single or a subset of circulating miRNAs (Table S1). However, lower levels of miRNA-145 and -155 showed an inverse correlation with CAD severity scores (Gensini and SYNTAX), and thus can add to the above-mentioned miRNA-133a, -499 correlations with vessel calcification (41,42). Interestingly, miRNA-155 was shown to be down-regulated in the plasma and tissue of AAA patients, and up-regulated in the plasma and tissue of heart failure patients (43-45). In vitro studies suggest a cell-specific pro-atherogenic effect under adaptive angiogenesis (46). Proliferative effects of miRNA-21, -199a, and anti-proliferative effects of miRNA-145, add to the possible concerted hyperplastic ability of these candidates (47).
All current studies are unable to provide sufficient statistical power to detect unstable AP in a clinically useful setting. The suggested candidate miRNAs have, however, a high potential to achieve this in larger trials, based on their experimentally proven role in atherosclerosis.
Heart failure and remodeling
Heart failure has been extensively studied for the involvement of miRNAs, and the first pre-clinical trials on therapeutic applications come from this field. Their use as biomarkers was addressed in over 25 studies. However, these often lacked sufficient power due to the inclusion of heterogeneous clinical phenotypes, such as ischemic, obstructive and dilative cardiomyopathy in relation to aortic stenosis (AS), congenital or post-MI origin (Table 1).
High circulating levels of miRNA-21, -27a, -29a, -155, -210, and low levels of miRNA-1, -142, -150, singly or in different combinations, could be demonstrated to correlate with the established protein biomarker for HF and BNP (Table S1). While miRNA-1, -21, -29a, -155, -210 have more or less defined roles from coherent in vitro and tissue studies, little is known about the other candidates (48,49).
Identification of HF in dyspneic patients could not be achieved by circulating miRNAs. Some candidate levels did however correlate well with disease severity indicated by NYHA stage (50-52). A lower expression of miRNA-150 was consistently associated with worse left ventricular function (53,54). Confounding evidence exists for the cardiac miRNA-133a, which was found at higher or lower expression levels in two independent cohorts of 246 and 64 patients. In both studies, its expression levels were associated with a worse outcome for LVM (55,56). In addition, subgroup analysis for AS indicated higher circulating levels for pro-fibrotic miRNA-21 (57,58).
In summary, the very well-studied influence of miRNAs on cardiac remodeling is currently poorly reflected by their applicability as biomarkers for disease, or severity predictors in heart failure.
Stroke
Although the most frequent outcome of CVD, only few studies have investigated miRNA biomarkers in stroke (Table S1). Among them, no prospective cohorts were studied, and only patients with the diagnosis of stroke by traditional means were included.
Leung et al. suggested a signature to discriminate between hemorrhagic and ischemic stroke, which was not, however, reproducible by others (59). Comparative analysis of blood and CSF has been confounding, suggesting a distinct role for the blood brain barrier in circulating miRNA shuttling (24,60). Coherent reports revealed up-regulation of miRNA-21 and -151a in patients with ischemic stroke (Table 1) (24,61,62). miRNA-21 elevation has also been shown in patients with carotid artery disease, and therefore been attributed with a predictive power for cerebrovascular events (63). The combination with miRNA-151a might be of interest, since it is encoded in the PTK2 gene, which is eventually triggered in response to neuronal damage (64).
Currently, no correlation for circulating miRNA in the detection or outcome after stroke exists.
Aneurysm disease
Aneurysm disease is mostly investigated in the setting of thoracic aortic, abdominal aortic (TAA/AAA), or intracranial aneurysms (ICA), via array-based candidate screening in small patient cohorts.
No coherent results from two studies of ICA were reported (Table S1). A subset of miRNA-15a, -21, -29a, -124a, -143, -145, -155, -223, however, showed down-regulation in AAA serum (Table 1) (45,65). Among them, miRNA-21 and -145 had a lower expression in AAA and TAA, despite the different embryologic background of these distinct parts of the aorta (66). Parallel tissue analysis revealed that miRNA-29, -124a, -155, and -223 were also repressed at the cellular level, whereas miRNA-21 expression was enhanced when compared to non-aneurysmatic controls (67). Additionally, a distinct role has been attributed to the miRNA-29 in AAA development (68). Apart from miR-21, detailed mechanistic studies are currently missing (67).
Future biomarkers on this subject should address a correlation with aneurysm size and rupture rate as eventual predictors of expansion and fatal outcome, as well as indicating patients prone to aneurysm development not only at aortic locations. This is of special interest, since there is currently no biomarker available for this clinically most-relevant purpose.
Peripheral arterial disease
Four independent studies on PAOD and critical limb ischemia (CLI) have not been able to identify an miRNA signature specific for the distinct clinical problems associated with this disease. These include stenosis-rate, localization, re-stenosis and ischemia. Only let-7e was reported to be lower in expression in the serum of PAOD patients in two studies (Table 1) (69,70). Despite its eventual role as a regulator of angiogenesis at the level of endothelial cells, little is known about its involvement in CVD (71).
