High tyrosine threonine kinase expression predicts a poor prognosis: a potential therapeutic target for endometrial carcinoma
Highlight box
Key findings
• Inhibition of TTK expression by a TTK inhibitor (NTRC0066-0) significantly suppressed the proliferation of the EC cells and synergistically increased the sensitivity of the EN and AN3-CA EC cell lines.
What is known and what is new?
• Previous analysis of publicly data found the overexpression of TTK molecule in EC and TTK was suggested to be used as prognostic marker for EC.
• In this study, downregulation of TTK significantly suppressed the growth of EC cells, and TTK knockdown synergistically increased the sensitivity of EC cells to the TTK inhibitor, which suggests that TTK inhibitors might have a potential use in clinical EC therapy.
What is the implication, and what should change now?
• The detailed mechanism including the inhibiting mechanism of TTK on EC cell should be further investigated in the future. The role of TTK inhibitor in combined treatment should be explores deeply.
Introduction
Endometrial carcinoma (EC), originating from the inner lining of the uterus, is the 6th most common female malignancy worldwide (1-3). Surgery is normally the first choice of treatment for women with early stage EC; however, adjuvant chemotherapy also plays an indispensable role in the treatment of early high-risk EC or high-grade EC. A study has reported on the promising efficacy of molecular-targeted therapies for human malignancies (4). For example, bevacizumab, the anti–vascular endothelial growth factor therapy agent, was approved for cancer therapy and has been shown to be effective in treating patients with advanced-stage metastatic ovarian cancer (5,6). Olaparib has been shown to have efficacy as a poly adenosine diphosphate ribose polymerase inhibitor in treating patients with relapsed ovarian cancer (7). The incidence and mortality rates of EC continue to increase globally (8,9). Thus, more effective approaches for the prediction and treatment of this disease need to be developed urgently.
As a highly regulated process involved in cell growth, the cell cycle has always been considered a driving force of tumorigenesis (10). The loss of control of the cell cycle is a hallmark of human tumors. Recently, research revealed that cell cycle–related proteins are involved in tumor development and not only regulate tumor cells but also modulate the tumor microenvironment (11-13). Consequently, great effort has been made to identify potential targets from cell cycle proteins for cancer therapies (14).
Tyrosine threonine kinase (TTK) protein kinase, which is the core modulator of the spindle assembly checkpoint (15), has emerged as one of the most important potential targets for treating cancers, including triple negative breast cancer (14,16), and the intrahepatic spread of liver cancer (17). Additionally, cyclin dependent kinase inhibitor 2A (CDKN2A) has been shown to be upregulated in multiple cancers, and it has been suggested that it be used as a biomarker for tumor immune infiltrates (18). Mutations of CDKN2A increase the sensitivity of cancer cells (in breast cancer, lung cancer, and melanoma) to TTK inhibition (18). However, currently, the targeting of cell cycle proteins for cancer therapy is in its infancy, and more efforts need to be made for its clinical application.
Previous analysis of publicly data found the overexpression of TTK molecule in EC and TTK was suggested to be used as prognostic marker for EC (19). In this study, we sought to identify potential targets for EC therapeutics through combing the publicly data and experimental results. Publicly available data from The Cancer Genome Atlas (TCGA), Genotype-Tissue Expression Project (GTEx), and Human Protein Atlas (HPA) databases were integrated and examined. We observed the abnormal activation of cell cycle-involved signaling pathways, and identified the dysregulated expression of CDKN2A and TTK and their modulating effects on the cell cycle. Further, we found that the downregulation of TTK significantly suppressed the growth of EC cells, and TTK knockdown synergistically increased the sensitivity of EC cells to the TTK inhibitor, which suggests that TTK inhibitors might have a potential use in clinical EC therapy. We present the following article in accordance with the MDAR reporting checklist (available at https://atm.amegroups.com/article/view/10.21037/atm-22-5783/rc).
