Research progress and clinical evaluation of histotripsy: a narrative review
Introduction
High-intensity focused ultrasound (HIFU) is an effective soft-tissue noninvasive ablation technique that was first used in the treatment of patients with Parkinson’s disease in the 1940s (1). Since then, HIFU has been gradually applied to the treatment of many solid organ diseases and has shown considerable efficacy. Traditional HIFU works based on the thermal effect, which causes coagulation thermal necrosis of the target lesion and irreversible cell death in the lesion (2). During treatment, the heating of the target lesion may also cause thermal damage to normal tissues, making it difficult to achieve an accurate treatment (3). Research teams from institutions such as the University of Michigan and the University of Washington have developed a new noninvasive treatment technique based on the cavitation effect of ultrasound, called histotripsy. Histotripsy uses pulsed HIFU with a low-duty cycle (the ratio of ultrasound on-time to total treatment time) to act on the target lesion, which mechanically destroys the cells in the lesion, homogenizes the lesion tissue, and does not cause thermal damage to the normal tissue outside the lesion (4). In addition, histotripsy also solves the problem of “thermal diffusion” that occurs when traditional HIFU is used to treat areas with rich blood supply, which has important clinical significance and application value (5,6). This article reviews the mechanism of histotripsy, the progress of animal model experiments for the clinical evaluation of histotripsy, and the clinical applications of histotripsy. We also analyze the advantages and limitations of histotripsy in order to provide a reference for future research directions, product optimization, and clinical applications. We present the following article in accordance with the Narrative Review reporting checklist (available at https://atm.amegroups.com/article/view/10.21037/atm-22-2578/rc).
Methods
A PubMed search using the search terms “histotripsy” and “mechanical high-intensity focused ultrasound” was conducted in March 2022 with no restriction on the type of article and publication date. Literature published from January 2006 to March 2022 was retrieved from the PubMed database. The retrieved publications were screened independently by 3 authors (S Li, Y Wei, and B Zhang) in 3 levels: titles, abstracts, and full texts. A fourth author (X Li) adjudicated controversial articles. The relevant information extracted from each selected article formed the framework of this review (Table 1). This article reviews histotripsy from the aspects of the mechanism, animal experiments, clinical trials, advantages, disadvantages, and optimization.
Table 1
Items | Specification |
---|---|
Date of search | March 16th, 2022 |
Databases and other sources searched | PubMed |
Search terms used | (I) Histotripsy; (II) mechanical high-intensity focused ultrasound |
Timeframe | Between 2006 and 2022 |
Inclusion and exclusion criteria | Inclusion criteria: (I) English-language articles; (II) full-text available; (III) deemed relevant from the title review |
Exclusion criteria: (I) non-English language articles; (II) editorials, letters to the editor, and abstracts | |
Selection process | (I) Selection by 3 authors (S Li, Y Wei, and B Zhang) |
(II) A fourth author (X Li) adjudicated controversial articles | |
Any additional considerations, if applicable | References of selected articles were reviewed, and those deemed relevant from the title review were included if full text was available and in English |
The mechanisms of histotripsy
There are 2 kinds of histotripsy techniques: cavitation cloud histotripsy and boiling histotripsy. Although the 2 kinds of histotripsy have different ablation mechanisms, the degree of ablation that can be achieved after treatment is similar between both techniques (7).
Cavitation cloud histotripsy
The mechanism of cavitation cloud histotripsy
The basis of cavitation cloud histotripsy is the ultrasonic cavitation effect. At a low focusing intensity of HIFU, thermal effects are dominant. With the increase of focusing intensity during HIFU, other biological effects will occur, such as ultrasonic cavitation effects (8,9). Ultrasonic cavitation refers to a series of dynamic processes, such as oscillation, compression, expansion, collapse, and closure of microbubbles excited by ultrasonic waves. This cavitation can be divided into steady-state and transient types (10). When ultrasonic waves travel through the tissue, cavitation nuclei in the tissue periodically oscillate at the frequency of the ultrasonic waves. At low ultrasonic intensity, the radial oscillation of the microbubble is controlled by ultrasonic pressure, and the volume is compressed and reduced in the positive pressure stage. In the negative pressure stage, the microbubbles are stretched and expanded; that is, the microbubbles swing left and right along the equilibrium radius, which is known as steady-state cavitation. As ultrasonic sound intensity increases, the vibration amplitude of the microbubble increases. Under this condition, the vibration of the microbubble is controlled by the inertia of the surrounding medium. The cavitation microbubble expands rapidly in the negative pressure phase and collapses rapidly in the subsequent positive pressure phase, which is known as transient cavitation (11,12). The collapse of microbubbles in transient cavitation generates extremely high-pressure shock waves on the order of gigapascals. The shock waves attenuate within 100 microns, accurately destroying the target lesion at the subcellular level (13). Cavitation cloud histotripsy uses the expansion and collapse of cavitation clouds composed of cavitation microbubbles generated by transient cavitation to disintegrate the target tissue into well-defined liquefied lesions (14-16). There are 2 mechanisms for the generation of cavitation clouds: intrinsic threshold and shock-scattering. In the intrinsic threshold mechanism, the peak negative pressure of the ultrasonic pulse directly exceeds the intrinsic threshold of tissue cavitation, resulting in cavitation clouds (17-19). The threshold value of water-based tissues (such as the thrombus, liver, kidney, heart, brain, spleen, and pancreas, and in blood and water) is around 28 MPa, as measured through ex vivo experiments (17). In the shock-scattering mechanism, the peak negative pressure of the ultrasonic pulse is lower than the cavitation threshold, and the generated microbubbles interact with the later-arriving ultrasonic waves via nonlinear sound propagation, increasing the peak amplitude and generating cavitation clouds (20). Unlike the intrinsic threshold mechanism, the peak positive pressure of the shock-scattering mechanism is higher than 50 MPa, and the peak negative pressure is about 20 MPa (20). Cavitation cloud histotripsy uses pressure levels comparable to extracorporeal shock wave lithotripsy (ESWL). However, unlike ESWL, cavitation cloud histotripsy uses pulses of 1–20 cycles (1–20 µs), and every cycle contains a rarefactional phase (<1 µs) (14). In general, cavitation cloud histotripsy has a relatively low frequency (usually from 0.75 to 1 MHz), short pulses (1–20 cycles), and a frequent pulse repetition frequency (PRF; 1 Hz–1 kHz) (14,21,22). The duty cycle of cavitation cloud histotripsy is less than 1%, which ensures there is no heat accumulation in the target area and no significant temperature increase. Kieran et al. (23) showed that the temperature in the target lesion does not reach the temperature threshold for thermal ablation during treatment with cavitation cloud histotripsy, proving the dominance of the mechanical effect of cavitation in the treatment of cavitation cloud histotripsy.
Cavitation memory
After a cavitation cloud composed of cavitation microbubbles collapses, residual microbubbles can persist for up to several seconds and function as weak cavitation nuclei in subsequent pulses (24). This phenomenon is called cavitation memory (24,25). The presence of such preexisting microbubbles, either within the focal region or along the intervening sound propagation path, can result in attenuated and scattered therapy pulses and a distorted intended focal region, which significantly affects the therapeutic effect of histotripsy and may increase the peripheral damage outside of the intended target (24,26,27). Decreasing the PRF of histotripsy allows sufficient time for residual microbubbles to dissolve between successive pulses, reducing the effects of cavitation memory (28). Previous studies have shown that, if PRF is less than or equal to 1 Hz, the influence of the cavitation memory can be neglected; however, the treatment speed of histotripsy under this condition is too low to meet clinical needs (24,28). Duryea et al. (29,30) found that adding a new set of 1,000-cycle, 1 MPa low-amplitude ultrasound sequences could produce bubble coalescence (BC), which makes residual microbubbles coalesce into large bubbles, effectively reducing cavitation memory. This method significantly reduces the effects of cavitation memory while maintaining the PRF at 100 Hz. Shi et al. (28) constructed a transducer system integrating histotripsy and BC to treat venous thrombosis. The experimental results showed that the system could significantly improve the therapeutic effect of histotripsy in the treatment of venous thrombosis. Lundt et al. (31) showed that guiding the treatment focus with electronic focal steering (EFS) allowed residual microbubbles to complete BC without adding an additional pulse sequence. These experimental results demonstrate that histotripsy with EFS can achieve BC without using a separate pulse sequence, which can accelerate ablation while minimizing energy deposition.
Factors influencing the cavitation cloud’s histotripsy effect
The size and the degree of the ablation applied to lesions produced by cavitation cloud histotripsy, along with its therapeutic efficacy, depend on pulse duration, operating frequency, PRF, and the number of pulses (32-34). Under high PRF conditions, the pulse interval is shorter than the time taken for bubble dissolution (21,23). Previously generated microbubbles collapse to form bubble fragments which last for several seconds after each pulse. New pulses generate new microbubbles, causing the microbubbles to accumulate within the target lesion and form a cavitation cloud (8,35-37). All the microbubbles in the cavitation cloud oscillate and disintegrate in interaction with the newly arrived ultrasonic waves, leading to destruction at the subcellular level (24). In addition, Vlaisavljevich et al. (38) showed that the f-number (ratio of focal length to transducer diameter) of the transducer also affects the cavitation bubble density and thus the treatment efficacy of cavitation cloud histotripsy. These experimental results showed that, when the f value is higher, the bubble density in the bubble cloud is significantly reduced; that is, the area of the cavitation cloud is reduced, which reduces the treatment efficacy of the cavitation cloud histotripsy.
In addition to the equipment and treatment setup factors mentioned above, the tissue properties of the target lesion can also affect the cavitation threshold. Lake et al. (39) performed histotripsy on various structures of the porcine kidney. The results showed that the renal collecting system had the highest therapeutic threshold followed by the renal medulla, while the renal cortex had the lowest therapeutic threshold. This finding provide the cavitation threshold of each type of tissue. Some research teams believe that tissue with higher stiffness (Young’s modulus) has a higher cavitation threshold for cavitation cloud histotripsy (37,40). Vlaisavljevich et al. (37) reported that the cavitation threshold increased significantly when the agarose concentration was increased from 0.3% to 1%, 2.5%, and 5%. Porcine skin, tongue, tendon, and cartilage (high Young’s modulus) have significantly higher cavitation thresholds than do other tissues, such as skeletal muscle, cardiac muscle, and the liver (medium Young’s modulus), while thresholds of the lungs, fat, and kidneys (low Young’s modulus) are significantly lower than the thresholds of other tissues. The experimental results of Lake et al. (39) also point to the presence of the above relationship; that is, tissues with higher fibrous connective tissue content have higher cavitation thresholds. In general, bubble expansion is reduced in tissue with increasing Young’s modulus, and smaller bubbles act as less effective reflectors for shockwaves. Therefore, in stiffer tissue, shockwaves with a higher positive pressure are required to achieve the same cavitation intrinsic threshold. Results of follow-up experiments by Vlaisavljevich et al. (41) using a phantom that could modulate tissue mechanical properties and ex vivo tissue showed that tissue stiffness and ultrasound frequency did not significantly affect the cavitation threshold. Maxwell et al. (17) reported that the viscosity and elasticity of tissue did not significantly change the cavitation threshold but changed the kinetics of bubble growth and collapse. The 2 studies mentioned above demonstrate that the intrinsic cavitation threshold does not change with the change in the mechanical properties of tissue.