Venous disease
Venous disease has been very sparsely addressed in two studies profiling patients after venous thromboembolism, and after pulmonary embolism (Table S1) (72,73). Unfortunately no matching candidates were found. Zhang et al., however, identified an up-regulation of miRNA-210, a known promoter of cell survival, in the plasma of eight patients with radiographic cerebral AV-malformation, thereby providing a link between the venous to the arterial side, in which miRNA-210 is involved in various conditions (Table S1) (74).
Diabetic vascular manifestations
In the context of diabetes, miRNAs are most often studied for disease identification (75). Despite the manifold heterogeneous aspects and complications of the disease, a few studies have focused on vascular manifestations other than diabetic retinopathy.
Peng et al. identified a correlation between urine miRNA-29a and albuminuria in a total of 83 type II diabetic patients. Pro-fibrotic miRNA-29b correlated inversely with carotid intima-media thickness, further suggesting a kidney-independent clearance of these miRNAs (68,76). Neointimal hyperplasia after coronary artery stenting, and its response to therapy after oral pioglitazone, an insulin sensitizer, was found to correlate with serum levels of miRNA-24 in 72 diabetic patients (77). This short ncRNA is a regulator of cytokine synthesis in macrophages, and migration in aortic smooth muscle cells (78). Finally Caporali et al. showed in 11 diabetic CLI patients, a concordant elevation in the serum and tissue levels of miRNA-503, a transcript with anti-proliferative effects, but with no known functional role in the field of CVD (79).
Other CVD
Besides these more or less frequent CVDs, a few pilot studies have been performed on more rare diseases, or special settings of pathologies (Table S1). In a study of 37 children with Kawasaki’s disease, among them 50% with coronary artery aneurysm, no significantly altered miRNA could be identified (80). Dong et al. reported a specific signature of three miRNAs that distinguished vascular dementia from Alzheimer’s disease (81). Two studies investigated serum from a total of 187 PAH patients, and suggested low miRNA-150 and high miRNA-23a correlated with survival and cardiac index respectively (82,83). The 28-day survival in critically ill patients with acute kidney injury was reported to be another predictive ability for miRNA-210 (84). Ferreira et al. could validate the cardiac myomirs to be elevated in Chagas disease associated cardiomyopathy (85).
Long non-coding RNA (lncRNA) in CVD
The number of lncRNAs studied in CVD is still very limited. Eight different transcripts have been studied as potential biomarkers in four studies with promising results (Table 2).
Higher levels of LIPCAR in plasma from HF patients following ICM were independently associated with an elevated risk for future cardiovascular death, and predictive for LVM (86). This effect was also reported for ANRIL, KCNQ1OT1, MIAT, and MALAT1 in a cohort of 414 MI patients (87). HIF1a-AS2, KCNQ1OT1, and MALAT1 were higher, ANRIL was lower, in patients with acute MI compared to healthy volunteers, and HIF1a-AS2 levels varied based on time of presentation after onset of chest pain. Additionally, ANRIL, KCNQ1OT1, MIAT, and MALAT1 had good predictive power to distinguish between ST-elevation myocardial infarction (STEMI) and non-STEMI (NSTEMI). CAD could be distinguished with high sensitivity from other CVD in the serum of 221 CAD patients, compared to 187 controls, by higher plasma levels of CoroMarker. This is a novel transcript without any determinable annotation (88). Finally, HIF1a-AS1 serum levels were significantly increased in 50 patients with TAA (89).
Little is known about the specific functions of these transcripts within the heart or vascular tissue. MIAT expression has been linked to genetic susceptibility for MI, and might act as a competing endogenous RNA for various targets (90,91). ANRIL was shown to be highly expressed in atherosclerotic plaques, and might be a “fine-tuner” within the inflammatory NF-κB pathway, by acting as an antisense regulator to the CDKN2B-CDKN2A gene cluster at the 9p21 locus (92,93). KCNQ1OT1 is an antisense transcript to KCNQ1, an epigenetic regulator of many targets, known from its pathogenic role in Beckwith-Wiedemann syndrome (94). HIF1a-AS1 and -AS2 are also antisense transcripts modulating HIF1A, a pro-angiogenic and anti-apoptotic gene up-regulated in many, if not all, CVDs (89).
ncRNA for therapy monitoring
Therapy monitoring via ncRNA in CVD has so far only been reported in pilot studies, but nevertheless has great potential for future investigations.
