Step-by-step guide to robotic-guided minimally invasive transforaminal lumbar interbody fusion (MI-TLIF)
Introduction
In 1982, Harms and Rolinger published the first report of a new lumbar interbody fusion technique that utilized a transforaminal route to access the disc space (1). The “transforaminal lumbar interbody fusion” (TLIF) approach allowed interbody fusions to be less invasive, as the unilateral approach avoided retraction of the thecal sac and nerve root, consequently minimizing complication risk (2,3). These benefits of the TLIF, together with its capacity to produce mechanical stability comparable to a posterior lumbar interbody fusion (PLIF), led to a rise in the technique’s popularity (4). In 2003, Foley et al. introduced the minimally invasive TLIF (MI-TLIF), which utilized a microscope and tubular retractor systems (5). The minimally invasive approach offered advantages such as reduced hospital stay, blood loss, and narcotic use; however, it added increased radiation exposure and new technical challenges such as working through smaller incisions and unfamiliar tubular retraction systems (6-9). Advancements in navigation systems, surgical instruments, and surgical robots have considerably reduced the early difficulties, enabling the MI-TLIF to become the modern cornerstone of MI lumbar interbody fusions. Spine robots are among the latest innovations with a promising potential to refine and improve the TLIF technique (10). A challenge with adopting robotic-guided spine surgery is overcoming the initial learning curve associated with every new technique, and a key objective of this guide is to share technical pearls that enable surgeons to overcome this potential barrier sooner. Robotic-guided spine surgery enables surgeons to perform pedicle screw instrumentation and interbody cage placement with more accuracy and precision while minimizing surgeon fatigue and radiation exposure (11,12).
Overview of the TLIF
The TLIF technique allows for posterolateral fusion and anterior interbody fusion through a single posterior approach that is familiar to spine surgeons. Unilateral direct foraminal decompression is achieved through a facetectomy, and indirect bilateral foraminal decompression can be achieved by restoring sagittal height and alignment through interbody cage placement. Furthermore, direct central and lateral recess decompression may be performed through a hemilaminotomy or bilateral laminotomies. A minimally invasive approach allows each of these objectives to be accomplished in a manner that reduces collateral damage to surrounding tissues and improves patients’ recovery time.
Indications
This approach may be used in patients with lumbar spinal stenosis from lumbar disc herniation and/or ligamentum flavum hypertrophy. It is also useful for patients with foraminal stenosis from facet hypertrophy, disc degeneration & disc collapse, recurrent lumbar disc herniation, and lumbar degenerative scoliosis. Patients with spinal instability due to trauma, spondylolysis, or spondylolisthesis may also benefit from this technique.
Contraindications
Patients with osteoporosis or severe osteopenia may fracture end plates more easily during disc space preparation and interbody trial & graft placement. Patients with severe disc space collapse or disc space autofusion may be unable to obtain sufficient disc space height without fracturing the vertebral body end plates. Finally, severe obesity may prevent the use of a tubular retractor system.
Step-by-step surgical technique
Positioning
Patients are positioned in a prone “superman” position on a radiolucent Jackson table to facilitate both robotic-assisted screw placement, three-dimensional intraoperative navigation registration, and fluoroscopic imaging if necessary for percutaneous pedicle screw placement as a back-up plan in case registration errors occur the robot. The head is supported by a foam-cushion face mask and helmet with an adjustable mirrored platform (ProneView, Dupaco, Inc., Oceanside, CA, USA). The neck should be in neutral alignment. The shoulders are placed at no more than 90 degrees of abduction in a comfortable position without excessive external rotation to avoid brachial plexus injury. The arms supported by arm boards and placed palm down with elbows flexed to 90 degrees or slightly less. The axillae should be padded, and the medial aspects of the elbows should be padded and free from pressure to avoid injury to the ulnar nerve. The abdomen should be hanging free in order to prevent increased venal caval pressure that can increase bleeding during surgery due to congestion of the vertebral veins. The anterior superior iliac spine bony prominence should be supported by a positioning pad to avoid injury to the lateral femoral cutaneous nerve. Finally, the Jackson table is set up with flat boards distally to support the legs, maximize hip extension, and optimize physiologic lumbar lordosis.