Boiling histotripsy
The mechanism of boiling histotripsy
Boiling histotripsy uses shockwaves based on nonlinear propagation effects to heat the target lesion, producing a millimeter-sized boiling bubble within milliseconds (22,42,43). The shear stress created around the oscillating bubble can tear tissue (7,43-46). The bubbles further interact with the incoming shock wave to facilitate mechanical tissue separation (45). An inertial cavitation cloud can be generated between the HIFU source and the boiling bubble and extend toward the HIFU transducer due to the shock-scattering effect (42,47). Along with this cavitation cloud, additional boiling bubbles can form within the HIFU focal region through incoming wave diffraction (42). A boiling histotripsy lesion produced in soft tissue is shaped like a tadpole, consisting of a head and tail, with the head facing the HIFU transducer (Figure 1). The shear force produced by boiling bubbles on the tissue produces the “tail” part of the boiling histotripsy lesion, while the emission of shock waves and the microjetting of the inertial cavitation cloud produce the “head”-shaped lesion (47,48). Although the boiling bubbles are generated by heating, the heat is restricted to the focal region and does not diffuse into nearby tissues (7,44). This does not cause thermal tissue necrosis because the heating time of the boiling histotripsy is controlled at the millisecond level, and the duty cycle is only about 2% (44). Wang et al. (46) demonstrated that boiling histotripsy does not use thermal effects to thermally ablate the target lesions but rather uses mechanical effects to produce mechanical damage at the subcellular level in the target lesions, thus exerting a therapeutic effect. Compared to cavitation cloud histotripsy, boiling histotripsy has a higher frequency (1–3 MHz), longer pulses (3,000–10,000 cycles), and lower PRF (1–2 Hz) (7). In addition to the different mechanisms for producing mechanical damage, the sound pressure of boiling histotripsy is also different from that of cavitation cloud histotripsy. The peak positive pressure of boiling histotripsy is about 80 MPa, and the peak negative pressure is about 10 MPa, which is lower than the peak negative pressure of cavitation cloud histotripsy (14,42). It is worth noting that, unlike the randomly generated cavitation clouds produced through shock-scattering cavitation cloud histotripsy, each pulse in boiling histotripsy can produce a boiling bubble, which ensures the stability of the treatment (7,49).
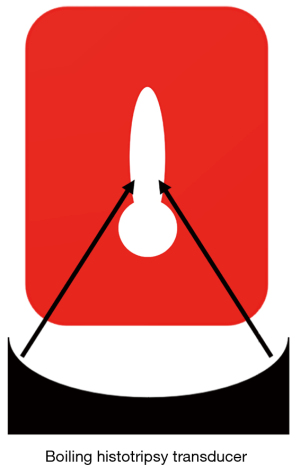
Factors influencing boiling histotripsy
The time to generate boiling bubbles depends on the output power and operating frequency of the transducer. Previous studies have shown that the higher the output power and the higher the operating frequency are, the shorter is the time to generate boiling bubbles (7,44). Khokhlova et al. (7) showed that, with the same output power, a transducer with a higher operating frequency could generate a shorter time for boiling bubbles in ex vivo liver tissue.
The size of the lesion produced by boiling histotripsy depends on the size of the ultrasonic focal point and the number of pulses (42). When the size of the ultrasonic focal point is fixed, the lesion size increases with the increase of the pulse number until the maximum lesion extent is reached. The maximum extent of the lesion is determined by the size of the ultrasonic focal point. The larger the focal size is, the greater the extent of the lesion (7,44). The size of the focal point depends on the operating frequency and size of the transducer: the lower the operating frequency of the transducer and the larger the f-number, the larger the focal point and the larger the maximum lesion extent (7,46).
The duty cycle and pulse duration can affect the degree of thermal damage to the lesions produced by boiling histotripsy (7,44). Khokhlova et al. (7) showed that in order to fully mechanically destruct ex vivo bovine heart tissue, the pulse duration should not only be longer than the time to generate a boiling bubble but should also be less than 30 ms, and the duty cycle should be less than 2%. Wang et al. (46) obtained similar results to Khokhlova et al. (7).
Application of histotripsy in animal models
Ex vivo and in vivo animal models are necessary for exploring the efficacy and safety of histotripsy before applying it to various clinical situations (21,50,51). In addition to animal models, some studies have involved the use of phantoms to explore the ex vivo effects of histotripsy and to predict in the vivo biological effects (52-55). At the macroscopic, ultrasound image, and cellular levels, the morphology of lesions in the phantom can accurately simulate the cavitation effect of soft tissue and can be used as a powerful tool for the clinical evaluation of histotripsy (8,56).
Feasibility evaluation of the mechanical ablation in histotripsy
Histotripsy uses mechanical effects to ablate the target lesion while minimizing energy diffusion during treatment, which reduces thermal tissue damage and protects adjacent structures. Roberts et al. (21) developed a focused annular array ultrasound system capable of delivering high-intensity and short-pulse energy to target lesions and of performing transcutaneous ablation in rabbit kidney tissue. Ablation experiments showed that a small number of pulses could produce sporadic lesions, manifested as focal hemorrhage and small areas of cellular damage, while a large number of pulses could cause complete destruction of the target area. The histological examination showed that increasing the number of pulses could make the tissue damage in the target area uniform and ensure a clear demarcation between the ablation and the surrounding tissue. This experiment demonstrated the feasibility of transcutaneous ablation of rabbit kidneys using cavitation cloud histotripsy without causing skin damage and extra-target damage. Parsons et al. (14) used the mechanical effect of cavitation clouds to ablate ex vivo porcine hearts. The results showed that histotripsy with higher PRF could reduce the number of residual intact cells in the lesion; however, thermal damage occurred if PRF exceeded the threshold. The above experiments demonstrate that histotripsy with appropriate parameters can completely mechanically ablate target lesions.
Thus far, animal experiments on boiling histotripsy have been less reported than have animal experiments on cavitation cloud histotripsy, and more studies are needed to evaluate the effectiveness and safety of boiling histotripsy. Khokhlova et al. (7) validated the mechanical ablation effect of boiling histotripsy using phantom and ex vivo bovine heart experiments, but this study did not perform a histological examination of the treated lesions and could not determine the extent of tissue damage. Maxwell et al. (6) constructed a boiling histotripsy system and performed ex vivo tissue experiments, successfully forming lesions at a depth of 7 cm in the bovine liver and 3–5 cm in the porcine abdominal wall. This study initially demonstrated the feasibility of boiling histotripsy for transcutaneous ablation. In an in vivo experiment (57) using a porcine liver model, all exposures resulted in elongated voids approximately 2 mm × 4 mm in size that were filled with liquefied tissue fragments without significant signs of heat damage, at a constant duty cycle (0.01) and pulse durations ranging from 1 to 20 ms. Histological examination revealed that the large blood vessels and biliary structures adjacent to the lesion were resistant to boiling histotripsy damage. The results of this study validate the feasibility of boiling histotripsy to generate mechanically ablated lesions in vivo.
Evaluation of cavitation cloud histotripsy in the treatment of benign prostatic hyperplasia (BPH)
Transurethral resection of the prostate (TURP) is the first-line treatment for BPH (58). Some research groups have tried to use minimally invasive methods, such as radiofrequency ablation based on thermal effects, to treat BPH, but the results have not met expectations (59,60). As a noninvasive treatment, histotripsy appears to be well tolerated by patients compared to other invasive treatments (61,62). Moreover, histotripsy can also accurately ablate the target lesion without causing thermal damage to the surrounding tissue. Animal experiments have shown that histotripsy may safely and effectively improve symptoms in the treatment of BPH. Hempel et al. (63) used a canine model to observe local and systemic responses to normal prostate histotripsy. The results showed that histotripsy produced consistent tissue fractionation and prostate debulking without collateral acoustic injury or clinical side effects, and it was well tolerated in the canine model. Styn et al. (64) assessed whether damage to the urethral sphincter, neurovascular bundle (NVB), and rectum occurred during histotripsy for BPH. The results showed that the NVB and sphincter were mostly undamaged, but subclinical damage of the rectum was obvious after 1,000 pulses, and the degree and severity of the damage increased with the increase of pulses. Roberts et al. (65) and Styn et al. (64) also found cases of rectal wall muscle damage in canine models treated with histotripsy prostatectomy. Before histotripsy can be used in the treatment of BPH, more studies are needed to determine a safe threshold that can ensure that surrounding tissue is not damaged.