Three studies have investigated the predictive role of miRNAs for rejection and graft failure after heart transplantation. Duong Van Huyen et al. screened a total of 113 heart transplant patients, and correlated serum and biopsy specimen miRNA levels from 30 subjects suffering from graft rejection. Four candidates were differentially expressed in blood and tissue coherently, and suggested as early markers for graft rejections, with special interest towards miRNA-155 up-regulation, which is also found in the failing heart (Table 1) (95). Wang et al. reported that preselected miRNA-133a/b, -208a levels in a very small set of seven patients were superior to TnI in predicting early graft failure in association with MI (96). Another seven candidates were found to be up-regulated in the same setting by Sukma et al., with no overlap with the previous studies or heart-specific miRNAs (97).
Willeit et al. discovered miRNAs with dose- and substance-specific expression during anti-platelet therapy, suggestive of a potential role for monitoring drugs that affect platelet function (98). Positive thiazolidinedione treatment response in type II diabetics was indicated by a lower expression of miRNA-320a, known to be elevated in hyperglycemic individuals (99,100).
Limitations in circulating ncRNA
All investigations reported so far have weaknesses in study design and applied methods, emphasizing a need for large multicenter trial cohort studies with, ideally, a screening and a validation cohort.
Only very few studies screened for multiple ncRNAs, due to high costs of array based investigations, but rather focused on preselected candidates from previous tissue profiling evaluations (Tables 1,S1). Cellular and extracellular signatures, however, cannot be expected to match completely, due to tissue-, cell-, and compartment-specific expression levels, and differing functions (45,65,95). Several other factors have been shown to influence ncRNA serum levels, some of which have great importance when investigating CVD. In particular, anti-platelet medication, heparin and statin treatment might influence circulating miRNA levels and release kinetics (101,102). In patients with end-stage kidney disease and eventual dialysis, the validity of circulating miRNAs is controversial, and warrants further research (56,103). From a more general perspective, age, sex, and smoking have been identified as confounders of circulating miRNA and microparticle distribution (104-106).
Especially for acute events, the timing and site of blood collection remains important, since the circulating transcriptome changes rapidly, and their levels might be altered during the passage from the arterial to the venous side (33,107,108). Furthermore, eventual heterogeneity of expression among different ethnic groups has to be taken into account (109,110). A major shortcoming in miRNA biomarker research is the difficulty of standardization for endogenous controls, which differs tremendously among all reported studies (111). Whether or not these concerns are also valid for circulating lncRNAs remains to be elucidated.
Therefore, discovery and evaluation of a more generalized biomarker in the complex regulatory network of disease, apart from generic markers of cellular damage and apoptosis (e.g., TnI or transaminase levels) requires elaborate study preparation, execution, and analysis.
Conclusions
In the emerging field of circulating ncRNA as biomarkers in CVD, the most persuasive results have come from plasma studies in MI and HF. In these conditions, tissue-specific signatures of miRNA expression levels in particular have proven equal to protein biomarkers in their great potential for predictive clinical use. The main reason for this is the well-advanced mechanistic understanding of certain ncRNAs, and how they are regulated (and regulating) under physiologic as well as pathologic conditions.
Probably the greatest clinical need for ncRNA biomarkers can be found in disease settings for which currently no alternatives are available. Disease development, outcome prediction and treatment response are such areas, especially when complicated pathologies require stratification of complex and cost-worthy treatment strategies. In addition to the sheer information, whether a patient has an acute MI or not, a disease-specific signature of ncRNAs could provide distinct information about localization and lesion area, the number of obstructed vessels, and early and/or late ischemia-related mortality. For this purpose, future studies require the necessary power, patient characteristics, uniform disease definitions, and ideally, parallel tissue expression detection for mechanistic purposes. If all these criteria are thoughtfully taken into account, the ncRNAs certainly have the ability to optimize biomarker applications in a time of evolving personalized medicine.
Acknowledgements
Funding: Research on non-coding RNAs in cardiovascular disease in Lars Maegdefessel’s lab is supported by the European Research Council (ERC StG NORVAS), the Swedish Heart-Lung-Foundation (20120615, 20130664, 20140186), the Ragnar Söderberg Foundation (M-14/55), and the Swedish Research Council (2015-01340).
Footnote
Conflicts of Interest: The authors have no conflicts of interest to declare.