Methods
Cell culture
The EC cell lines, including the EN [EC cells, DSMZ (Deutsche Sammlung von Mikroorganismen und Zellkulturen) No. ACC 564] and AN3-CA (EC cells, ATCC No. HTB-111™) cell lines, were purchased, and both cell lines were cultured at 37 ℃ in 5% carbon dioxide. The medium used for the cells was RPMI-1640 (sigma, R8758) supplemented with 20% fetal bovine serum (Gibco, 10091-148). The cells were grown in the log phase (1×105–1×106 cells/mL).
siRNA
Both the EN and AN3-CA cells were transfected with MISSION® esiRNA (SIGMA, EHU026581) using lipofectamine 2000 (ThermoFisher SCIENTIFIC, 11668019) in accordance with the manufacturer’s protocol. The cells were seeded into 6-well plates (1.5×105 cells /well). The SilencerTM Select Negative Control#1 siRNA (Thermo Fisher SCIENTIFIC, 4390843) was used as the control.
Western blot
Western blot assays were performed as described previously (15). The following primary antibodies were used: Anti-TTK/Mps1 antibody (ab24226) and Anti-beta Actin (ab6276). The following secondary antibodies were used: Goat Anti-Mouse IgG H&L (ab6708) and Goat Anti-Rabbit IgG H&L (HRP) (ab6721).
IC50 assays
Both the EN cells and AN3-CA cells, which were transfected with siNC (negative control) and siTTK respectively, were seeded into 24-well plates at a density of 5×104 cells/well in triplicate. The cells were then treated with the TTK inhibitor (MedChemExpress, NTRC0066-0) at the indicated concentrations (0.1, 1, 10, 100, and 1,000 nM). The number of cells was counted and calculated after 3 days using a counting plate under the microscope. The experiment was repeated 3 times.
Cell proliferation assays
The EN cells and AN3-CA cells were transfected with siNC and siTTK, respectively, and were seeded onto 24-well plates at a density of 5×104 cells/well in triplicate. The number of cells was counted everyday using a counting plate under the microscope. The experiment was repeated 3 times.
Public databases
The Cancer Genome Atlas (TCGA) data was downloaded from the TCGA Data Portal. And publicly available data from TCGA, Genotype-Tissue Expression Project (GTEx), and Human Protein Atlas (HPA) databases were comprehensively dissected. The study was conducted in accordance with the Declaration of Helsinki (as revised in 2013).
Statistical analysis
R studio 4.0.3 (R Foundation, Vienna, Austria) was used for the statistical analysis in this study. The gene set enrichment analysis (GSEA), and the Gene Ontology (GO), and Kyoto Encyclopedia of Genes and Genomes (KEGG) analyses of the TCGA data were carried out with the R package “ClusterProfiler” (20). The protein-protein interaction (PPI) network was analyzed using the Search Tool for Recurring Instances of Neighboring Genes (STRING) database (the medium confidence value is 0.400). The statistical significance was identified by a one-way analysis of variance or student’s t-test in this study. P values <0.05 were considered statistically significant (*, P<0.05; **, P<0.01; ***, P<0.005).
Results
The differentially expressed genes (DEGs) and related signaling pathways in EC
To explore the potential target genes for EC therapeutics, gene expression profiles of uterine corpus endometrial carcinoma (UCEC) were integrated and analyzed based on the Gene Expression Profiling Interactive Analysis (GEPIA) database and the Genotype-Tissue Expression Project (N=91; T=174) (GTEx) data. The detailed workflow of the study is shown in Figure 1A. The DEGs, including the upregulated genes [2,038] and downregulated genes [5,093], were identified and visualized in a volcano plot [P<0.05 and fold change (FC) >2 or FC <0.5] (Figure 1B). To further understand the role of these DEGs in human diseases, both the upregulated and downregulated genes were mapped onto the relevant chromosomes by a GEPIA. Notably, the distribution of the mapping genes revealed that the upregulated and downregulated genes were largely clustered on the same chromosomes, including chromosomes 19, 17, and 11 (Figure 1C,1D). These findings encouraged us to further explore the related potential molecules and signaling pathways of these DEGs in EC development.