Step 1: planning
After standard prepping and draping, we prefer placing navigation registration posts into both posterior superior iliac spines in a slight lateral-to-medial trajectory (Figure 1). The star navigation reference frame and 5-point boxed mask attaches to the larger caliber post to achieve intraoperative computed tomography (CT) imaging that is used for planning of pedicle screw, tubular retractor, and/or interbody cage starting points and trajectories. The image guidance infrared camera is at the caudal end of the table. The spine robot and associated viewing screen is at the cranial end of the table across from the lead surgeon. Our preference is the spine robot from Globus Medical (Audubon, PA, USA). The operating microscope is at the cranial end of the table on the same side as the lead surgeon. We prefer the Ziehm Vision mobile 3D C-arm (Ziehm Imaging, Orlando, FL, USA) for our intraoperative navigation platform. Note that pre-operative CT scans may also be used instead of intraoperative CT imaging, but we prefer to the latter because it provides real-time information of the lumbosacral spinal alignment on the Jackson table.
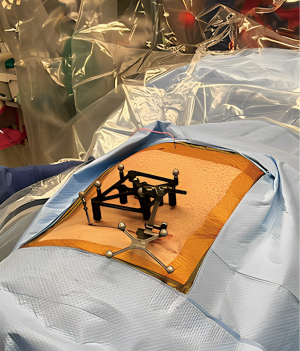
After intraoperative CT imaging is performed it is important to verify landmarks with the navigation probe to ensure 3D navigation registration accuracy. If surface landmarks are discordant with intraoperative navigation trajectories then another CT scan spin is recommended. Next, we plan trajectories for pedicle screws and the tubular retractor on the robotic viewing screen. Entry points for lumbar pedicle screws are generally chosen based on the intersection of the mid transverse process, lateral border of the superior articular facet, and mamillary process. A unilateral facetectomy is usually made on the side of worse radicular symptoms. It is important to consider a starting point that is slightly more lateral and a trajectory with a slight lateral angulation for pedicle screws on the side of the facetectomy to allow more space for tubular retractor docking and interbody cage placement (Figure 2). The trajectory of the tubular retractor should be angled medially and directly over the facet joint, with a line of site leading into the intervertebral disc space of interest.

Step 2: robotic-guided pedicle screw placement
Robotic-guided placement of pedicle screws according to planned starting points and trajectories is facilitated by a port connected to a robotic arm. This arm rotates and translates in space to reach the designated location for pedicle screw entry with the aid of a foot pedal. Once the port is in position and the proper screw trajectory is verified on the viewing screen, a transverse stab incision is made with a 10-blade inserted through the port. This cut should be made deep enough to cut the underlying lumbar fascia and provide a seamless path for navigated instruments and pedicle screw. A drill is used to create the starting point and should be advanced carefully into the vertebral body until a point just beyond the end of the pedicle. The drill should be started just off of the bone and then advanced in order to reduce the potential to skive off from the appropriate target. An undersized tap is then advanced, stopping at a point just distal to the drill stopping point. Finally, the pedicle screw is placed under power until it reaches the designated position.
Step 3: placement of tubular retractor
Robotic-guided placement of the tubular retractor begins with planning a starting point and trajectory directly over the facet joint that needs to be removed (Figure 3). The robot arm goes into the programmed position, which usually results in a navigated starting point approximately 2–3 cm lateral to the midline of the lumbar spine. We prefer making a 2 cm vertical incision at this site and traverse the fascia in order to access the facet joints. Serial dilators are used to expand the lumbar fascia and provide increasingly greater visualization. We prefer dilating up to a 2.5 cm diameter tubular retractor that is ultimately connected to the robotic arm. Appropriate tubular retractor placement directly over the facet joint is critical for performing a unilateral facetectomy.