References
- Mozaffarian D, Benjamin EJ, Go AS, et al. Heart disease and stroke statistics--2015 update: a report from the American Heart Association. Circulation 2015;131:e29-322. [Crossref] [PubMed]
- Leal J, Luengo-Fernandez R, Gray A, et al. Economic burden of cardiovascular diseases in the enlarged European Union. Eur Heart J 2006;27:1610-9. [Crossref] [PubMed]
- Hirayama A, Kusuoka H, Yamamoto H, et al. Serial changes in plasma brain natriuretic peptide concentration at the infarct and non-infarct sites in patients with left ventricular remodelling after myocardial infarction. Heart 2005;91:1573-7. [Crossref] [PubMed]
- Vasan RS. Biomarkers of cardiovascular disease: molecular basis and practical considerations. Circulation 2006;113:2335-62. [Crossref] [PubMed]
- Enroth S, Johansson A, Enroth SB, et al. Strong effects of genetic and lifestyle factors on biomarker variation and use of personalized cutoffs. Nat Commun 2014;5:4684. [Crossref] [PubMed]
- Pennisi E. Genomics. ENCODE project writes eulogy for junk DNA. Science 2012;337:1159-61. [Crossref] [PubMed]
- Liu G, Mattick JS, Taft RJ. A meta-analysis of the genomic and transcriptomic composition of complex life. Cell Cycle 2013.2061-72. [PubMed]
- Arner E, Daub CO, Vitting-Seerup K, et al. Transcribed enhancers lead waves of coordinated transcription in transitioning mammalian cells. Science 2015;347:1010-4. [Crossref] [PubMed]
- Lee RC, Feinbaum RL, Ambros V, et al. The C. elegans heterochronic gene lin-4 encodes small RNAs with antisense complementarity to lin-14. Cell 1993;75:843-54. [Crossref] [PubMed]
- Londin E, Loher P, Telonis AG, et al. Analysis of 13 cell types reveals evidence for the expression of numerous novel primate- and tissue-specific microRNAs. Proc Natl Acad Sci U S A 2015;112:E1106-15. [Crossref] [PubMed]
- Janssen HL, Kauppinen S, Hodges MR. HCV infection and miravirsen. N Engl J Med 2013;369:878. [PubMed]
- Friedman RC, Farh KK, Burge CB, et al. Most mammalian mRNAs are conserved targets of microRNAs. Genome Res 2009;19:92-105. [Crossref] [PubMed]
- Shi Q, Yang X. Circulating MicroRNA and Long Noncoding RNA as Biomarkers of Cardiovascular Diseases. J Cell Physiol 2015. [Epub ahead of print]. [PubMed]
- Derrien T, Johnson R, Bussotti G, et al. The GENCODE v7 catalog of human long noncoding RNAs: analysis of their gene structure, evolution, and expression. Genome Res 2012;22:1775-89. [Crossref] [PubMed]
- Qu Z, Adelson DL. Evolutionary conservation and functional roles of ncRNA. Front Genet 2012;3:205. [Crossref] [PubMed]
- Guil S, Esteller M. RNA-RNA interactions in gene regulation: the coding and noncoding players. Trends Biochem Sci 2015;40:248-56. [Crossref] [PubMed]
- Jalali S, Bhartiya D, Lalwani MK, et al. Systematic transcriptome wide analysis of lncRNA-miRNA interactions. PloS one 2013;8:e53823. [Crossref] [PubMed]
- Zampetaki A, Willeit P, Drozdov I, et al. Profiling of circulating microRNAs: from single biomarkers to re-wired networks. Cardiovasc Res 2012;93:555-62. [Crossref] [PubMed]
- Huan T, Rong J, Tanriverdi K, et al. Dissecting the roles of microRNAs in coronary heart disease via integrative genomic analyses. Arterioscler Thromb Vasc Biol 2015;35:1011-21. [Crossref] [PubMed]
- Yang KC, Yamada KA, Patel AY, et al. Deep RNA sequencing reveals dynamic regulation of myocardial noncoding RNAs in failing human heart and remodeling with mechanical circulatory support. Circulation 2014;129:1009-21. [Crossref] [PubMed]
- Matkovich SJ, Van Booven DJ, Youker KA, et al. Reciprocal regulation of myocardial microRNAs and messenger RNA in human cardiomyopathy and reversal of the microRNA signature by biomechanical support. Circulation 2009;119:1263-71. [Crossref] [PubMed]
- Mlcochova H, Hezova R, Meli AC, et al. Urinary microRNAs as a new class of noninvasive biomarkers in oncology, nephrology, and cardiology. Methods Mol Biol 2015;1218:439-63. [Crossref] [PubMed]