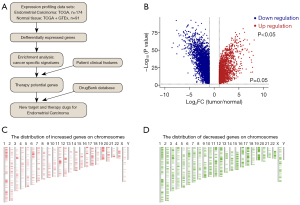
To understand the functional features of the DEGs, pathway enrichment analyses of the 2,038 upregulated genes were conducted using the GO and KEGG databases. The GO enrichment analysis revealed that the upregulated genes in the EC specimens were involved in a number of signaling pathways, such as the cell division, cell cycle, and cell proliferation pathways (Figure 2A). The KEGG analysis showed that the relevant pathways included the cell cycle, metabolic, and protein 53 singling pathways (Figure 2B). Further, using the STRING database, we generated an integrated network (with a confidence score of 0.4) with the prioritized genes from the DEGs. The enrichment for the PPI network (P<1.0e-16) was observed among the combined gene set (Figure 2C). Additionally, the GSEA showed that the cell cycle–associated signaling pathways were significantly activated in EC patients, including the Hallmark_E2F_Targets (NES =2.3), Hallmark_G2M_Checkpoint (NES =2.0), Hallmark_Glycolysis (NES =1.6), and Hallmark_MTORC1_ Signaling (NES =1.8) (Figure 2D). These results suggested that these cell cycle–involved signaling pathways and their potential modulating components could serve as potential targets for EC therapy.
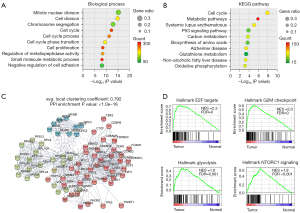
To further examine the role of the DEGs and explore their related signaling pathways, we then conducted UCEC gene expression profiling based on TCGA data sets (N=35, T=552). The upregulated genes [2,544] and downregulated genes [3,129] in the tumor specimens were modulated significantly compared to the normal control specimens (P<0.05 and FC >2 or FC <0.5; Figure S1A). Pathway enrichment analyses of the GO and KEGG databases confirmed that the cell cycle–involved signaling pathways, such as the cell cycle, and cellular senescence deoxyribonucleic acid (DNA) replication pathways, were enriched by these upregulated genes (Figure 3A,3B). The PPI network based on the top [509] upregulated genes revealed the significant PPIs (Figure S1B). Consistent with our earlier findings, the KEGG analysis results showed that the most enriched signaling pathways were the cell cycle, DNA replication, and carbon metabolism pathways (Figure S1C). Additionally, the GSEA analysis also suggested that the cell cycle-related signaling pathways were significantly activated in the EC specimens, including in the regulation of cell division, and the regulation of the meiotic cell cycle (Figure 3C). These results suggested that cell cycle–related molecules might play a critical role in EC development.
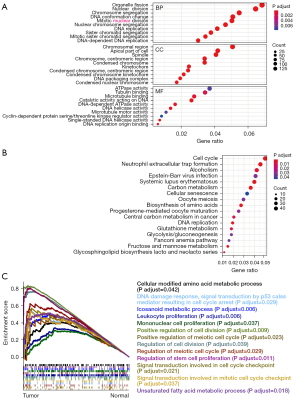
Expression of CDKN2A and TTK was upregulated in EC patients
To identify potential targets for EC therapy, the upregulated 2,038 genes in the EC specimens and the 786 genes associated with a poor prognosis in EC patients from the “GEPIA” data sets were further analyzed according to the scheme in Figure 4A. Notably, 125 genes were found to involve cell cycle regulators, transcription factors, protein kinase, metabolism enzymes, non-coding ribonucleic acids (RNAs), and epigenetic modifiers. Furthermore, both CDKN2A and TTK were significantly upregulated in the EC specimens based on the GEPIA database (Figure 4B,4C). Additionally, the expression of CDKN2A and TTK was significantly associated with the poor overall survival of EC patients, which strongly suggests that they could play a potential prognostic role in EC progression (Figure 4D,4E). The expression of CDKN2A was significantly correlated with TTK expression (Figure 4F), and both CDKN2A and TTK molecules were involved in the cell cycle signaling pathway (Figure 5).