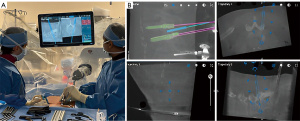
Step 4: unilateral facetectomy
The microscope is brought in after the tubular retractor is placed. Any creeping muscle should be removed with Bovie electrocautery so that the medial inferior articular process (IAP) and lateral superior articular process (SAP) can be identified. The vertically-oriented facet joint space should be in the center of the microscopic field. A burr is then used to resect the IAP and a portion of the medial lamina, but not enough to visualize the lateral dura. Next, the SAP is resected until the top of the caudal pedicle is reached. A 2 mm Kerrison rongeur can be used to remove excess bone just medial to the caudal pedicle, allowing for an ideal “runway” for cage placement. The soft tissue underneath the facet joints is removed carefully using a Kerrison rongeur in a caudal to cranial direction, being mindful that the exiting nerve root lies in the superolateral aspect of Camden’s Triangle. It’s important to remove sufficient soft tissue to expose the underlying dorsal aspect of interbody disc. This soft tissue can be coagulated using bipolar cautery in order to control muscular and venous bleeding. Often times this dissection will allow access to the disc space without ever exposing the exiting nerve root above.
Step 5: discectomy & disc preparation
Once clear visualization of the posterior disk is achieved, the diskectomy is started by first making a transverse annular incision with a 15 blade. A size 7 navigated shaver is then placed into the disc space and a lateral fluoroscopic X-ray is obtained in order to assess proper trajectory and angulation of the interbody trial, as well as confirm accuracy of navigation for the upcoming cage placement (Figure 4). The shaver is rotated inside the disc space to breakdown the intervertebral disc and a pituitary rongeur is used to remove disc material. This process is repeated as shaver sizes are increased to sizes 8 and 9 to gradually increase the interspace height while removing as much disk material as possible. Remaining superior and inferior cartilaginous endplate material are then carefully removed with curved rasps instruments (Figure 5). Meticulous technique must be used during end plate preparation in order to avoid violating the end plate surfaces, which can predispose to graft subsidence.
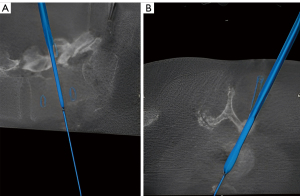
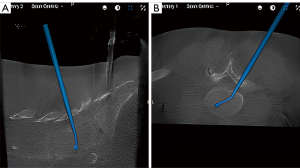
Step 6: interbody implant insertion
After thorough end plate preparation and diskectomy, the interbody cage is inserted into the interspace using robotic navigation guidance to overlay the projected ghost image. An articulating or bullet-style interbody graft may be used for this step. Our preference is to use an expandable, articulating interbody cage because we can better place the cage anteriorly at the periphery of the end plate to reduce subsidence and maximize lumbar lordosis. We refer to adjacent normal intervertebral disks as a guide for appropriate disk-height restoration and graft size. The interbody graft may be advanced in the interspace under navigation guidance and lateral fluoroscopic X-rays may be taken at any point to confirm trajectory and location (Figure 6). Once the graft is in the desired location and confirmed with fluoroscopic X-ray, it is expanded to increase interbody height. Interbody graft placement is followed by autograft insertion into the interspace through a funnel. At this point the tubular retractor system is removed in preparation for percutaneous rod placement.
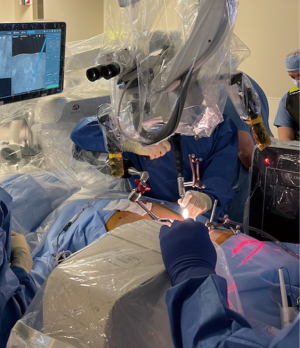
Step 7: percutaneous rod placement
Percutaneous rod placement begins with expanding the lumbar fascial cuts distally and proximally at the screw incision sites using a scalpel or Bovie cautery to allow for smoother rod passage. A pre-contoured lordotic rod is selected depending on the length of the construct. Rod passage begins by placing the rod into the proximal end of the construct using a rod-holder. As the rod is advanced the surgeon can evaluate whether the rod is successfully placed within a screw tulip by rotating the screw extensions. After the rod is felt to have passed through each pedicle screw tulip in the construct, a lateral fluoroscopic X-ray is obtained to assess rod length and location. If the rod is properly placed within the pedicle screw heads then a set screw is placed at the distal end of the construct first. Sequential intraoperative lateral X-rays are taken during this step to ensure the rod lays nicely into the pedicle screw tulip. The remaining set screws are placed using the same technique. The rod holder is removed after the set screws have been placed and if we are satisfied with the rod configuration and length. After the rod holder is removed, final tightening of the construct is performed and the screw extensions are removed. The wounds are irrigated with normal saline and closed in layers in standard fashion.