- Papadopoulos T, Belliere J, Bascands JL, et al. miRNAs in urine: a mirror image of kidney disease? Expert Rev Mol Diagn 2015;15:361-74. [Crossref] [PubMed]
- Sørensen SS, Nygaard AB, Nielsen MY, et al. miRNA expression profiles in cerebrospinal fluid and blood of patients with acute ischemic stroke. Transl Stroke Res 2014;5:711-8. [Crossref] [PubMed]
- Mayr M, Zampetaki A, Willeit P, et al. MicroRNAs within the continuum of postgenomics biomarker discovery. Arterioscler Thromb Vasc Biol 2013;33:206-14. [Crossref] [PubMed]
- van Rooij E, Quiat D, Johnson BA, et al. A family of microRNAs encoded by myosin genes governs myosin expression and muscle performance Dev Cell 2009;17:662-73. [Crossref] [PubMed]
- He F, Lv P, Zhao X, et al. Predictive value of circulating miR-328 and miR-134 for acute myocardial infarction. Mol Cell Biochem 2014;394:137-44. [Crossref] [PubMed]
- Li C, Fang Z, Jiang T, et al. Serum microRNAs profile from genome-wide serves as a fingerprint for diagnosis of acute myocardial infarction and angina pectoris. BMC Med Genomics 2013;6:16. [Crossref] [PubMed]
- Zeller T, Keller T, Ojeda F, et al. Assessment of microRNAs in patients with unstable angina pectoris. Eur Heart J 2014;35:2106-14. [Crossref] [PubMed]
- Wu YH, Zhao H, Zhou LP, et al. miR-134 Modulates the Proliferation of Human Cardiomyocyte Progenitor Cells by Targeting Meis2. Int J Mol Sci 2015;16:25199-213. [Crossref] [PubMed]
- Devaux Y, Mueller M, Haaf P, et al. Diagnostic and prognostic value of circulating microRNAs in patients with acute chest pain. J Intern Med 2015;277:260-71. [Crossref] [PubMed]
- Zampetaki A, Willeit P, Tilling L, et al. Prospective study on circulating MicroRNAs and risk of myocardial infarction. J Am Coll Cardiol 2012;60:290-9. [Crossref] [PubMed]
- Liebetrau C, Mollmann H, Dorr O, et al. Release kinetics of circulating muscle-enriched microRNAs in patients undergoing transcoronary ablation of septal hypertrophy. J Am Coll Cardiol 2013;62:992-8. [Crossref] [PubMed]
- Zhang W, Shang T, Huang C, et al. Plasma microRNAs serve as potential biomarkers for abdominal aortic aneurysm. Clin Biochem 2015;48:988-92. [Crossref] [PubMed]
- Ji Q, Jiang Q, Yan W, et al. Expression of circulating microRNAs in patients with ST segment elevation acute myocardial infarction. Minerva Cardioangiol 2015;63:397-402. [PubMed]
- Yao Y, Du J, Cao X, et al. Plasma levels of microRNA-499 provide an early indication of perioperative myocardial infarction in coronary artery bypass graft patients. PloS One 2014;9:e104618. [Crossref] [PubMed]
- Mooren FC, Viereck J, Kruger K, et al. Circulating microRNAs as potential biomarkers of aerobic exercise capacity. Am J Physiol Heart Circ Physiol 2014;306:H557-63. [Crossref] [PubMed]
- Olivieri F, Antonicelli R, Spazzafumo L, et al. Admission levels of circulating miR-499-5p and risk of death in elderly patients after acute non-ST elevation myocardial infarction. Int J Cardiol 2014;172:e276-8. [Crossref] [PubMed]
- Chen X, Zhang L, Su T, et al. Kinetics of plasma microRNA-499 expression in acute myocardial infarction. J Thorac Dis 2015;7:890-6. [PubMed]
- Wang F, Long G, Zhao C, et al. Plasma microRNA-133a is a new marker for both acute myocardial infarction and underlying coronary artery stenosis. J Transl Med 2013;11:222. [Crossref] [PubMed]
- Zhu GF, Yang LX, Guo RW, et al. microRNA-155 is inversely associated with severity of coronary stenotic lesions calculated by the Gensini score. Coron Artery Dis 2014;25:304-10. [Crossref] [PubMed]
- Gao H, Guddeti RR, Matsuzawa Y, et al. Plasma Levels of microRNA-145 Are Associated with Severity of Coronary Artery Disease. PloS One 2015;10:e0123477. [Crossref] [PubMed]