Evaluation of cavitation cloud histotripsy in the treatment of solid tumors
Studies have shown that histotripsy has a good effect in the treatment of various solid tumors, such as those of the prostate (65-67), liver (68), bone (69), and kidney (21,70). Radiofrequency ablation has been shown to be effective in the local control of inoperable hepatocellular carcinoma (HCC), and guidelines recommend RFA as a first-line option (71-73). Mainstream liver tumor ablation methods work based on thermal effects, but radiofrequency ablation is limited by the heat sink effect of blood flow through the vascular-rich liver. Histotripsy based on mechanical effects makes up for the shortcomings of radiofrequency ablation in the treatment of liver tumors. Vlaisavljevich et al. (68) conducted experiments on transcutaneous porcine liver histotripsy. The results showed that different tissues with different mechanical strengths had different sensitivities to histotripsy. After treatment, the main blood vessels and bile ducts of the liver remained intact, but the surrounding liver parenchyma was completely destroyed. This research team then used rat models to study the long-term effects of transcutaneous liver histotripsy. The results showed that the treated lesions formed a cell-free homogenate with a clear boundary between the lesions and surrounding normal tissue. The cell-free homogenate was then replaced with the regenerated liver parenchyma, and only minor fibrous lesions remained after 28 days. In addition to experiments using histotripsy ablation on normal liver tissue, some research teams also used patient-derived xenograft mouse models to ablate tumors with histotripsy to verify the feasibility and safety of histotripsy in the treatment of liver tumors (74,75). Some experimental results showed that the tumor diameter was reduced by an average of 73% without obvious adverse events on the 26th day after histotripsy, and the tumor progression-free survival and overall survival were significantly prolonged compared with the no-treatment control group (74). Worlikar et al. (76) used histotripsy to partially ablate tumors in a rat HCC model. They showed that the tumor burden reduced after histotripsy, with near-complete resorption of the ablated tumor in 14 of the 15 (93.3%) treated rats. This experiment preliminarily verified the feasibility and safety of histotripsy in the treatment of HCC and suggested that histotripsy may stimulate the immune response, leading to local tumor cell destruction. Although the feasibility of histotripsy in the treatment of liver tumors has been experimentally confirmed, there are still notable problems, such as pulmonary hemorrhage caused by transcutaneous liver histotripsy and aberrations caused by ribs, that represent limitations to future clinical applications (77). Kim et al. (78) compared in vivo transcostal and transabdominal histotripsy for the ablation of liver tissue without aberration correction using a porcine model. The experiment showed that the abdominal wall temperature increased by 3.9±2.1 ℃ after 40 minutes of transcostal histotripsy treatment. Although transcostal histotripsy produced smaller ablation lesions than did abdominal histotripsy under the same parameters and duration of treatment, the team believed that transcostal histotripsy without aberration correction could be an effective and safe treatment. Knott et al. (79) also performed a transcostal histotripsy liver ablation experiment using a porcine model, and their results showed complete liver ablation lesions with no tissue residue in all 6 pigs. Edema was noted in the body wall overlying the ablation on T2-weighted (T2W) magnetic resonance imaging (MRI) in 5 out of 5 (1 animal did not undergo MRI), and lung discoloration in the right lower lobe was present in 5 out of 6 animals with alveolar hemorrhage. Transcostal liver histotripsy ablation was also considered feasible and effective by Knott et al. (79).
The risk of promoting tumor metastasis during histotripsy is also a concern (23). However, Styn et al. (70) used the New Zealand rabbit kidney tumor model implanted with a VX-2 tumor to carry out an experiment of histotripsy in the treatment of kidney tumors and performed a histological examination of rabbit lungs after treatment. The experiments showed no statistically significant increase in the number or density of lung metastases after histotripsy.
Evaluation of cavitation cloud histotripsy in the treatment of brain diseases
Histotripsy can potentially be used for noninvasive brain therapy due to the narrow ablation and sharp borders in the lesions it produces (80). Since the skull is highly absorptive and reflective to ultrasound, significant attenuation and aberrations as ultrasound travels through the skull are a concern (81). In order to improve the treatment precision to meet the requirements of treating brain diseases, Lee et al. (82) combined histotripsy with laser-induced focused ultrasound, achieving microcavitation smaller than 100 µm. Lee et al. (82) reported that histotripsy combined with laser-induced focused ultrasound could allow for targeted, high-precision cutting or ablation of a target lesion while minimizing damage to surrounding healthy brain tissue. The combination of histotripsy and high-resolution imaging systems can be applied to targeted therapies for brain diseases, effectively protecting the normal tissue around the lesion. In order to verify the feasibility and safety of histotripsy in the treatment of brain diseases, Sukovich et al. (83) performed an in vivo simulation experiment using porcine brains after partial craniectomy. The experiment showed that histotripsy could produce well-defined lesions of any shape and size in the cerebral cortex after partial craniectomy, and no major bleeding or other complications due to the treatment were observed. The feasibility and safety of histotripsy in the treatment of brain diseases still need to be further confirmed by in vivo experiments in animals without partial craniectomy. Recently, a transcranial MR-guided histotripsy (tcMRgHt) system using EFS to correct the aberration was developed by a research team at the University of Michigan (84). The team used the tcMRgHt system to successfully create target lesions in 2 ex vivo pig brains each encased in a human skull, without extensive hemorrhage or edema around the target. The experiment confirmed the feasibility of the system for treating head diseases and verified the compatibility of the system in the magnetic resonance (MR) environment. The team then conducted in vivo experiments using 8 pigs that had been partially craniotomized and covered with human skulls (4). Successful tissue ablation in the target zone was observed in all 8 pigs without evidence of excessive brain edema or hemorrhage outside of the target zone. Posttreatment MRI and histological examination showed a good correlation between the lesion and the target zone and a sharp demarcation between the lesions and surrounding tissue. This study demonstrated for the first time the in vivo viability of the tcMRgHt system in a porcine brain, thereby enabling further studies of the tcMRgHt system in brain surgery.
In addition, the feasibility of histotripsy in the treatment of intracerebral hemorrhage (ICH) has also been demonstrated by some studies. Patients with ICH can be treated by histotripsy to liquefy the blood clot in the brain and then by catheterization to withdraw the liquefied volume (85). Gerhardson et al. (86) showed that using histotripsy with EFS to correct the aberration can liquefy massive clots through the human skull at a rate of 16 mL/min without overheating the skull. Subsequently, Gerhardson et al. (87) further verified the feasibility and safety of histotripsy in the treatment of ICH using a porcine ICH model. The in vivo experiment showed that histotripsy could liquefy the center of the clot without directly damaging the perivascular brain tissue. All groups showed mild ischemia and gliosis in the perivascular area, and there were no death or signs of neurological dysfunction. Gerhardson et al. (85) designed a histotripsy treatment for ICH with a microhydrophone referred to as a catheter hydrophone (CH) that can be inserted into the catheter to correct the aberration. This treatment allows for the treatment of ICH without MRI. The ex vivo experiment confirmed that treatment using transcranial histotripsy to liquefy the clot followed by catheter drainage of the liquefied volume is an order of magnitude faster than are current minimally invasive catheter techniques using thrombolytic drugs.
Evaluation of cavitation cloud histotripsy in the treatment of heart diseases
Some cardiac patients are ineligible for surgery due to advanced age and many complex underlying diseases. Minimally invasive histotripsy may be an alternative treatment option for such patients in the future. Xu et al. (88) verified the safety of histotripsy in creating atrial septal and ventricular septal defects in the treatment of hypoplastic left heart syndrome by measuring the size of tissue fragments produced by histotripsy. Xu et al. also conducted preclinical studies using a canine model (89) and a porcine model (90) to generate atrial septal and ventricular septal defects, respectively. No significant adverse events related to treatment were observed due to histotripsy, further validating the feasibility and safety of histotripsy in the treatment of neonatal dysplastic heart syndrome.
Implanted prosthetic tissue valves can calcify over time and eventually fail (91). After severe calcification occurs, invasive procedures such as surgery are often required to replace the valve, but surgery is associated with significant morbidity and mortality (92). As a noninvasive treatment, histotripsy can potentially be used to treat valve calcification. Villemain et al. (93) conducted both ex vivo and in vivo sheep model experiments to evaluate the efficacy of histotripsy to significantly improve the valve opening of severe degenerative calcified bioprosthetic valves. The in vivo experiment showed that the transvalvular gradient decreased by an average of 50% after treatment (from 16.2±3.2 to 8.2±1.3 mmHg; P<0.001), with a decrease of valve stiffness (from 82.6±10 to 41.7±7 kPa; P<0.001), and an increase of valve area (from 1.10±0.1 to 1.58±0.1 cm2; P<0.001). To verify the feasibility and safety of histotripsy in the treatment of calcified aortic stenosis (CAS), Messas et al. (94) performed an in vivo experiment in a porcine model using a commercial histotripsy device (Valvosoft, Cardiawave). The experiment showed that the feasibility endpoint—the ability to deliver histotripsy transthoracically to the pig aortic valve—was successfully achieved in all treated pigs (n=15). Seven pigs developed nonsustained ventricular tachycardia (NSVT) during histotripsy treatment, but no life-threatening arrhythmias occurred. None of the pigs developed significant arrhythmia or myocardial ischemia during the 1-month follow-up. This study preliminarily verified the feasibility and safety of histotripsy in the treatment of CAS.
Evaluation of cavitation cloud histotripsy in the treatment of thrombus
In addition to the use of histotripsy in the treatment of the abovementioned diseases, thrombus therapy is also an application direction of histotripsy in the future. Thus far, arteriovenous thrombosis is mainly treated by drug thrombolysis, interventional thrombectomy, and surgical thrombectomy (95-97). However, there are still some limitations in traditional treatments of arteriovenous thromboses, such as drug thrombolysis. This treatment can lead to adverse reactions, such as dangerous bleeding, and some patients have contraindications to interventional surgery, such as a contrast agent allergy, while others cannot tolerate surgical thrombectomy (98,99). Several ex vivo and in vivo studies have demonstrated that histotripsy can completely dissolve thrombi transcutaneously (28,100-102). Maxwell et al. (27) used a porcine femoral venous thrombosis model to conduct a simulated experiment of histotripsy in the treatment of venous thrombosis. In the experiment, 10 out of 12 cases had a weakened intravascular echo, while 7 cases had improved venous blood flow and obvious thrombus ablation. Histological examination of the vessels revealed the presence of vascular endothelial denudation and minor hemorrhages in the adventitia and adjacent muscle and adipose tissue, but vessel wall perforation was not observed. This study preliminarily demonstrated the feasibility and safety of histotripsy for thrombus ablation. Studies have shown that the content of fibrous tissue in the target lesion can increase the therapeutic threshold of histotripsy (37,39,40,103). Therefore, more pulses are needed to treat aged clots than acute clots with histotripsy (27,28,104-106). A blood vessel with a higher content of fibrous connective tissue has a higher histotripsy threshold (37,39,40,103), which can protect the blood vessel from damage during the process of ablation of thrombus by histotripsy and ensure the safety of histotripsy in the treatment of thrombus. Recently, an ultrasound transducer for combined histotripsy-thrombolytic therapy was developed (107). The experiment showed that the transducer could produce sufficiently accurate cavitation clouds and thrombus ablation ex vivo. Further in vivo experiments are needed to verify the feasibility and safety of histotripsy-thrombolytic therapy in the future.
The size of the fragments generated after ablation is an important factor affecting the safety of histotripsy in the treatment of thrombosis. If the fragments are too large, serious complications, such as a pulmonary embolism, can occur. Xu et al. (88) performed ex vivo experiments using porcine atrial walls, livers, and kidneys to determine the relevant factors affecting the size of fragments generated by histotripsy. The results showed that the largest diameter of the fragments produced by 3-cycle pulse histotripsy was smaller than the size of the antiembolism filter, and the proportion of larger fragments produced by the short pulse was lower than that produced by a long pulse. This study preliminarily demonstrated the safety of histotripsy in the treatment of venous thrombosis, hypoplastic left heart syndrome, and other diseases prone to vascular embolism.