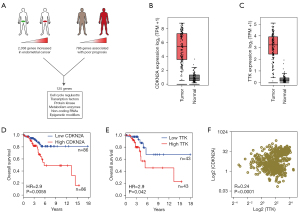
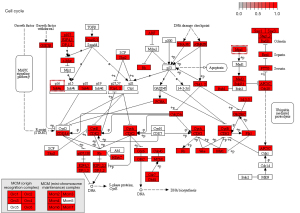
Notably, the GSEA analysis showed that TTK expression was enriched in the processes associated with the cell cycle, such as nucleolus and RNA processing (Figure 6A,6B). The GO and KEGG analyses also revealed a correlation between TTK expression and tumor progression–associated signaling pathways, including chromosome segregation, nuclear division, mitotic nuclear division, and cell cycle (Figure S2A,S2B). Thus, we hypothesized that the TTK molecules might be pivotal in the prognosis of EC patients and in the development of targeted therapies for EC.
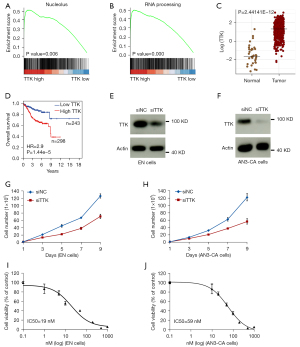
TTK knockdown increased the sensitivity of the TTK inhibitor in the EC cells
Previous studies have shown the suppressing effect of TTK inhibitors in various human malignancies (14,18), but similar results have rarely been described in EC. Our analysis of the TCGA data sets (N=35, T =552) showed that TTK was highly expressed in the EC samples (Figure 6C) and its expression was associated with the poor overall survival of EC patients (Figure 6D). To further investigate the role of TTK in EC development, we disrupted its expression using small-interfering RNA (siRNA) in the EN and AN3-CA EC cell lines. The TTK knockdown results were confirmed by Western blot analysis (Figure 6E,6F).
Following siRNA disruption, proliferation assays were performed to determine the effect of TTK on the proliferation of the EC cells. The results confirmed that TTK knockdown significantly inhibited the growth of the EN and AN3-CA cells (Figure 6G,6H). As TTK is the key regulator of the spindle assembly checkpoint in the cell cycle, we conjectured that the knockdown of TTK would affect the sensitivity of EC cells to the TTK inhibitor. IC50 (half maximal inhibitory concentration) assays were then conducted and the results showed that TTK disruption significantly decreased the 50% inhibitory concentration of the TTK inhibitor (NTRC0066-0) in both the EN and AN3-CA cells (Figure 6I,6J). Thus, our findings showed the therapeutic targeting role of TTK molecules in EC therapy.
Discussion
Recently, many studies have detailed the genomic characterization (1,3) and the clinical grades (21) of EC, but the prognosis of EC patients is still very poor, and the mortality rate of patients with recurrent or advanced disease continues to increase worldwide. Currently, surgery is the primary therapeutic choice for patients with early stage EC; however, effective therapeutic strategies for advanced or recurrent EC need to be improved (4). Molecular-targeted therapy, which has shown promising efficacy in some malignances (22,23), needs to be further explored in EC patients. Knowledge of the gene expression landscape and the molecular basis for EC development is required if novel molecular-targeted therapies are to be developed. Our research provides valuable data for the exploration of new molecular associations among proteins with potential mechanistic significance.