Please note that all procedures performed in this study were in accordance with the ethical standards of the institutional and/or national research committee(s) and with the Helsinki Declaration (as revised in 2013). Written informed consent was obtained from the patients for publication of this manuscript and accompanying images. A copy of the written consent is available for review by the editorial office of this journal.
Comments
Minimally invasive spinal surgery (MISS) relies heavily on intraoperative imaging due to poor visualization and palpation (13,14). Conventional fluoroscopy is associated is associated with pedicle screw misplacement and increased radiation exposure. As a result, there has been an evolution of newer imaging modalities over the last two decades, one of which is robotic navigation (11,15-17). Current-generation robotics offers integrated navigation capability and K-wireless placement of pedicle screws through a rigid robotic arm. It also allows for planning and placement of the interbody device (10,18).
Pedicle screw placement under fluoroscopic guidance is associated with high rates of misplacement (19-21). Two recent meta-analyses showed superior accuracy of pedicle screw placement with robotic assistance compared to traditional fluoroscopy (22,23). Proximal level violation by pedicle screws is supposed to increase the risk of adjacent segment disease (24-26). Robotics has been shown to decrease proximal facet joint violation rates (22,27). A 2021 study by Maalouly et al. showed a high degree of accuracy (98%) of pedicle screw placement with minimally invasive robot-assisted spinal fusion. The single surgeon in this study had a learning curve that improved with time, ultimately reaching a plateau at around 25 cases (28). Our group’s most recent study that is currently under review for publication details our experience with 1,050 screws using floor-mounted robotics and showed an overall pedicle screw accuracy of 96.4%, proximal level violation rate of 1.3%, and a single incident (0.1%) of screw-related complication that required screw revision.
Due to its heavy reliance on intraoperative imaging, MISS has been associated with an increase in intraoperative radiation usage, long-term exposure to which can lead to radiation-related health risks (7,29,30). Robotics has been associated with a reduction in radiation exposure as it decreases the requirement of intraoperative X-rays (31-33). The surgeon and surgical staff are not exposed to the initial (preoperative or intraoperative) CT scan. Shahi et al. demonstrated significant reduction in radiation exposure both to the surgeon and the patient with robotics compared to navigation, with no significant difference in the operative time (34).
In addition to the bone quality of the patient, screw size is an important contributor to the stability of the pedicle screw construct. An increase in pedicle screw diameter and length has been shown to increase the fixation strength (35). Good fixation in interbody fusion surgeries is important in order to provide a stable biomechanical environment for fusion to occur. A comparative study of robotics and navigation by Shafi et al. demonstrated that robotics allows for placement of the optimal pedicle screws with greater diameter and length compared to surgical navigation alone with similarly high accuracy (9).
Postoperative recovery is a common query that patients have when undergoing elective spine surgery. MISS is supposed to lead to shorter hospital stays and quicker return to activities due to less tissue damage and muscle dissection. Robotic MI-TLIF has been shown to lead to less intraoperative blood loss, shorter hospital length of stay, lower postoperative back pain and disability, and quicker recovery (36). A recent study demonstrated that patients undergoing MI-TLIF utilizing robotic assistance returned to driving, returned to work, and discontinued opioids by 18, 25, and 11 days, respectively, following surgery (37).