Evaluation of histotripsy in the treatment of abscesses
Superficial abscesses are most often treated with incision and drainage (108). Deep abscesses, such as abdominal abscesses, are usually treated with percutaneous catheter drainage (109). If the contents of deep abscesses are too viscous, the effectiveness of percutaneous catheter drainage significantly declines (110). A research team proposed using cavitation cloud histotripsy or boiling histotripsy to liquefy the viscous contents of the abscess to make it easier to drain through the catheter (111). After determining the appropriate parameters of cavitation cloud histotripsy and boiling histotripsy through ex vivo experiments, the team conducted an in vivo experiment in a pig abscess model. Experiments showed that both cavitation cloud histotripsy and boiling histotripsy can liquefy the abscess contents, reduce the viscosity of the contents, and destroy the fibrous septa in the abscess. Cavitation cloud histotripsy resulted in higher bacterial kill rates compared to boiling histotripsy. This study preliminarily verified the effectiveness of histotripsy in the treatment of abscesses and showed the potential for histotripsy to be an adjunct to the treatment of abscesses in the future.
Evaluation of boiling histotripsy
As mentioned above, the research of cavitation cloud histotripsy in the treatment of solid tumors has made considerable progress. Recently, some studies have been devoted to verifying the feasibility and safety of boiling histotripsy in the treatment of solid tumors through in vivo experiments. Hoogenboom et al. (112,113) performed a series of in vivo experiments of MR-guided boiling histotripsy tumor ablation using rat tumor models. The experiment (113), using a rat EL4 thymoma model alone, showed that the target tissue was completely disintegrated after boiling histotripsy ablation, with a narrow transition zone (<200 µm) containing many apoptotic cells between the disintegrated tumor tissue and the important tumor tissue. The team believes that T2W imaging is an appropriate method to evaluate treatment effects during or after boiling histotripsy since T2W imaging is highly consistent with hematoxylin and eosin-stained sections. Subsequently, the team used 3 rat tumor models of soft tissue melanoma (B16OVA), compact growing thymoma (EL4), and highly vascularized neuroblastoma (9464D) to conduct boiling histotripsy ablation experiments with 100 pulses and 200 pulses (112). Histopathology after the 100-pulse treatment showed completely disintegrated lesions in the target area with sharp borders in the compact EL4 and 9464D tumors, while for B16OVA tumors, the lesion was not completely ablated and still contained a mixture of partly viable clusters of cells, microvascular remnants, and tumor cell debris. Homogeneous liquefied lesions formed in B16OVA tumor tissue after 200 pulses of boiling histotripsy treatment. This study showed that boiling histotripsy could feasibly completely ablate tumors in vivo, but appropriate parameters needed to be selected according to the characteristics of different tumors.
Massive intraabdominal, retroperitoneal, and intramuscular hematomas can place enormous pressure on surrounding tissues, leading to serious complications, including infection, organ failure, and amputation (114,115). Currently, the treatment of massive hematoma mainly includes drainage and surgery (116). However, the drainage effect of acute blood clots is often poor, and surgical treatment may also lead to risks of recurrence, infection, and intraoperative accidents (116). Some research teams (117,118) proposed that boiling histotripsy could be used to liquefy the hematoma and make it amenable to being drained out of the body. Ponomarchuk et al. (118) used 2 boiling histotripsy regimes with longer (10 ms) and shorter (2 ms) pulses to liquefy freshly coagulated large-volume human blood samples ex vivo to evaluate the feasibility of using boiling histotripsy for hematoma treatment. Experiments showed that both boiling histotripsy regimens could successfully generate fluid-filled cavities in a hematoma model within a clinically relevant time (3–4 mL within 2–11 minutes). Most of the fragments in the liquefied cavity were smaller than 20 µm, and the largest fragment size was 150 µm, which was still smaller than the diameter of a commonly used fine needle. This study preliminarily demonstrated the feasibility of using boiling histotripsy in the treatment of hematoma. In the future, in vivo experiments are still needed to further verify the efficacy and safety of boiling histotripsy in the treatment of hematoma.
Liver transplantation is the primary and final clinical treatment for patients with inherited liver disease and end-stage liver disease (119). In the context of a donor shortage, hepatocyte transplantation is considered an effective alternative (120). However, the current hepatocyte transplantation technology has problems, such as a low engraftment level of transplanted hepatocytes (121). Pahk et al. proposed the direct injection of hepatocytes into the cavity formed by boiling histotripsy within the liver parenchyma to promote the successful uptake, proliferation, and integration of the transplanted cells into the diseased liver (122,123). The team conducted an in vivo experiment using a mouse model, which showed that the serum albumin levels of rats with zero initial serum albumin levels after treatment with this method could return to 50% normal by the seventh day (122). This study demonstrated that boiling histotripsy has promising potential for improving the outcomes of hepatocyte transplantation in the treatment of liver disease.
Evaluation of tumor immune function in histotripsy
Previous studies have shown that histotripsy can also promote tumor immunity in the tumor microenvironment. A current hypothesis is that boiling histotripsy can upregulate the number of functional CD8+ T cells and promote M1 macrophage activation to promote tumor immunity (48,124). Pahk et al. (48) demonstrated that boiling histotripsy could alter the phenotype of naive macrophages and tumor-associated macrophages in the tumor microenvironment and generate a large number of damage-associated molecular patterns (DAMP). DAMPs can stimulate powerful immune responses by binding to receptors (125,126). Hu et al. (127) found that histotripsy could generate more DAMPs and more significantly stimulated downstream immune changes, such as via the activation of dendritic cells, than did conventional HIFU based on thermal effects. Qu et al. (126) used cavitation cloud histotripsy to treat subcutaneous melanoma in mice and found that histotripsy stimulated efficient local intratumoral infiltration of innate and adaptive immune cell populations. The magnitude of immune stimulation was stronger than that of radiotherapy or thermal ablation.
Cavitation cloud histotripsy can also promote distant immune responses in tumors at untreated sites and inhibit the growth of metastases. Experiments by Worlikar et al. (128) using a rat HCC model showed that 9 out of 11 rats developed complete local tumor regression after ablation of 50–75% of the tumor volume using cavitation cloud histotripsy, whereas all untreated rats demonstrated local tumor progression and developed intrahepatic metastases. Hendricks-Wenger et al. (129) found that cavitation cloud histotripsy could increase the activation of the innate immune system by altering the pancreatic cancer immune microenvironment in a murine Pan02 tumor model. In addition, the combined treatment of checkpoint inhibitors and histotripsy increased the efficacy of checkpoint inhibitors, which is of great significance for tumor immunotherapy (130).
Clinical research progress of histotripsy
Clinical trials of histotripsy for BPH
In a phase I clinical trial (NCT01896973) conducted at 2 US medical centers in 2016 and 2017, 25 patients with dysuria due to BPH were treated with the prostate histotripsy device (Vortx Rx) manufactured by HistoSonics (131). After the histotripsy treatment, 3 cases of transient urinary retention (<3 days), 1 case of urinary retention (8 days in duration, defined as serious), a minor anal abrasion, and microscopic hematuria were considered to be the device-related adverse events. Although no debulking in prostate tissue was observed with transrectal ultrasound imaging or with endoscopic visualization and no clinically significant improvement in uroflow or postvoid residual urine occurred, the participants’ international prostate symptom score improved significantly compared to the baseline before treatment. Compared with the canine prostate transabdominal ultrasound transmission, the human transperineal ultrasound transmits more bone blocks, and the human prostate is also located deeper than is the canine prostate. Therefore, the ultrasonic pressure used in this human trial may not be sufficient to effectively destroy tissue, and histotripsy to ablate human prostate tissue may also require higher pressures or higher pulse numbers. This trial demonstrated the safety of histotripsy in humans, but improvements in equipment and parameters are needed to demonstrate the effectiveness of histotripsy for BPH.
Clinical trial of histotripsy for liver cancer
A phase I clinical trial (NCT03741088) of liver histotripsy in patients with multifocal hepatic malignant tumors was conducted in Barcelona in 2019 (132). This trial also used a clinical prototype liver histotripsy device (Vortx Rx) made by HistoSonics. The trial included 11 hepatic malignant tumors in 8 patients: 7 colorectal liver metastases in 5 patients, 2 metastatic tumors in one patient with cholangiocarcinoma, and one metastatic tumor in one patient with breast cancer and one patient with one primary HCC tumor. No major adverse events related to histotripsy occurred during the trial, demonstrating the safety of histotripsy in the treatment of malignant hepatic tumors. Local tumor regression was observed by MRI 2 months after histotripsy treatment for all tumors except for 1 mislocated tumor of 5 mm in size. Tumor markers in 1 patient with HCC and 1 patient with colorectal liver metastasis continued to decline after histotripsy treatment, and the patient with colorectal liver metastasis experienced untreated distant colorectal cancer shrinkage 8 weeks after histotripsy treatment. This trial demonstrated the safety and efficacy of human liver histotripsy and collected the first evidence that human histotripsy can cause the shrinking of distant tumors.
Clinical trial of histotripsy for CAS
A phase I clinical trial (NCT03779620) of cardiac histotripsy in 10 patients with severe calcific aortic stenosis using a histotripsy device (Valvosoft, Cardiawave) was conducted in France and the Netherlands in 2019 (133). Patients included in the trial were unsuitable for percutaneous aortic valve replacement or open-heart surgery due to their old age and severe comorbidities. This clinical trial used an external multielement-focused ultrasound transducer (700 kHz, 1.25 MHz) for up to 60 minutes of histotripsy at a PRF of 100–300 Hz, a duty cycle of 0.25%, and a peak negative pressure of 15–20 MPa to soften the calcified aortic valve. At 1 month after treatment, the aortic valve area of the 6 patients increased by an average of 27.6% (P=0.03), and the mean pressure gradient decreased by 23.5% (P=0.03). No patient experienced serious or significant adverse reactions. By the researchers’ calculations, compared with nonresponders, responders received higher focal energy and a longer duration of treatment. Responders’ treatment effects remained unchanged at 6 months after treatment. This trial initially demonstrated histotripsy for calcific aortic stenosis to be safe and effective.