In this study, the integrated molecular characterization of EC genes was examined based on data from the GEPIA database (comprising 173 EC and 91 control samples). The results provided insights into the significant DEGs and signaling pathways which might have potential diagnostic and therapeutic instructions. Our analysis identified the activation of several cell cycle–involved signaling pathways, such as the cell cycle, cellular senescence, and DNA replication pathways, which innately regulate the process of cell growth, the duplication of genetic material, and cell division. These results were then confirmed using data (comprising 552 EC and 35 control samples) from TCGA database; however, these data contained less control samples. Notably, our analyzing results indicated that the signaling pathways mononuclear cell proliferation and neutrophil extracellular trap formation were enriched in EC tissue, suggested tumor immune microenvironment might be involved in the EC development, and further details will be investigated in the next step.
The use of many molecular inhibitors to target cell cycle proteins has resulted in progress in cancer treatments, such as CDK4/6 inhibitors (24-28). However, the actual application of the inhibitors of cell cycle proteins in clinics is still in its infancy (10), especially in the treatment of EC patients. The factors that influence treatment of EC patients are their responsiveness and resistance to cell cycle inhibitors. The comprehensive functions of cell cycle proteins should be delineated in the context of cancer therapy. Thus, in relation to therapeutic strategies for cancer treatment, the cell cycle-related field is worthy of further research.
Further, our functional analysis identified TTK protein kinase, which has emerged as a promising target for cancer therapies, including triple negative breast cancer therapy (14,16,29,30). As a key regulator of the spindle assembly checkpoint, TTK plays an important role in maintaining genomic integrity (31). The TTK inhibitor CFI-402,257 has been shown to suppress the growth of cancer cells and tumor cells in humans and mice (14,32-34). However, it has been reported that the genetic disruption involved in mitotic progression leads to resistance to the TTK inhibitor CFI-402257 (16). Thus, the mechanism mediating the sensitivity and resistance of TTK inhibitors should be further investigated before their clinical development. Previous study has also found that TTK was associated with an aneuploidy-tolerant state in malignancy (30). So, TTK might promote EC development via facilitating cell-cycle related mitosis. Our data revealed that TTK knockdown significantly inhibited the proliferation of EC cells, but the detailed regulating mechanism including the effect of TTK on DNA damage, apoptosis, migration and so on, need to be further investigated in the future.
CDKN2A was found to be upregulated in the EC patients in this study. The products of the CDKN2A locus are p14ARF and p16INK4A, which have a tumor suppressive function in melanoma (35,36). The somatic mutation of CDKN2A is considered a major driver of pancreatic ductal and melanoma tumorigenesis (37). Recently, CDKN2A was observed to be overexpressed in multiple cancers and it has been suggested that CDKN2A could be used as a biomarker for immune infiltration (38). CDKN2A mutations have been reported to be significantly associated with the sensitivity of triple negative breast cancer cells to TTK and the CLK inhibitor CC-671 (18). The probable prognostic and modulating role of CDKN2A should be taken into account in EC treatments with TTK inhibitors.
An enrichment analysis of the publicly available data sets and an evaluation of EC cell lines were carried out to explore the biologically relevant pathways and molecules for EC therapy. Evidence supporting a molecular-targeted strategy for EC therapy is limited, but recent progress in molecular-targeted strategies has been made in many other malignances. The disappointing results of previous clinical trials might be attributed to the administration methods of the targeted therapies (39), including monotherapies. On a related note, our study found that TTK suppression increased the sensitivity of EC cells to the TTK inhibitor (NTRC0066-0), which provides strong support for the use of TTK inhibitors in EC therapy.
The upregulation of the CDKN2A molecule was found to be associated with a poor prognosis in EC patients, and CDKN2A and TTK might serve as common biomarkers in the prognosis of EC patients. Notably, both the CDKN2A and TTK molecules were involved in the cell cycle–signaling pathway, but only high expression of the TTK molecule was found in the tumor progression–associated signaling pathways, containing chromosome segregation, nuclear division, mitotic nuclear division, cell cycle and so on. Besides, previous studies have found that inhibition of TTK molecule will increase the radiosensitivity of breast cancer and liver cancer (40,41). TTK inhibition might enhance the sensitivity of EC to radiotherapy. Totally, our study identified a potential prognostic biomarker and targeted molecule for EC treatment, which might be beneficial in exploring new therapeutic strategies.