As with any new technology, robotic spine surgery is associated with an initial learning curve, which has been studied in terms of pedicle screw accuracy, operative time, and radiation dose. Khan et al. demonstrated a minimal learning curve with robotic spine surgery (38). Urakov et al. showed no significant difference in the speed of pedicle screw instrumentation based on the years of experience or dedication to spine surgery (39). A recent systematic review demonstrated a threshold of 20–30 cases and proposed an application of robotic spine surgery to residency curricula (40). In our training program, fellows rotate through MISS blocks for 3 months where they are exposed to robotic spine surgery, spinal navigation technology, and percutaneous pedicle screw placement using fluoroscopic- and navigation-guidance. We standardize surgeon training in robotic MI-TLIF by teaching fellows the 7 key technical steps highlighted in this guide.
Conclusions
Robotic-guided MI-TLIF is a safe procedure that allows for accurate and reliable pedicle screw placement, less collateral damage to the soft tissues of the low back, and decreased radiation exposure.
Acknowledgments
Funding: None.
Footnote
Conflicts of Interests: All authors have completed the ICMJE uniform disclosure form (available at https://atm.amegroups.com/article/view/10.21037/atm-22-3273/coif). SAQ serves as an unpaid Editorial Board Member of Annals of Translational Medicine from September 2021 to August 2023. SAQ also reports that he works for Globus Medical as paid presenter or speaker, consultant and receives royalties. The other authors have no conflicts of interest to declare.
Ethical Statement: The authors are accountable for all aspects of the work in ensuring that questions related to the accuracy or integrity of any part of the work are appropriately investigated and resolved. Furthermore, all procedures performed in this study were in accordance with the ethical standards of the institutional and/or national research committee(s) and with the Helsinki Declaration (as revised in 2013). Written informed consent was obtained from the patients for publication of this manuscript and accompanying images. A copy of the written consent is available for review by the editorial office of this journal.
Open Access Statement: This is an Open Access article distributed in accordance with the Creative Commons Attribution-NonCommercial-NoDerivs 4.0 International License (CC BY-NC-ND 4.0), which permits the non-commercial replication and distribution of the article with the strict proviso that no changes or edits are made and the original work is properly cited (including links to both the formal publication through the relevant DOI and the license). See: https://creativecommons.org/licenses/by-nc-nd/4.0/.
References
- Harms J, Rolinger H. A one-stager procedure in operative treatment of spondylolistheses: dorsal traction-reposition and anterior fusion (author's transl). Z Orthop Ihre Grenzgeb 1982;120:343-7. [Crossref] [PubMed]
- Schwender JD, Holly LT, Rouben DP, et al. Minimally invasive transforaminal lumbar interbody fusion (TLIF): technical feasibility and initial results. J Spinal Disord Tech 2005;18:S1-6. [Crossref] [PubMed]
- Perez-Cruet MJ, Hussain NS, White GZ, et al. Quality-of-life outcomes with minimally invasive transforaminal lumbar interbody fusion based on long-term analysis of 304 consecutive patients. Spine (Phila Pa 1976) 2014;39:E191-8. [Crossref] [PubMed]
- Humphreys SC, Hodges SD, Patwardhan AG, et al. Comparison of posterior and transforaminal approaches to lumbar interbody fusion. Spine (Phila Pa 1976) 2001;26:567-71. [Crossref] [PubMed]