- Roncarati R, Viviani Anselmi C, Losi MA, et al. Circulating miR-29a, among other up-regulated microRNAs, is the only biomarker for both hypertrophy and fibrosis in patients with hypertrophic cardiomyopathy. J Am Coll Cardiol 2014;63:920-7. [Crossref] [PubMed]
- Kuehl U, Lassner D, Gast M, et al. Differential Cardiac MicroRNA Expression Predicts the Clinical Course in Human Enterovirus Cardiomyopathy. Circ Heart Fail 2015;8:605-18. [Crossref] [PubMed]
- Kin K, Miyagawa S, Fukushima S, et al. Tissue- and plasma-specific MicroRNA signatures for atherosclerotic abdominal aortic aneurysm. J Am Heart Assoc 2012;1:e000745. [Crossref] [PubMed]
- Pankratz F, Bemtgen X, Zeiser R, et al. MicroRNA-155 Exerts Cell-Specific Antiangiogenic but Proarteriogenic Effects During Adaptive Neovascularization. Circulation 2015;131:1575-89. [Crossref] [PubMed]
- Bhattachariya A, Dahan D, Ekman M, et al. Spontaneous activity and stretch-induced contractile differentiation are reduced in vascular smooth muscle of miR-143/145 knockout mice. Acta Physiol (Oxf) 2015;215:133-43. [Crossref] [PubMed]
- Thum T. Noncoding RNAs and myocardial fibrosis. Nat Rev Cardiol 2014;11:655-63. [Crossref] [PubMed]
- Kim HW, Jiang S, Ashraf M, et al. Stem cell-based delivery of Hypoxamir-210 to the infarcted heart: implications on stem cell survival and preservation of infarcted heart function. J Mol Med (Berl) 2012;90:997-1010. [Crossref] [PubMed]
- Endo K, Naito Y, Ji X, et al. MicroRNA 210 as a biomarker for congestive heart failure. Biol Pharm Bull 2013;36:48-54. [Crossref] [PubMed]
- Vogel B, Keller A, Frese KS, et al. Multivariate miRNA signatures as biomarkers for non-ischaemic systolic heart failure. Eur Heart J 2013;34:2812-22. [Crossref] [PubMed]
- Cakmak HA, Coskunpinar E, Ikitimur B, et al. The prognostic value of circulating microRNAs in heart failure: preliminary results from a genome-wide expression study. J Cardiovasc Med (Hagerstown) 2015;16:431-7. [Crossref] [PubMed]
- Devaux Y, Vausort M, McCann GP, et al. A panel of 4 microRNAs facilitates the prediction of left ventricular contractility after acute myocardial infarction. PLoS One 2013;8:e70644. [Crossref] [PubMed]
- Devaux Y, Vausort M, McCann GP, et al. MicroRNA-150: a novel marker of left ventricular remodeling after acute myocardial infarction. Circ Cardiovasc Genet 2013;6:290-8. [Crossref] [PubMed]
- Fan KL, Zhang HF, Shen J, et al. Circulating microRNAs levels in Chinese heart failure patients caused by dilated cardiomyopathy. Indian Heart J 2013;65:12-6. [Crossref] [PubMed]
- Wen P, Song D, Ye H, et al. Circulating MiR-133a as a biomarker predicts cardiac hypertrophy in chronic hemodialysis patients. PLoS One 2014;9:e103079. [Crossref] [PubMed]
- Villar AV, Garcia R, Merino D, et al. Myocardial and circulating levels of microRNA-21 reflect left ventricular fibrosis in aortic stenosis patients. Int J Cardiol 2013;167:2875-81. [Crossref] [PubMed]
- Coffey S, Williams MJ, Phillips LV, et al. Circulating microRNA Profiling Needs Further Refinement Before Clinical Use in Patients With Aortic Stenosis. J Am Heart Assoc 2015;4:e002150. [Crossref] [PubMed]
- Leung LY, Chan CP, Leung YK, et al. Comparison of miR-124-3p and miR-16 for early diagnosis of hemorrhagic and ischemic stroke. Clin Chim Acta 2014;433:139-44. [Crossref] [PubMed]
- Peng G, Yuan Y, Wu S, et al. MicroRNA let-7e Is a Potential Circulating Biomarker of Acute Stage Ischemic Stroke. Transl Stroke Res 2015;6:437-45. [Crossref] [PubMed]
- Tan KS, Armugam A, Sepramaniam S, et al. Expression profile of MicroRNAs in young stroke patients. PLoS one 2009;4:e7689. [Crossref] [PubMed]
- Kim JM, Jung KH, Chu K, et al. Atherosclerosis-Related Circulating MicroRNAs as a Predictor of Stroke Recurrence. Transl Stroke Res 2015;6:191-7. [Crossref] [PubMed]
- Tsai PC, Liao YC, Wang YS, et al. Serum microRNA-21 and microRNA-221 as potential biomarkers for cerebrovascular disease. J Vasc Res 2013;50:346-54. [Crossref] [PubMed]