Conclusions
Our data identified the high expressing level of TTK molecule in EC tissue based on the public databases. Furthermore, inhibition of TTK significantly suppress the proliferation of the EC cells and obviously increased the sensitivity of the EN and AN3-CA EC cell lines to TTK inhibitor (NTRC0066-0). Our findings indicated that the TTK inhibitor could be used in EC therapy. In sum, this study highlighted the potential predictive role of TTK molecules and showed that TTK molecules might serve as prospective targets for EC therapy.
Acknowledgments
Funding: This work was supported by Scientific academic backbone with off-campus supervisor project (funded by Shanghai University of Medicine and Health Sciences).
Footnote
Reporting Checklist: The authors have completed the MDAR reporting checklist. Available at https://atm.amegroups.com/article/view/10.21037/atm-22-5783/rc
Data Sharing Statement: Available at https://atm.amegroups.com/article/view/10.21037/atm-22-5783/dss
Conflicts of Interest: All authors have completed the ICMJE uniform disclosure form (available at https://atm.amegroups.com/article/view/10.21037/atm-22-5783/coif). The authors have no conflicts of interest to declare.
Ethical Statement: The authors are accountable for all aspects of the work in ensuring that questions related to the accuracy or integrity of any part of the work are appropriately investigated and resolved. The study was conducted in accordance with the Declaration of Helsinki (as revised in 2013).
Open Access Statement: This is an Open Access article distributed in accordance with the Creative Commons Attribution-NonCommercial-NoDerivs 4.0 International License (CC BY-NC-ND 4.0), which permits the non-commercial replication and distribution of the article with the strict proviso that no changes or edits are made and the original work is properly cited (including links to both the formal publication through the relevant DOI and the license). See: https://creativecommons.org/licenses/by-nc-nd/4.0/.
References
- Dou Y, Kawaler EA, Cui Zhou D, et al. Proteogenomic Characterization of Endometrial Carcinoma. Cell 2020;180:729-748.e26. [Crossref] [PubMed]
- Bray F, Ferlay J, Soerjomataram I, et al. Global cancer statistics 2018: GLOBOCAN estimates of incidence and mortality worldwide for 36 cancers in 185 countries. CA Cancer J Clin 2018;68:394-424. [Crossref] [PubMed]
- Cancer Genome Atlas Research Network. Integrated genomic characterization of endometrial carcinoma. Nature 2013;497:67-73. [Crossref] [PubMed]
- Mitamura T, Dong P, Ihira K, et al. Molecular-targeted therapies and precision medicine for endometrial cancer. Jpn J Clin Oncol 2019;49:108-20. [Crossref] [PubMed]
- Oza AM, Cook AD, Pfisterer J, et al. Standard chemotherapy with or without bevacizumab for women with newly diagnosed ovarian cancer (ICON7): overall survival results of a phase 3 randomised trial. Lancet Oncol 2015;16:928-36. [Crossref] [PubMed]
- Perren TJ, Swart AM, Pfisterer J, et al. A phase 3 trial of bevacizumab in ovarian cancer. N Engl J Med 2011;365:2484-96. [Crossref] [PubMed]