- Foley KT, Holly LT, Schwender JD. Minimally invasive lumbar fusion. Spine (Phila Pa 1976) 2003;28:S26-35. [Crossref] [PubMed]
- Jain D, Ray WZ, Vaccaro AR. Advances in Techniques and Technology in Minimally Invasive Lumbar Interbody Spinal Fusion. JBJS Rev 2020;8:e0171. [Crossref] [PubMed]
- Kumar A, Merrill RK, Overley SC, et al. Radiation Exposure in Minimally Invasive Transforaminal Lumbar Interbody Fusion: The Effect of the Learning Curve. Int J Spine Surg 2019;13:39-45. [Crossref] [PubMed]
- Safaee MM, Oh T, Pekmezci M, et al. Radiation exposure with hybrid image-guidance-based minimally invasive transforaminal lumbar interbody fusion. J Clin Neurosci 2018;48:122-7. [Crossref] [PubMed]
- Shafi KA, Pompeu YA, Vaishnav AS, et al. Does robot-assisted navigation influence pedicle screw selection and accuracy in minimally invasive spine surgery? Neurosurg Focus 2022;52:E4. [Crossref] [PubMed]
- Alluri RK, Avrumova F, Sivaganesan A, et al. Overview of Robotic Technology in Spine Surgery. HSS J 2021;17:308-16. [Crossref] [PubMed]
- Vaishnav AS, Othman YA, Virk SS, et al. Current state of minimally invasive spine surgery. J Spine Surg 2019;5:S2-S10. [Crossref] [PubMed]
- Weiner JA, McCarthy MH, Swiatek P, et al. Narrative review of intraoperative image guidance for transforaminal lumbar interbody fusion. Ann Transl Med 2021;9:89. [Crossref] [PubMed]
- Virk S, Qureshi S. Navigation in minimally invasive spine surgery. J Spine Surg 2019;5:S25-30. [Crossref] [PubMed]
- Huang M, Tetreault TA, Vaishnav A, et al. The current state of navigation in robotic spine surgery. Ann Transl Med 2021;9:86. [Crossref] [PubMed]
- Vaishnav AS, Gang CH, Qureshi SA. Time-demand, Radiation Exposure and Outcomes of Minimally Invasive Spine Surgery With the Use of Skin-Anchored Intraoperative Navigation: The Effect of the Learning Curve. Clin Spine Surg 2022;35:E111-20. [Crossref] [PubMed]
- Bovonratwet P, Gu A, Chen AZ, et al. Computer-Assisted Navigation Is Associated With Decreased Rates of Hardware-Related Revision After Instrumented Posterior Lumbar Fusion. Global Spine J 2021; Epub ahead of print. [Crossref] [PubMed]
- Qureshi S, Lu Y, McAnany S, et al. Three-dimensional Intraoperative Imaging Modalities in Orthopaedic Surgery: A Narrative Review. J Am Acad Orthop Surg 2014;22:800-9. [Crossref] [PubMed]
- Avrumova F, Sivaganesan A, Alluri RK, et al. Workflow and Efficiency of Robotic-Assisted Navigation in Spine Surgery. HSS J 2021;17:302-7. [Crossref] [PubMed]
- Gautschi OP, Schatlo B, Schaller K, et al. Clinically relevant complications related to pedicle screw placement in thoracolumbar surgery and their management: a literature review of 35,630 pedicle screws. Neurosurg Focus 2011;31:E8. [Crossref] [PubMed]
- Baird EO, McAnany SJ, Overley S, et al. Accuracy of Percutaneous Pedicle Screw Placement: Does Training Level Matter? Clin Spine Surg 2017;30:E748-53. [Crossref] [PubMed]
- Korkmaz M, Sarıyılmaz K, Ozkunt O, et al. Quantitative comparison of a laterally misplaced pedicle screw with a re-directed screw. How much pull-out strength is lost? Acta Orthop Traumatol Turc 2018;52:459-63. [Crossref] [PubMed]
- Li HM, Zhang RJ, Shen CL. Accuracy of Pedicle Screw Placement and Clinical Outcomes of Robot-assisted Technique Versus Conventional Freehand Technique in Spine Surgery From Nine Randomized Controlled Trials: A Meta-analysis. Spine (Phila Pa 1976) 2020;45:E111-9. [Crossref] [PubMed]
- Fan Y, Du JP, Liu JJ, et al. Accuracy of pedicle screw placement comparing robot-assisted technology and the free-hand with fluoroscopy-guided method in spine surgery: An updated meta-analysis. Medicine (Baltimore) 2018;97:e10970. [Crossref] [PubMed]