Advantages and limitations of histotripsy
Advantages
- Noninvasiveness—histotripsy does not require a puncture or incision, leaving no scarring on the skin surface and reducing tumor spread caused by surgical incisions (134,135);
- Complete ablation—histotripsy can mechanically divide tumor tissue into acellular fragments, reducing the chance of local tumor recurrence (128), and the fragments can be absorbed by the body after treatment;
- Tissue selectivity—different tissues have different histotripsy thresholds. By choosing appropriate parameters, the lesion can be ablated without damaging vital blood vessels or nerves (39,40);
- Clear boundary—the target area and surrounding normal tissue form a clear boundary after histotripsy, and the transition zone is very narrow, usually only less than 1 mm (68,83);
- Immune effect—histotripsy has been shown to stimulate potent local and systemic immune responses, effectively eliminate residual tumors, inhibit distant metastases, and enhance the efficacy of immunotherapy (48,128).
Limitations
- Depth limitation—attenuation of ultrasound increases with target depth; therefore, histotripsy has limitations in treating obese patients and deeper organs (136);
- Application limitation—gas and bone block ultrasound delivery; therefore, histotripsy is unsuitable for treating obscured organs (81). Since the cavitation threshold for gas-containing organs such as the lung and gastrointestinal tract is very low, histotripsy can cause extensive damage to surrounding normal tissue;
- Metastasis possibility—currently, histotripsy can only treat local tumors, and there is still insufficient evidence to show that histotripsy can treat distant metastases or micrometastases (23). There is a theoretical risk that histotripsy may release tumor cells from the target tumor, leading to an increased risk of metastasis.
Optimization of histotripsy systems
Before clinical application, the treatment accuracy and efficiency of histotripsy still needs to be improved, and the influence of acoustic access on the treatment effect needs to be reduced to expand the application scope of histotripsy.
In order to make histotripsy applicable to the treatment of diseases that require high precision, some research teams have adopted various methods to improve the treatment precision of histotripsy. Research has shown that, when the ultrasonic pulse is applied for fewer than 2 periods in which the impact scattering is minimized, the generation of a dense bubble cloud only depends on 1 or 2 negative half periods of the applied ultrasonic pulse exceeding the inherent threshold of the medium (17). Lin et al. (18) used the intrinsic threshold mechanism to directly generate dense cavitation clouds using a single negative half-cycle peak negative pressure that exceeded the tissue intrinsic cavitation threshold. The experiment showed that this method could achieve the purpose of accurately generating damage in a small area, and the spatial extent of the damaged area could be accurately estimated through the tissue extent exceeding the intrinsic cavitation threshold.
In addition to changing the method of generating cavitation clouds to improve accuracy, Baac et al. (137) used the spatiotemporal superposition of 2 ultrasonic pulses (high frequency and low frequency) to produce a tight cavitation zone of 100 µm in water, which is an order of magnitude smaller than those obtained by the previous high-amplitude transducers. Similarly, Lin et al. (19) improved the accuracy of histotripsy by adding low-frequency pump pulses to make high-frequency probe pulses, which were lower than the intrinsic threshold of tissue cavitation, exceed the threshold. This method is also known as dual-beam histotripsy. Dense cavitation clouds are produced only when the dual-beam pulse combination exceeds an intrinsic threshold. Due to the stronger immunity of low-frequency pump pulses to attenuation and distortion, dual-beam histotripsy has a huge advantage when accuracy concerning the damage extent is highly required. In a follow-up study, Lin et al. (138) used an imaging sensor to provide high-frequency probe pulses, simplifying the dual-beam histotripsy system.
Special nanodroplets can diffuse through the tumor vasculature, accumulate preferentially in the tumor, and lower the cavitation threshold of the tumor (139). Nanodroplet-mediated histotripsy was developed to take advantage of this feature (140). Vlaisavljevich et al. (141) used perfluoropentane-encapsulated nanodroplets to selectively lower the threshold for the cavitation of tumors. By selecting appropriate parameters, cavitation could be selectively generated, and tumors could be selectively ablated.
As described above, the shear force produced by boiling bubbles, the emission of shock waves, and the microjetting of the inertial cavitation cloud produce the lesion during boiling histotripsy. The treatment accuracy of boiling histotripsy can be improved by eliminating the damage caused by the inertial cavitation cloud. Pahk et al. (142) proposed a new boiling histotripsy method without the shock-scattering effect, termed pressure-modulated shockwave histotripsy. The experimental results showed that pressure-modulated shockwave histotripsy could generate a boiling vapor bubble via localized shockwave heating and control the extent and lifetime of the boiling vapor bubble by manipulating peak pressure magnitudes and the HIFU pulse length. High-speed camera experimental results showed that, in the proposed pressure-modulated shock wave histotripsy, the boiling bubbles generated by the shock wave heating merged to form a larger bubble (on the order of hundreds of micrometers) at the HIFU focus. This merged boiling bubble then persisted within the HIFU focal region until the end of the exposure for 10, 50, or 100 ms.
Robot-assisted histotripsy is an important direction of future research. After more than 20 years of development and practice, the role of surgical robots in obtaining a clearer surgical field, filtering the doctor’s hand shake, reducing the doctor’s fatigue, and increasing the stability of surgery has been confirmed (143,144). Smolock et al. designed a software-controlled robotic system for histotripsy using a robotic arm positioner. The feasibility and safety of this system have been demonstrated through in vivo ablation experiments in the porcine liver (145) and in the porcine kidney (146). Fixing the boiling histotripsy transducer on a robotic arm with partial respiration motion compensation can reduce targeting errors caused by respiration motion (147). The ex vivo experiment (147) showed a reduction of at least 89% of the value of the targeting error during treatment, while the treatment time increased by no more than 1%. This technique is helpful for the histotripsy ablation of treatment sites that are more affected by respiratory motion. With the development of robot-assisted systems, robot-assisted histotripsy in the future can improve treatment efficiency and achieve better therapeutic effects than can ordinary histotripsy systems.
Conclusions
Histotripsy is a noninvasive, nonionizing, nonthermal, ultrasound-guided ablation technique that has broad application prospects. Histotripsy has numerous advantages over surgery and traditional HIFU. Thus far, significant progress has been made in the clinical application of histotripsy for liver tumors, BPH, and aortic valve calcification stenosis. Phase I clinical trials have demonstrated the safety and efficacy of histotripsy in the treatment of these diseases. More research is still needed to evaluate and optimize its efficacy and safety and to fully explore its mechanism of action, the pathological and immunological effects, and the short-term and long-term reactions of the body after treatment.
Acknowledgments
Funding: None.
Footnote
Reporting Checklist: The author has completed the Narrative Review reporting checklist. Available at https://atm.amegroups.com/article/view/10.21037/atm-22-2578/rc
Peer Review File: Available at https://atm.amegroups.com/article/view/10.21037/atm-22-2578/prf
Conflicts of Interest: All authors have completed the ICMJE uniform disclosure form (available at https://atm.amegroups.com/article/view/10.21037/atm-22-2578/coif). The authors have no conflicts of interest to declare.
Ethical Statement: The authors are accountable for all aspects of the work in ensuring that questions related to the accuracy or integrity of any part of the work are appropriately investigated and resolved.
Open Access Statement: This is an Open Access article distributed in accordance with the Creative Commons Attribution-NonCommercial-NoDerivs 4.0 International License (CC BY-NC-ND 4.0), which permits the non-commercial replication and distribution of the article with the strict proviso that no changes or edits are made and the original work is properly cited (including links to both the formal publication through the relevant DOI and the license). See: https://creativecommons.org/licenses/by-nc-nd/4.0/.
References
- Fry FJ, Ades HW, Fry WJ. Production of reversible changes in the central nervous system by ultrasound. Science 1958;127:83-4. [Crossref] [PubMed]
- Lee S, Kim HJ, Park HJ, et al. Morphometric analysis of high-intensity focused ultrasound-induced lipolysis on cadaveric abdominal and thigh skin. Lasers Med Sci 2017;32:1143-51. [Crossref] [PubMed]
- Ding X, Wang Y, Zhang Q, et al. Modulation of transcranial focusing thermal deposition in nonlinear HIFU brain surgery by numerical simulation. Phys Med Biol 2015;60:3975-98. [Crossref] [PubMed]