- Kim J, Krichevsky A, Grad Y, et al. Identification of many microRNAs that copurify with polyribosomes in mammalian neurons. Proc Natl Acad Sci U S A 2004;101:360-5. [Crossref] [PubMed]
- Ikonomidis JS, Ivey CR, Wheeler JB, et al. Plasma biomarkers for distinguishing etiologic subtypes of thoracic aortic aneurysm disease. J Thorac Cardiovasc Surg 2013;145:1326-33. [Crossref] [PubMed]
- Ruddy JM, Jones JA, Ikonomidis JS. Pathophysiology of thoracic aortic aneurysm (TAA): is it not one uniform aorta? Role of embryologic origin. Prog Cardiovasc Dis 2013;56:68-73. [Crossref] [PubMed]
- Maegdefessel L, Azuma J, Toh R, et al. MicroRNA-21 blocks abdominal aortic aneurysm development and nicotine-augmented expansion. Sci Transl Med 2012;4:122ra22. [Crossref] [PubMed]
- Maegdefessel L, Azuma J, Tsao PS. MicroRNA-29b regulation of abdominal aortic aneurysm development. Trends Cardiovasc Med 2014;24:1-6. [Crossref] [PubMed]
- Stather PW, Sylvius N, Sidloff DA, et al. Identification of microRNAs associated with abdominal aortic aneurysms and peripheral arterial disease. Br J Surg 2015;102:755-66. [Crossref] [PubMed]
- Stather PW, Sylvius N, Wild JB, et al. Differential MicroRNA Expression Profiles in Peripheral Arterial Disease. Circ Cardiovasc Genet 2013;6:490-7. [Crossref] [PubMed]
- Manole CG, Cismasiu V, Gherghiceanu M, et al. Experimental acute myocardial infarction: telocytes involvement in neo-angiogenesis. J Cell Mol Med 2011;15:2284-96. [Crossref] [PubMed]
- Starikova I, Jamaly S, Sorrentino A, et al. Differential expression of plasma miRNAs in patients with unprovoked venous thromboembolism and healthy control individuals. Thromb Res 2015;136:566-72. [Crossref] [PubMed]
- Xiao J, Jing ZC, Ellinor PT, et al. MicroRNA-134 as a potential plasma biomarker for the diagnosis of acute pulmonary embolism. J Transl Med 2011;9:159. [Crossref] [PubMed]
- Zhang Q, Kandic I, Faughnan ME, et al. Elevated circulating microRNA-210 levels in patients with hereditary hemorrhagic telangiectasia and pulmonary arteriovenous malformations: a potential new biomarker. Biomarkers 2013;18:23-9. [Crossref] [PubMed]
- Rawal S, Manning P, Katare R. Cardiovascular microRNAs: as modulators and diagnostic biomarkers of diabetic heart disease. Cardiovasc Diabetol 2014;13:44. [Crossref] [PubMed]
- Peng H, Zhong M, Zhao W, et al. Urinary miR-29 correlates with albuminuria and carotid intima-media thickness in type 2 diabetes patients. PLoS One 2013;8:e82607. [Crossref] [PubMed]
- Hong SJ, Choi SC, Cho JY, et al. Pioglitazone increases circulating microRNA-24 with decrease in coronary neointimal hyperplasia in type 2 diabetic patients- optical coherence tomography analysis. Circ J 2015;79:880-8. [Crossref] [PubMed]
- Maegdefessel L, Spin JM, Raaz U, et al. miR-24 limits aortic vascular inflammation and murine abdominal aneurysm development. Nat Commun 2014;5:5214. [Crossref] [PubMed]
- Caporali A, Meloni M, Vollenkle C, et al. Deregulation of microRNA-503 contributes to diabetes mellitus-induced impairment of endothelial function and reparative angiogenesis after limb ischemia. Circulation 2011;123:282-91. [Crossref] [PubMed]
- Rowley AH, Pink AJ, Reindel R, et al. A study of cardiovascular miRNA biomarkers for Kawasaki disease. Pediatr Infect Dis J 2014;33:1296-9. [Crossref] [PubMed]
- Dong H, Li J, Huang L, et al. Serum MicroRNA Profiles Serve as Novel Biomarkers for the Diagnosis of Alzheimer's Disease. Dis Markers 2015;2015:625659.
- Rhodes CJ, Wharton J, Boon RA, et al. Reduced microRNA-150 is associated with poor survival in pulmonary arterial hypertension. Am J Respir Crit Care Med 2013;187:294-302. [Crossref] [PubMed]
- Sarrion I, Milian L, Juan G, et al. Role of circulating miRNAs as biomarkers in idiopathic pulmonary arterial hypertension: possible relevance of miR-23a. Oxid Med Cell Longev 2015;2015:792846.