- Pujade-Lauraine E, Ledermann JA, Selle F, et al. Olaparib tablets as maintenance therapy in patients with platinum-sensitive, relapsed ovarian cancer and a BRCA1/2 mutation (SOLO2/ENGOT-Ov21): a double-blind, randomised, placebo-controlled, phase 3 trial. Lancet Oncol 2017;18:1274-84. [Crossref] [PubMed]
- Ma J, Kong FF, Yang D, et al. lncRNA MIR210HG promotes the progression of endometrial cancer by sponging miR-337-3p/137 via the HMGA2-TGF-β/Wnt pathway. Mol Ther Nucleic Acids 2021;24:905-22. [Crossref] [PubMed]
- Monsivais D, Peng J, Kang Y, et al. Activin-like kinase 5 (ALK5) inactivation in the mouse uterus results in metastatic endometrial carcinoma. Proc Natl Acad Sci U S A 2019;116:3883-92. [Crossref] [PubMed]
- Suski JM, Braun M, Strmiska V, et al. Targeting cell-cycle machinery in cancer. Cancer Cell 2021;39:759-78. [Crossref] [PubMed]
- Deng J, Wang ES, Jenkins RW, et al. CDK4/6 Inhibition Augments Antitumor Immunity by Enhancing T-cell Activation. Cancer Discov 2018;8:216-33. [Crossref] [PubMed]
- Goel S, DeCristo MJ, Watt AC, et al. CDK4/6 inhibition triggers anti-tumour immunity. Nature 2017;548:471-5. [Crossref] [PubMed]
- Zhang J, Bu X, Wang H, et al. Cyclin D-CDK4 kinase destabilizes PD-L1 via cullin 3-SPOP to control cancer immune surveillance. Nature 2018;553:91-5. [Crossref] [PubMed]
- Mason JM, Wei X, Fletcher GC, et al. Functional characterization of CFI-402257, a potent and selective Mps1/TTK kinase inhibitor, for the treatment of cancer. Proc Natl Acad Sci U S A 2017;114:3127-32. [Crossref] [PubMed]
- Abrieu A, Magnaghi-Jaulin L, Kahana JA, et al. Mps1 is a kinetochore-associated kinase essential for the vertebrate mitotic checkpoint. Cell 2001;106:83-93. [Crossref] [PubMed]
- Thu KL, Silvester J, Elliott MJ, et al. Disruption of the anaphase-promoting complex confers resistance to TTK inhibitors in triple-negative breast cancer. Proc Natl Acad Sci U S A 2018;115:E1570-7. [Crossref] [PubMed]
- Miao R, Wu Y, Zhang H, et al. Utility of the dual-specificity protein kinase TTK as a therapeutic target for intrahepatic spread of liver cancer. Sci Rep 2016;6:33121. [Crossref] [PubMed]
- Zhu D, Xu S, Deyanat-Yazdi G, et al. Synthetic Lethal Strategy Identifies a Potent and Selective TTK and CLK1/2 Inhibitor for Treatment of Triple-Negative Breast Cancer with a Compromised G1-S Checkpoint. Mol Cancer Ther 2018;17:1727-38. [Crossref] [PubMed]
- Yang Q, Yu B, Sun J. TTK, CDC25A, and ESPL1 as Prognostic Biomarkers for Endometrial Cancer. Biomed Res Int 2020;2020:4625123. [Crossref] [PubMed]
- Yu G, Wang LG, Han Y, et al. clusterProfiler: an R package for comparing biological themes among gene clusters. OMICS 2012;16:284-7. [Crossref] [PubMed]
- Horeweg N, Workel HH, Loiero D, et al. Tertiary lymphoid structures critical for prognosis in endometrial cancer patients. Nat Commun 2022;13:1373. [Crossref] [PubMed]
- Gerald D, Chintharlapalli S, Augustin HG, et al. Angiopoietin-2: an attractive target for improved antiangiogenic tumor therapy. Cancer Res 2013;73:1649-57. [Crossref] [PubMed]
- Ledermann JA, Hackshaw A, Kaye S, et al. Randomized phase II placebo-controlled trial of maintenance therapy using the oral triple angiokinase inhibitor BIBF 1120 after chemotherapy for relapsed ovarian cancer. J Clin Oncol 2011;29:3798-804. [Crossref] [PubMed]