- Lu N, Gupta D, Daou BJ, et al. Transcranial Magnetic Resonance-Guided Histotripsy for Brain Surgery: Pre-clinical Investigation. Ultrasound Med Biol 2022;48:98-110. [Crossref] [PubMed]
- Aubry JF, Pauly KB, Moonen C, et al. The road to clinical use of high-intensity focused ultrasound for liver cancer: technical and clinical consensus. J Ther Ultrasound 2013;1:13. [Crossref] [PubMed]
- Maxwell AD, Yuldashev PV, Kreider W, et al. A Prototype Therapy System for Transcutaneous Application of Boiling Histotripsy. IEEE Trans Ultrason Ferroelectr Freq Control 2017;64:1542-57. [Crossref] [PubMed]
- Khokhlova TD, Canney MS, Khokhlova VA, et al. Controlled tissue emulsification produced by high intensity focused ultrasound shock waves and millisecond boiling. J Acoust Soc Am 2011;130:3498-510. [Crossref] [PubMed]
- Maxwell AD, Wang TY, Yuan L, et al. A tissue phantom for visualization and measurement of ultrasound-induced cavitation damage. Ultrasound Med Biol 2010;36:2132-43. [Crossref] [PubMed]
- Khokhlova VA, Bailey MR, Reed JA, et al. Effects of nonlinear propagation, cavitation, and boiling in lesion formation by high intensity focused ultrasound in a gel phantom. J Acoust Soc Am 2006;119:1834-48. [Crossref] [PubMed]
- Farny CH, Glynn Holt R, Roy RA. The correlation between bubble-enhanced HIFU heating and cavitation power. IEEE Trans Biomed Eng 2010;57:175-84. [Crossref] [PubMed]
- Miller DL. Overview of experimental studies of biological effects of medical ultrasound caused by gas body activation and inertial cavitation. Prog Biophys Mol Biol 2007;93:314-30. [Crossref] [PubMed]
- Vignon F, Shi WT, Powers JE, et al. Microbubble cavitation imaging. IEEE Trans Ultrason Ferroelectr Freq Control 2013;60:661-70. [Crossref] [PubMed]
- Brujan EA. The role of cavitation microjets in the therapeutic applications of ultrasound. Ultrasound Med Biol 2004;30:381-7. [Crossref] [PubMed]
- Parsons JE, Cain CA, Abrams GD, et al. Pulsed cavitational ultrasound therapy for controlled tissue homogenization. Ultrasound Med Biol 2006;32:115-29. [Crossref] [PubMed]
- Wang TY, Xu Z, Winterroth F, et al. Quantitative ultrasound backscatter for pulsed cavitational ultrasound therapy- histotripsy. IEEE Trans Ultrason Ferroelectr Freq Control 2009;56:995-1005. [Crossref] [PubMed]
- Miller DL, Averkiou MA, Brayman AA, et al. Bioeffects considerations for diagnostic ultrasound contrast agents. J Ultrasound Med 2008;27:611-32; quiz 633-6. [Crossref] [PubMed]
- Maxwell AD, Cain CA, Hall TL, et al. Probability of cavitation for single ultrasound pulses applied to tissues and tissue-mimicking materials. Ultrasound Med Biol 2013;39:449-65. [Crossref] [PubMed]
- Lin KW, Kim Y, Maxwell AD, et al. Histotripsy beyond the intrinsic cavitation threshold using very short ultrasound pulses: microtripsy. IEEE Trans Ultrason Ferroelectr Freq Control 2014;61:251-65. [Crossref] [PubMed]
- Lin KW, Duryea AP, Kim Y, et al. Dual-beam histotripsy: a low-frequency pump enabling a high-frequency probe for precise lesion formation. IEEE Trans Ultrason Ferroelectr Freq Control 2014;61:325-40. [Crossref] [PubMed]
- Maxwell AD, Wang TY, Cain CA, et al. Cavitation clouds created by shock scattering from bubbles during histotripsy. J Acoust Soc Am 2011;130:1888-98. [Crossref] [PubMed]
- Roberts WW, Hall TL, Ives K, et al. Pulsed cavitational ultrasound: a noninvasive technology for controlled tissue ablation (histotripsy) in the rabbit kidney. J Urol 2006;175:734-8. [Crossref] [PubMed]
- Xu Z, Ludomirsky A, Eun LY, et al. Controlled ultrasound tissue erosion. IEEE Trans Ultrason Ferroelectr Freq Control 2004;51:726-36. [Crossref] [PubMed]
- Kieran K, Hall TL, Parsons JE, et al. Refining histotripsy: defining the parameter space for the creation of nonthermal lesions with high intensity, pulsed focused ultrasound of the in vitro kidney. J Urol 2007;178:672-6. [Crossref] [PubMed]
- Wang TY, Xu Z, Hall TL, et al. An efficient treatment strategy for histotripsy by removing cavitation memory. Ultrasound Med Biol 2012;38:753-66. [Crossref] [PubMed]
- Flynn HG, Church CC. A mechanism for the generation of cavitation maxima by pulsed ultrasound. J Acoust Soc Am 1984;76:505-12. [Crossref] [PubMed]
- Lundt JE, Allen SP, Shi J, et al. Non-invasive, Rapid Ablation of Tissue Volume Using Histotripsy. Ultrasound Med Biol 2017;43:2834-47. [Crossref] [PubMed]
- Maxwell AD, Owens G, Gurm HS, et al. Noninvasive treatment of deep venous thrombosis using pulsed ultrasound cavitation therapy (histotripsy) in a porcine model. J Vasc Interv Radiol 2011;22:369-77. [Crossref] [PubMed]
- Shi A, Lundt J, Deng Z, et al. Integrated Histotripsy and Bubble Coalescence Transducer for Thrombolysis. Ultrasound Med Biol 2018;44:2697-709. [Crossref] [PubMed]
- Duryea AP, Cain CA, Roberts WW, et al. Removal of residual cavitation nuclei to enhance histotripsy fractionation of soft tissue. IEEE Trans Ultrason Ferroelectr Freq Control 2015;62:2068-78. [Crossref] [PubMed]
- Duryea AP, Cain CA, Tamaddoni HA, et al. Removal of residual nuclei following a cavitation event using low-amplitude ultrasound. IEEE Trans Ultrason Ferroelectr Freq Control 2014;61:1619-26. [Crossref] [PubMed]
- Lundt J, Hall T, Rao A, et al. Coalescence of residual histotripsy cavitation nuclei using low-gain regions of the therapy beam during electronic focal steering. Phys Med Biol 2018;63:225010. [Crossref] [PubMed]
- Nightingale KR, Church CC, Harris G, et al. Conditionally Increased Acoustic Pressures in Nonfetal Diagnostic Ultrasound Examinations Without Contrast Agents: A Preliminary Assessment. J Ultrasound Med 2015;34:1-41. [Crossref] [PubMed]
- Edsall C, Ham E, Holmes H, et al. Effects of frequency on bubble-cloud behavior and ablation efficiency in intrinsic threshold histotripsy. Phys Med Biol 2021; [Crossref] [PubMed]
- Schade GR, Styn NR, Ives KA, et al. Prostate histotripsy: evaluation of prostatic urethral treatment parameters in a canine model. BJU Int 2014;113:498-503. [Crossref] [PubMed]
- Ikeda T, Yoshizawa S, Tosaki M, et al. Cloud cavitation control for lithotripsy using high intensity focused ultrasound. Ultrasound Med Biol 2006;32:1383-97. [Crossref] [PubMed]
- Maeda K, Colonius T. Bubble cloud dynamics in an ultrasound field. J Fluid Mech 2019;862:1105-34. [Crossref] [PubMed]
- Vlaisavljevich E, Maxwell A, Warnez M, et al. Histotripsy-induced cavitation cloud initiation thresholds in tissues of different mechanical properties. IEEE Trans Ultrason Ferroelectr Freq Control 2014;61:341-52. [Crossref] [PubMed]
- Vlaisavljevich E, Gerhardson T, Hall T, et al. Effects of f-number on the histotripsy intrinsic threshold and cavitation bubble cloud behavior. Phys Med Biol 2017;62:1269-90. [Crossref] [PubMed]
- Lake AM, Xu Z, Wilkinson JE, et al. Renal ablation by histotripsy--does it spare the collecting system? J Urol 2008;179:1150-4. [Crossref] [PubMed]
- Vlaisavljevich E, Kim Y, Owens G, et al. Effects of tissue mechanical properties on susceptibility to histotripsy-induced tissue damage. Phys Med Biol 2014;59:253-70. [Crossref] [PubMed]
- Vlaisavljevich E, Lin KW, Maxwell A, et al. Effects of ultrasound frequency and tissue stiffness on the histotripsy intrinsic threshold for cavitation. Ultrasound Med Biol 2015;41:1651-67. [Crossref] [PubMed]
- Pahk KJ, Gélat P, Sinden D, et al. Numerical and Experimental Study of Mechanisms Involved in Boiling Histotripsy. Ultrasound Med Biol 2017;43:2848-61. [Crossref] [PubMed]
- Canney MS, Khokhlova VA, Bessonova OV, et al. Shock-induced heating and millisecond boiling in gels and tissue due to high intensity focused ultrasound. Ultrasound Med Biol 2010;36:250-67. [Crossref] [PubMed]
- Simon JC, Sapozhnikov OA, Khokhlova VA, et al. Ultrasonic atomization of tissue and its role in tissue fractionation by high intensity focused ultrasound. Phys Med Biol 2012;57:8061-78. [Crossref] [PubMed]
- Pahk KJ, de Andrade MO, Gélat P, et al. Mechanical damage induced by the appearance of rectified bubble growth in a viscoelastic medium during boiling histotripsy exposure. Ultrason Sonochem 2019;53:164-77. [Crossref] [PubMed]
- Wang YN, Khokhlova T, Bailey M, et al. Histological and biochemical analysis of mechanical and thermal bioeffects in boiling histotripsy lesions induced by high intensity focused ultrasound. Ultrasound Med Biol 2013;39:424-38. [Crossref] [PubMed]
- Pahk KJ, Lee S, Gélat P, et al. The interaction of shockwaves with a vapour bubble in boiling histotripsy: The shock scattering effect. Ultrason Sonochem 2021;70:105312. [Crossref] [PubMed]
- Pahk KJ, Shin CH, Bae IY, et al. Boiling Histotripsy-induced Partial Mechanical Ablation Modulates Tumour Microenvironment by Promoting Immunogenic Cell Death of Cancers. Sci Rep 2019;9:9050. [Crossref] [PubMed]
- Hoogenboom M, Eikelenboom D, den Brok MH, et al. Mechanical high-intensity focused ultrasound destruction of soft tissue: working mechanisms and physiologic effects. Ultrasound Med Biol 2015;41:1500-17. [Crossref] [PubMed]
- Tran BC, Seo J, Hall TL, et al. Microbubble-enhanced cavitation for noninvasive ultrasound surgery. IEEE Trans Ultrason Ferroelectr Freq Control 2003;50:1296-304. [Crossref] [PubMed]
- Xu Z, Fowlkes JB, Rothman ED, et al. Controlled ultrasound tissue erosion: the role of dynamic interaction between insonation and microbubble activity. J Acoust Soc Am 2005;117:424-35. [Crossref] [PubMed]
- Vlaisavljevich E, Maxwell A, Mancia L, et al. Visualizing the Histotripsy Process: Bubble Cloud-Cancer Cell Interactions in a Tissue-Mimicking Environment. Ultrasound Med Biol 2016;42:2466-77. [Crossref] [PubMed]