- Sakaura H, Miwa T, Yamashita T, et al. Cortical bone trajectory screw fixation versus traditional pedicle screw fixation for 2-level posterior lumbar interbody fusion: comparison of surgical outcomes for 2-level degenerative lumbar spondylolisthesis. J Neurosurg Spine 2018;28:57-62. [Crossref] [PubMed]
- Cong T, Sivaganesan A, Mikhail CM, et al. Facet Violation With Percutaneous Pedicle Screw Placement: Impact of 3D Navigation and Facet Orientation. HSS J 2021;17:281-8. [Crossref] [PubMed]
- Bagheri SR, Alimohammadi E, Zamani Froushani A, et al. Adjacent segment disease after posterior lumbar instrumentation surgery for degenerative disease: Incidence and risk factors. J Orthop Surg (Hong Kong) 2019;27:2309499019842378. [Crossref] [PubMed]
- Zhou LP, Zhang RJ, Li HM, et al. Comparison of Cranial Facet Joint Violation Rate and Four Other Clinical Indexes Between Robot-assisted and Freehand Pedicle Screw Placement in Spine Surgery: A Meta-analysis. Spine (Phila Pa 1976) 2020;45:E1532-40. [Crossref] [PubMed]
- Maalouly J, Sarkar M, Choi J. Retrospective study assessing the learning curve and the accuracy of minimally invasive robot-assisted pedicle screw placement during the first 41 robot-assisted spinal fusion surgeries. Mini-invasive Surgery 2021;5:35. [Crossref]
- Srinivasan D, Than KD, Wang AC, et al. Radiation safety and spine surgery: systematic review of exposure limits and methods to minimize radiation exposure. World Neurosurg 2014;82:1337-43. [Crossref] [PubMed]
- Mroz TE, Abdullah KG, Steinmetz MP, et al. Radiation exposure to the surgeon during percutaneous pedicle screw placement. J Spinal Disord Tech 2011;24:264-7. [Crossref] [PubMed]
- Keric N, Doenitz C, Haj A, et al. Evaluation of robot-guided minimally invasive implantation of 2067 pedicle screws. Neurosurg Focus 2017;42:E11. [Crossref] [PubMed]
- Lieberman IH, Hardenbrook MA, Wang JC, et al. Assessment of pedicle screw placement accuracy, procedure time, and radiation exposure using a miniature robotic guidance system. J Spinal Disord Tech 2012;25:241-8. [Crossref] [PubMed]
- Kantelhardt SR, Martinez R, Baerwinkel S, et al. Perioperative course and accuracy of screw positioning in conventional, open robotic-guided and percutaneous robotic-guided, pedicle screw placement. Eur Spine J 2011;20:860-8. [Crossref] [PubMed]
- Shahi P, Vaishnav A, Araghi K, et al. Robotics Reduces Radiation Exposure in Minimally Invasive Lumbar Fusion Compared With Navigation. Spine (Phila Pa 1976) 2022;47:1279-86. [Crossref] [PubMed]
- Wittenberg RH, Lee KS, Shea M, et al. Effect of screw diameter, insertion technique, and bone cement augmentation of pedicular screw fixation strength. Clin Orthop Relat Res 1993;278-87. [Crossref] [PubMed]
- Cui GY, Han XG, Wei Y, et al. Robot-Assisted Minimally Invasive Transforaminal Lumbar Interbody Fusion in the Treatment of Lumbar Spondylolisthesis. Orthop Surg 2021;13:1960-8. [Crossref] [PubMed]
- Shinn D, Mok JK, Vaishnav AS, et al. Recovery Kinetics After Commonly Performed Minimally Invasive Spine Surgery Procedures. Spine (Phila Pa 1976) 2022;47:1489-96. [Crossref] [PubMed]
- Khan A, Meyers JE, Siasios I, et al. Next-Generation Robotic Spine Surgery: First Report on Feasibility, Safety, and Learning Curve. Oper Neurosurg (Hagerstown) 2019;17:61-9. [Crossref] [PubMed]
- Urakov TM, Chang KH, Burks SS, et al. Initial academic experience and learning curve with robotic spine instrumentation. Neurosurg Focus 2017;42:E4. [Crossref] [PubMed]
- Pennington Z, Judy BF, Zakaria HM, et al. Learning curves in robot-assisted spine surgery: a systematic review and proposal of application to residency curricula. Neurosurg Focus 2022;52:E3. [Crossref] [PubMed]