- Cancer Genome Atlas Research Network. The Cancer Genome Atlas Pan-Cancer analysis project. Nat Genet 2013;45:1113-20. [Crossref] [PubMed]
- Álvarez-Fernández M, Malumbres M. Mechanisms of Sensitivity and Resistance to CDK4/6 Inhibition. Cancer Cell 2020;37:514-29. [Crossref] [PubMed]
- Ciznadija D, Liu Y, Pyonteck SM, et al. Cyclin D1 and cdk4 mediate development of neurologically destructive oligodendroglioma. Cancer Res 2011;71:6174-83. [Crossref] [PubMed]
- Klein ME, Kovatcheva M, Davis LE, et al. CDK4/6 Inhibitors: The Mechanism of Action May Not Be as Simple as Once Thought. Cancer Cell 2018;34:9-20. [Crossref] [PubMed]
- Hafner M, Mills CE, Subramanian K, et al. Multiomics Profiling Establishes the Polypharmacology of FDA-Approved CDK4/6 Inhibitors and the Potential for Differential Clinical Activity. Cell Chem Biol 2019;26:1067-80.e8. [Crossref] [PubMed]
- Liu Y, Laufer R, Patel NK, et al. Discovery of Pyrazolo[1,5-a]pyrimidine TTK Inhibitors: CFI-402257 is a Potent, Selective, Bioavailable Anticancer Agent. ACS Med Chem Lett 2016;7:671-5. [Crossref] [PubMed]
- Daniel J, Coulter J, Woo JH, et al. High levels of the Mps1 checkpoint protein are protective of aneuploidy in breast cancer cells. Proc Natl Acad Sci U S A 2011;108:5384-9. [Crossref] [PubMed]
- Musacchio A. The Molecular Biology of Spindle Assembly Checkpoint Signaling Dynamics. Curr Biol 2015;25:R1002-18. [Crossref] [PubMed]
- Colombo R, Caldarelli M, Mennecozzi M, et al. Targeting the mitotic checkpoint for cancer therapy with NMS-P715, an inhibitor of MPS1 kinase. Cancer Res 2010;70:10255-64. [Crossref] [PubMed]
- Kwiatkowski N, Jelluma N, Filippakopoulos P, et al. Small-molecule kinase inhibitors provide insight into Mps1 cell cycle function. Nat Chem Biol 2010;6:359-68. [Crossref] [PubMed]
- Tardif KD, Rogers A, Cassiano J, et al. Characterization of the cellular and antitumor effects of MPI-0479605, a small-molecule inhibitor of the mitotic kinase Mps1. Mol Cancer Ther 2011;10:2267-75. [Crossref] [PubMed]
- Bennett DC. Genetics of melanoma progression: the rise and fall of cell senescence. Pigment Cell Melanoma Res 2016;29:122-40. [Crossref] [PubMed]
- Zeng H, Jorapur A, Shain AH, et al. Bi-allelic Loss of CDKN2A Initiates Melanoma Invasion via BRN2 Activation. Cancer Cell 2018;34:56-68.e9. [Crossref] [PubMed]
- Kimura H, Paranal RM, Nanda N, et al. Functional CDKN2A assay identifies frequent deleterious alleles misclassified as variants of uncertain significance. Elife 2022;11:71137. [Crossref] [PubMed]
- Chen Z, Guo Y, Zhao D, et al. Comprehensive Analysis Revealed that CDKN2A is a Biomarker for Immune Infiltrates in Multiple Cancers. Front Cell Dev Biol 2021;9:808208. [Crossref] [PubMed]
- Dedes KJ, Wetterskog D, Ashworth A, et al. Emerging therapeutic targets in endometrial cancer. Nat Rev Clin Oncol 2011;8:261-71. [Crossref] [PubMed]
- Zhang H, Yao W, Zhang M, et al. TTK inhibitor promotes radiosensitivity of liver cancer cells through p21. Biochem Biophys Res Commun 2021;550:84-91. [Crossref] [PubMed]
- Chandler B C., Moubadder L, Ritter C L., et al. TTK inhibition radiosensitizes basal-like breast cancer through impaired homologous recombination. J Clin Invest 2020;130:958-73. [Crossref] [PubMed]