- Nanda Kumar Y, Singh Z, Wang YN, et al. Development of Tough Hydrogel Phantoms to Mimic Fibrous Tissue for Focused Ultrasound Therapies. Ultrasound Med Biol 2022;48:1762-77. [Crossref] [PubMed]
- Wang TY, Hall TL, Xu Z, et al. Imaging feedback of histotripsy treatments using ultrasound shear wave elastography. IEEE Trans Ultrason Ferroelectr Freq Control 2012;59:1167-81. [Crossref] [PubMed]
- Lafon C, Zderic V, Noble ML, et al. Gel phantom for use in high-intensity focused ultrasound dosimetry. Ultrasound Med Biol 2005;31:1383-9. [Crossref] [PubMed]
- Peek AT, Thomas GPL, Leotta DF, et al. Robust and durable aberrative and absorptive phantom for therapeutic ultrasound applications. J Acoust Soc Am 2022;151:3007. [Crossref] [PubMed]
- Khokhlova TD, Wang YN, Simon JC, et al. Ultrasound-guided tissue fractionation by high intensity focused ultrasound in an in vivo porcine liver model. Proc Natl Acad Sci U S A 2014;111:8161-6. [Crossref] [PubMed]
- Mayer EK, Kroeze SG, Chopra S, et al. Examining the 'gold standard': a comparative critical analysis of three consecutive decades of monopolar transurethral resection of the prostate (TURP) outcomes. BJU Int 2012;110:1595-601. [Crossref] [PubMed]
- Diri MA, Gul M. Effect of bipolar radiofrequency thermotherapy on benign prostate hyperplasia. Andrologia 2020;52:e13467. [Crossref] [PubMed]
- Liu R, Duan S, Cao H, et al. A pilot study of the shapes of ablation lesions in the canine prostate by laser, radiofrequency and microwave and their clinical significance. PLoS One 2020;15:e0223229. [Crossref] [PubMed]
- Schade GR, Hall TL, Roberts WW. Urethral-sparing histotripsy of the prostate in a canine model. Urology 2012;80:730-5. [Crossref] [PubMed]
- Schade GR, Keller J, Ives K, et al. Histotripsy focal ablation of implanted prostate tumor in an ACE-1 canine cancer model. J Urol 2012;188:1957-64. [Crossref] [PubMed]
- Hempel CR, Hall TL, Cain CA, et al. Histotripsy fractionation of prostate tissue: local effects and systemic response in a canine model. J Urol 2011;185:1484-9. [Crossref] [PubMed]
- Styn N, Hall TL, Fowlkes JB, et al. Histotripsy homogenization of the prostate: thresholds for cavitation damage of periprostatic structures. J Endourol 2011;25:1531-5. [Crossref] [PubMed]
- Roberts WW, Teofilovic D, Jahnke RC, et al. Histotripsy of the prostate using a commercial system in a canine model. J Urol 2014;191:860-5. [Crossref] [PubMed]
- Darnell SE, Hall TL, Tomlins SA, et al. Histotripsy of the Prostate in a Canine Model: Characterization of Post-Therapy Inflammation and Fibrosis. J Endourol 2015;29:810-5. [Crossref] [PubMed]
- Hall TL, Hempel CR, Wojno K, et al. Histotripsy of the prostate: dose effects in a chronic canine model. Urology 2009;74:932-7. [Crossref] [PubMed]
- Vlaisavljevich E, Kim Y, Allen S, et al. Image-guided non-invasive ultrasound liver ablation using histotripsy: feasibility study in an in vivo porcine model. Ultrasound Med Biol 2013;39:1398-409. [Crossref] [PubMed]
- Arnold L, Hendricks-Wenger A, Coutermarsh-Ott S, et al. Histotripsy Ablation of Bone Tumors: Feasibility Study in Excised Canine Osteosarcoma Tumors. Ultrasound Med Biol 2021;47:3435-46. [Crossref] [PubMed]
- Styn NR, Hall TL, Fowlkes JB, et al. Histotripsy of renal implanted VX-2 tumor in a rabbit model: investigation of metastases. Urology 2012;80:724-9. [Crossref] [PubMed]
- European Association For The Study Of The Liver. EASL-EORTC clinical practice guidelines: management of hepatocellular carcinoma. J Hepatol 2012;56:908-43. Erratum in: J Hepatol 2012 Jun;56(6):1430. [Crossref] [PubMed]
- Kim N, Cheng J, Jung I, et al. Stereotactic body radiation therapy vs. radiofrequency ablation in Asian patients with hepatocellular carcinoma. J Hepatol 2020;73:121-9. [Crossref] [PubMed]
- Heimbach JK, Kulik LM, Finn RS, et al. AASLD guidelines for the treatment of hepatocellular carcinoma. Hepatology 2018;67:358-80. [Crossref] [PubMed]
- Hendricks-Wenger A, Saunier S, Simon A, et al. Histotripsy for the Treatment of Cholangiocarcinoma in a Patient-Derived Xenograft Mouse Model. Ultrasound Med Biol 2022;48:293-303. [Crossref] [PubMed]
- Hendricks-Wenger A, Weber P, Simon A, et al. Histotripsy for the Treatment of Cholangiocarcinoma Liver Tumors: In vivo Feasibility and Ex vivo Dosimetry Study. IEEE Trans Ultrason Ferroelectr Freq Control 2021;68:2953-64. [Crossref] [PubMed]
- Worlikar T, Mendiratta-Lala M, Vlaisavljevich E, et al. Effects of Histotripsy on Local Tumor Progression in an in vivo Orthotopic Rodent Liver Tumor Model. BME Front 2020;2020:9830304. [Crossref] [PubMed]
- Vlaisavljevich E, Greve J, Cheng X, et al. Non-Invasive Ultrasound Liver Ablation Using Histotripsy: Chronic Study in an In vivo Rodent Model. Ultrasound Med Biol 2016;42:1890-902. [Crossref] [PubMed]
- Kim Y, Vlaisavljevich E, Owens GE, et al. In vivo transcostal histotripsy therapy without aberration correction. Phys Med Biol 2014;59:2553-68. [Crossref] [PubMed]
- Knott EA, Longo KC, Vlaisavljevich E, et al. Transcostal Histotripsy Ablation in an In vivo Acute Hepatic Porcine Model. Cardiovasc Intervent Radiol 2021;44:1643-50. [Crossref] [PubMed]
- Kim Y, Hall TL, Xu Z, et al. Transcranial histotripsy therapy: a feasibility study. IEEE Trans Ultrason Ferroelectr Freq Control 2014;61:582-93. [Crossref]
- Ammi AY, Mast TD, Huang IH, et al. Characterization of ultrasound propagation through ex-vivo human temporal bone. Ultrasound Med Biol 2008;34:1578-89. [Crossref] [PubMed]
- Lee T, Luo W, Li Q, et al. Laser-Induced Focused Ultrasound for Cavitation Treatment: Toward High-Precision Invisible Sonic Scalpel. Small 2017; [Crossref] [PubMed]
- Sukovich JR, Cain CA, Pandey AS, et al. In vivo histotripsy brain treatment. J Neurosurg 2018; Epub ahead of print. [Crossref] [PubMed]
- Lu N, Hall TL, Choi D, et al. Transcranial MR-Guided Histotripsy System. IEEE Trans Ultrason Ferroelectr Freq Control 2021;68:2917-29. [Crossref] [PubMed]
- Gerhardson T, Sukovich JR, Pandey AS, et al. Catheter Hydrophone Aberration Correction for Transcranial Histotripsy Treatment of Intracerebral Hemorrhage: Proof-of-Concept. IEEE Trans Ultrason Ferroelectr Freq Control 2017;64:1684-97. [Crossref] [PubMed]
- Gerhardson T, Sukovich JR, Pandey AS, et al. Effect of Frequency and Focal Spacing on Transcranial Histotripsy Clot Liquefaction, Using Electronic Focal Steering. Ultrasound Med Biol 2017;43:2302-17. [Crossref] [PubMed]
- Gerhardson T, Sukovich JR, Chaudhary N, et al. Histotripsy Clot Liquefaction in a Porcine Intracerebral Hemorrhage Model. Neurosurgery 2020;86:429-36. [Crossref] [PubMed]
- Xu Z, Fan Z, Hall TL, et al. Size measurement of tissue debris particles generated from pulsed ultrasound cavitational therapy-histotripsy. Ultrasound Med Biol 2009;35:245-55. [Crossref] [PubMed]
- Xu Z, Owens G, Gordon D, et al. Noninvasive creation of an atrial septal defect by histotripsy in a canine model. Circulation 2010;121:742-9. [Crossref] [PubMed]
- Owens GE, Miller RM, Owens ST, et al. Intermediate-term effects of intracardiac communications created noninvasively by therapeutic ultrasound (histotripsy) in a porcine model. Pediatr Cardiol 2012;33:83-9. [Crossref] [PubMed]
- Gössl M, Rihal CS. Percutaneous treatment of aortic and mitral valve paravalvular regurgitation. Curr Cardiol Rep 2013;15:388. [Crossref] [PubMed]
- Potter DD, Sundt TM 3rd, Zehr KJ, et al. Risk of repeat mitral valve replacement for failed mitral valve prostheses. Ann Thorac Surg 2004;78:67-72; discussion 67-72. [Crossref] [PubMed]
- Villemain O, Robin J, Bel A, et al. Pulsed Cavitational Ultrasound Softening: a new non-invasive therapeutic approach of calcified bioprosthetic valve stenosis. JACC Basic Transl Sci 2017;2:372-83. [Crossref] [PubMed]
- Messas E, Rémond MC, Goudot G, et al. Feasibility and safety of non-invasive ultrasound therapy (NIUT) on porcine aortic valve. Phys Med Biol 2020;65:215004. [Crossref] [PubMed]
- Feinberg J, Nielsen EE, Jakobsen JC. Thrombolysis for acute upper extremity deep vein thrombosis. Cochrane Database Syst Rev 2017;12:CD012175. [Crossref] [PubMed]
- Girona M, Säly C, Makaloski V, et al. Catheter-Directed Thrombolysis for Postpartum Deep Venous Thrombosis. Front Cardiovasc Med 2022;9:814057. [Crossref] [PubMed]
- Robertson L, McBride O, Burdess A. Pharmacomechanical thrombectomy for iliofemoral deep vein thrombosis. Cochrane Database Syst Rev 2016;11:CD011536. [Crossref] [PubMed]
- Kalaitzopoulos DR, Panagopoulos A, Samant S, et al. Management of venous thromboembolism in pregnancy. Thromb Res 2022;211:106-13. [Crossref] [PubMed]
- Sandercock PAG, Ricci S. Controversies in Thrombolysis. Curr Neurol Neurosci Rep 2017;17:60. [Crossref] [PubMed]
- Bollen V, Hendley SA, Paul JD, et al. In Vitro Thrombolytic Efficacy of Single- and Five-Cycle Histotripsy Pulses and rt-PA. Ultrasound Med Biol 2020;46:336-49. [Crossref] [PubMed]
- Hendley SA, Bollen V, Anthony GJ, et al. In vitro assessment of stiffness-dependent histotripsy bubble cloud activity in gel phantoms and blood clots. Phys Med Biol 2019;64:145019. [Crossref] [PubMed]
- Bader KB, Hendley SA, Bollen V. Assessment of Collaborative Robot (Cobot)-Assisted Histotripsy for Venous Clot Ablation. IEEE Trans Biomed Eng 2021;68:1220-8. [Crossref] [PubMed]
- Cooper M, Zhen X, Rothman ED, Levin AM, Advincula AP, Fowlkes JB, et al., editors. Controlled ultrasound tissue erosion: the effects of tissue type, exposure parameters and the role of dynamic microbubble activity. IEEE Ultrasonics Symposium, 2004. 23-27 August 2004; Montreal, Quebec, Canada. IEEE, 2004.