- Lorenzen JM, Kielstein JT, Hafer C, et al. Circulating miR-210 predicts survival in critically ill patients with acute kidney injury. Clin J Am Soc Nephrol 2011;6:1540-6. [Crossref] [PubMed]
- Ferreira LR, Frade AF, Santos RH, et al. MicroRNAs miR-1, miR-133a, miR-133b, miR-208a and miR-208b are dysregulated in Chronic Chagas disease Cardiomyopathy. Int J Cardiol 2014;175:409-17. [Crossref] [PubMed]
- Kumarswamy R, Bauters C, Volkmann I, et al. Circulating long noncoding RNA, LIPCAR, predicts survival in patients with heart failure. Circ Res 2014;114:1569-75. [Crossref] [PubMed]
- Vausort M, Wagner DR, Devaux Y. Long noncoding RNAs in patients with acute myocardial infarction. Circ Res 2014;115:668-77. [Crossref] [PubMed]
- Yang Y, Cai Y, Wu G, et al. Plasma long non-coding RNA, CoroMarker, a novel biomarker for diagnosis of coronary artery disease. Clin Sci (Lond) 2015;129:675-85. [Crossref] [PubMed]
- Zhao Y, Feng G, Wang Y, et al. Regulation of apoptosis by long non-coding RNA HIF1A-AS1 in VSMCs: implications for TAA pathogenesis. Int J Clin Exp Pathol 2014;7:7643-52. [PubMed]
- Ishii N, Ozaki K, Sato H, et al. Identification of a novel non-coding RNA, MIAT, that confers risk of myocardial infarction. J Hum Genet 2006;51:1087-99. [Crossref] [PubMed]
- Yan B, Yao J, Liu JY, et al. lncRNA-MIAT regulates microvascular dysfunction by functioning as a competing endogenous RNA. Circ Res 2015;116:1143-56. [Crossref] [PubMed]
- Zhang W, Chen Y, Liu P, et al. Variants on chromosome 9p21.3 correlated with ANRIL expression contribute to stroke risk and recurrence in a large prospective stroke population. Stroke 2012;43:14-21. [Crossref] [PubMed]
- Zhou X, Han X, Wittfeldt A, et al. Long non-coding RNA ANRIL regulates inflammatory responses as a novel component of NF-kappaB pathway. RNA Biol 2016;13:98-108. [Crossref] [PubMed]
- Chiesa N, De Crescenzo A, Mishra K, et al. The KCNQ1OT1 imprinting control region and non-coding RNA: new properties derived from the study of Beckwith-Wiedemann syndrome and Silver-Russell syndrome cases. Hum Mol Genet 2012;21:10-25. [Crossref] [PubMed]
- Duong Van Huyen JP, Tible M, Gay A, et al. MicroRNAs as non-invasive biomarkers of heart transplant rejection. Eur Heart J 2014;35:3194-202. [Crossref] [PubMed]
- Wang E, Nie Y, Zhao Q, et al. Circulating miRNAs reflect early myocardial injury and recovery after heart transplantation. J Cardiothorac Surg 2013;8:165. [Crossref] [PubMed]
- Sukma Dewi I, Torngren K, Gidlof O, et al. Altered serum miRNA profiles during acute rejection after heart transplantation: potential for non-invasive allograft surveillance. J Heart Lung Transplant 2013;32:463-6. [Crossref] [PubMed]
- Willeit P, Zampetaki A, Dudek K, et al. Circulating microRNAs as novel biomarkers for platelet activation. Circ Res 2013:595-600. [PubMed]
- Flowers E, Aouizerat BE, Abbasi F, et al. Circulating microRNA-320a and microRNA-486 predict thiazolidinedione response: Moving towards precision health for diabetes prevention. Metabolism 2015;64:1051-9. [Crossref] [PubMed]
- Shantikumar S, Caporali A, Emanueli C. Role of microRNAs in diabetes and its cardiovascular complications. Cardiovasc Res 2012;93:583-93. [Crossref] [PubMed]
- Kaudewitz D, Zampetaki A, Mayr M. MicroRNA Biomarkers for Coronary Artery Disease? Curr Atheroscler Rep 2015;17:70. [Crossref] [PubMed]
- Min PK, Park J, Isaacs S, et al. Influence of Statins on Distinct Circulating MicroRNAs during Prolonged Aerobic Exercise. J Appl Physiol 1985;2016:120-20. [PubMed]
- Emilian C, Goretti E, Prospert F, et al. MicroRNAs in patients on chronic hemodialysis (MINOS study). Clin J Am Soc Nephrol 2012;7:619-23. [Crossref] [PubMed]
- Badrnya S, Baumgartner R, Assinger A. Smoking alters circulating plasma microvesicle pattern and microRNA signatures. Thromb Haemost 2014;112:128-36. [Crossref] [PubMed]
- Meder B, Backes C, Haas J, et al. Influence of the confounding factors age and sex on microRNA profiles from peripheral blood. Clin Chem 2014;60:1200-8. [Crossref] [PubMed]
- Queirós AM, Eschen C, Fliegner D, et al. Sex- and estrogen-dependent regulation of a miRNA network in the healthy and hypertrophied heart. Int J Cardio 2013;169:331-8. [Crossref] [PubMed]
- De Rosa S, Fichtlscherer S, Lehmann R, et al. Transcoronary concentration gradients of circulating microRNAs. Circulation 2011;124:1936-44. [Crossref] [PubMed]
- Białek S, Gorko D, Zajkowska A, et al. Release kinetics of circulating miRNA-208a in the early phase of myocardial infarction. Kardiol Pol 2015;73:613-9. [PubMed]
- Huang RS, Gamazon ER, Ziliak D, et al. Population differences in microRNA expression and biological implications. RNA Biol 2011;8:692-701. [Crossref] [PubMed]
- Wang X, Sundquist J, Zoller B, et al. Determination of 14 circulating microRNAs in Swedes and Iraqis with and without diabetes mellitus type 2. PLoS One 2014;9:e86792. [Crossref] [PubMed]
- Moldovan L, Batte KE, Trgovcich J, et al. Methodological challenges in utilizing miRNAs as circulating biomarkers. J Cell Mol Med 2014;18:371-90. [Crossref] [PubMed]