- Zhang X, Owens GE, Cain CA, et al. Histotripsy Thrombolysis on Retracted Clots. Ultrasound Med Biol 2016;42:1903-18. [Crossref] [PubMed]
- Xie H, Kim K, Aglyamov SR, et al. Staging deep venous thrombosis using ultrasound elasticity imaging: animal model. Ultrasound Med Biol 2004;30:1385-96. [Crossref] [PubMed]
- Feghhi S, Sniadecki NJ. Mechanobiology of platelets: techniques to study the role of fluid flow and platelet retraction forces at the micro- and nano-scale. Int J Mol Sci 2011;12:9009-30. [Crossref] [PubMed]
- Maxwell AD, Haworth KJ, Holland CK, et al. Design and Characterization of an Ultrasound Transducer for Combined Histotripsy-Thrombolytic Therapy. IEEE Trans Ultrason Ferroelectr Freq Control 2022;69:156-65. [Crossref] [PubMed]
- Mistry RD. Skin and soft tissue infections. Pediatr Clin North Am 2013;60:1063-82. [Crossref] [PubMed]
- Rivera-Sanfeliz G. Percutaneous abdominal abscess drainage: a historical perspective. AJR Am J Roentgenol 2008;191:642-3. [Crossref] [PubMed]
- Lorenz J, Thomas JL. Complications of percutaneous fluid drainage. Semin Intervent Radiol 2006;23:194-204. [Crossref] [PubMed]
- Matula TJ, Wang YN, Khokhlova T, et al. Treating Porcine Abscesses with Histotripsy: A Pilot Study. Ultrasound Med Biol 2021;47:603-19. [Crossref] [PubMed]
- Hoogenboom M, Eikelenboom DC, van den Bijgaart RJE, et al. Impact of MR-guided boiling histotripsy in distinct murine tumor models. Ultrason Sonochem 2017;38:1-8. [Crossref] [PubMed]
- Hoogenboom M, Eikelenboom D, den Brok MH, et al. In vivo MR guided boiling histotripsy in a mouse tumor model evaluated by MRI and histopathology. NMR Biomed 2016;29:721-31. [Crossref] [PubMed]
- Bovonratwet P, Fu MC, Tyagi V, et al. Incidence, Risk Factors, and Clinical Implications of Postoperative Hematoma Requiring Reoperation Following Anterior Cervical Discectomy and Fusion. Spine (Phila Pa 1976) 2019;44:543-9. [Crossref] [PubMed]
- Chung KT. Intra-Abdominal Hematoma Following Enoxaparin Injection. Clin Med Insights Case Rep 2016;9:35-8. [Crossref] [PubMed]
- Li Y, Liu Y, Li R, et al. Histotripsy Liquefaction of Large Hematoma for Intracerebral Hemorrhage Using Millisecond-Length Ultrasound Pulse Groups Combined With Fundamental and Second Harmonic Superposition: A Preliminary Study. Ultrasound Med Biol 2020;46:1244-57. [Crossref] [PubMed]
- Khokhlova TD, Kucewicz JC, Ponomarchuk EM, et al. Effect of Stiffness of Large Extravascular Hematomas on Their Susceptibility to Boiling Histotripsy Liquefaction in Vitro. Ultrasound Med Biol 2020;46:2007-16. [Crossref] [PubMed]
- Ponomarchuk EM, Rosnitskiy PB, Khokhlova TD, et al. Ultrastructural Analysis of Volumetric Histotripsy Bio-effects in Large Human Hematomas. Ultrasound Med Biol 2021;47:2608-21. [Crossref] [PubMed]
- Chaubal G, Nanavati AJ, Biradar V, et al. Monosegment Liver Allografts for Liver Transplantation in Infants Weighing Less Than 6 kg: An Initial Indian Experience. Transplant Proc 2021;53:1670-3. [Crossref] [PubMed]
- Russo FP, Parola M. Stem and progenitor cells in liver regeneration and repair. Cytotherapy 2011;13:135-44. [Crossref] [PubMed]
- Zhou H, Dong X, Kabarriti R, et al. Single liver lobe repopulation with wildtype hepatocytes using regional hepatic irradiation cures jaundice in Gunn rats. PLoS One 2012;7:e46775. [Crossref] [PubMed]
- Pahk KJ, Mohammad GH, Malago M, et al. A Novel Approach to Ultrasound-Mediated Tissue Decellularization and Intra-Hepatic Cell Delivery in Rats. Ultrasound Med Biol 2016;42:1958-67. [Crossref] [PubMed]
- Pahk KJ, Dhar DK, Malago M, Saffari N. Ultrasonic Histotripsy for Tissue Therapy. J Phys Conf Ser 2015;581:012001. [Crossref]
- Singh MP, Sethuraman SN, Miller C, et al. Boiling histotripsy and in-situ CD40 stimulation improve the checkpoint blockade therapy of poorly immunogenic tumors. Theranostics 2021;11:540-54. [Crossref] [PubMed]
- Schade GR, Wang YN, D'Andrea S, et al. Boiling Histotripsy Ablation of Renal Cell Carcinoma in the Eker Rat Promotes a Systemic Inflammatory Response. Ultrasound Med Biol 2019;45:137-47. [Crossref] [PubMed]
- Qu S, Worlikar T, Felsted AE, et al. Non-thermal histotripsy tumor ablation promotes abscopal immune responses that enhance cancer immunotherapy. J Immunother Cancer 2020;8:e000200. [Crossref] [PubMed]
- Hu Z, Yang XY, Liu Y, et al. Release of endogenous danger signals from HIFU-treated tumor cells and their stimulatory effects on APCs. Biochem Biophys Res Commun 2005;335:124-31. [Crossref] [PubMed]
- Worlikar T, Zhang M, Ganguly A, et al. Impact of Histotripsy on Development of Intrahepatic Metastases in a Rodent Liver Tumor Model. Cancers (Basel) 2022;14:1612. [Crossref] [PubMed]
- Hendricks-Wenger A, Sereno J, Gannon J, et al. Histotripsy Ablation Alters the Tumor Microenvironment and Promotes Immune System Activation in a Subcutaneous Model of Pancreatic Cancer. IEEE Trans Ultrason Ferroelectr Freq Control 2021;68:2987-3000. [Crossref] [PubMed]
- Hendricks-Wenger A, Hutchison R, Vlaisavljevich E, et al. Immunological Effects of Histotripsy for Cancer Therapy. Front Oncol 2021;11:681629. [Crossref] [PubMed]
- Schuster TG, Wei JT, Hendlin K, et al. Histotripsy Treatment of Benign Prostatic Enlargement Using the Vortx R(x) System: Initial Human Safety and Efficacy Outcomes. Urology 2018;114:184-7. [Crossref] [PubMed]
- Vidal-Jove J, Serres X, Vlaisavljevich E, et al. First-in-man histotripsy of hepatic tumors: the THERESA trial, a feasibility study. Int J Hyperthermia 2022;39:1115-23. [Crossref] [PubMed]
- Messas E, IJsselmuiden A, Goudot G, et al. Feasibility and Performance of Noninvasive Ultrasound Therapy in Patients With Severe Symptomatic Aortic Valve Stenosis: A First-in-Human Study. Circulation 2021;143:968-70. [Crossref] [PubMed]
- Alieva M, van Rheenen J, Broekman MLD. Potential impact of invasive surgical procedures on primary tumor growth and metastasis. Clin Exp Metastasis 2018;35:319-31. [Crossref] [PubMed]
- Deshmukh U, McAdow M, Black J, et al. Isolated port site recurrence of node-negative clinical stage IB1 cervical adenocarcinoma. Gynecol Oncol Rep 2017;20:54-7. [Crossref] [PubMed]
- Kim Y, Gelehrter SK, Fifer CG, et al. Non-invasive pulsed cavitational ultrasound for fetal tissue ablation: feasibility study in a fetal sheep model. Ultrasound Obstet Gynecol 2011;37:450-7. [Crossref] [PubMed]
- Baac HW, Lee T, Ok JG, et al. Dual-frequency focused ultrasound using optoacoustic and piezoelectric transmitters for single-pulsed free-field cavitation in water. Appl Phys Lett 2013;103:234103. [Crossref]
- Lin KW, Hall TL, Xu Z, et al. Histotripsy Lesion Formation Using an Ultrasound Imaging Probe Enabled by a Low-Frequency Pump Transducer. Ultrasound Med Biol 2015;41:2148-60. [Crossref] [PubMed]
- Kaymaz B, Mustafa W, Hall S, et al. Experimental and Computational Investigation of Clustering Behavior of Cyclodextrin-Perfluorocarbon Inclusion Complexes as Effective Histotripsy Agents. Mol Pharm 2022;19:2907-21. [Crossref] [PubMed]
- Vlaisavljevich E, Durmaz YY, Maxwell A, et al. Nanodroplet-mediated histotripsy for image-guided targeted ultrasound cell ablation. Theranostics 2013;3:851-64. [Crossref] [PubMed]
- Vlaisavljevich E, Aydin O, Yuksel Durmaz Y, et al. Effects of Ultrasound Frequency on Nanodroplet-Mediated Histotripsy. Ultrasound Med Biol 2015;41:2135-47. [Crossref] [PubMed]
- Pahk KJ. Control of the dynamics of a boiling vapour bubble using pressure-modulated high intensity focused ultrasound without the shock scattering effect: A first proof-of-concept study. Ultrason Sonochem 2021;77:105699. [Crossref] [PubMed]
- Liang H, Liang W, Zhao L, et al. Robotic Versus Video-assisted Lobectomy/Segmentectomy for Lung Cancer: A Meta-analysis. Ann Surg 2018;268:254-9. [Crossref] [PubMed]
- Sathianathen NJ, Kalapara A, Frydenberg M, et al. Robotic Assisted Radical Cystectomy vs Open Radical Cystectomy: Systematic Review and Meta-Analysis. J Urol 2019;201:715-20. [Crossref] [PubMed]
- Smolock AR, Cristescu MM, Vlaisavljevich E, et al. Robotically Assisted Sonic Therapy as a Noninvasive Nonthermal Ablation Modality: Proof of Concept in a Porcine Liver Model. Radiology 2018;287:485-93. [Crossref] [PubMed]
- Knott EA, Swietlik JF, Longo KC, et al. Robotically-Assisted Sonic Therapy for Renal Ablation in a Live Porcine Model: Initial Preclinical Results. J Vasc Interv Radiol 2019;30:1293-302. [Crossref] [PubMed]
- Thomas GPL, Khokhlova TD, Khokhlova VA. Partial Respiratory Motion Compensation for Abdominal Extracorporeal Boiling Histotripsy Treatments With a Robotic Arm. IEEE Trans Ultrason Ferroelectr Freq Control 2021;68:2861-70. [Crossref] [PubMed]
(English Language Editors: C.Mullens and J.Gray)