Peroxiredoxin 6 alleviates high glucose-induced inflammation and apoptosis in HK-2 cells by inhibiting TLR4/NF-κB signaling
Highlight box
Key findings
• PRDX6 protects HG-induced HK-2 cells against inflammation and apoptosis by inhibiting TLR4/NF-κB signaling.
What is known and what is new?
• PRDX6 is involved in the incidence and progression of inflammatory diseases. PRDX6 plays an important role in the pathogenesis of diabetes and PRDX6 elevation suppresses oxidative stress and ferroptosis to ease podocyte injury in DN.
• PRDX6 alleviates HG-induced inflammation and apoptosis of human renal tubular epithelial cells by inhibiting TLR4/NF-κB signaling.
What is the implication, and what should change now?
• This study identify a novel molecular mechanism underlying the PRDX6-mediated DN process and provides further evidence supporting the use of PRDX6-based targeted therapy in the treatment of DN.
Introduction
Diabetes is a complicated metabolic disease that is characterized by elevated levels of blood glucose and insulin resistance (1,2). The International Diabetes Federation estimated that there were >463 million cases of diabetes in 2019, and predicted that this figure would increase to 570 million by 2030 (3). Diabetic nephropathy (DN) is the most prevalent microvascular complication of diabetes, and it imposes a great burned on around 20–40% of individuals with diabetes (4,5). DN has also been confirmed to be a primary cause of end-stage renal disease responsible for diabetic mortality (6). DN has a complex and distinctive pathogenesis; however, it is well documented that glucose metabolism disturbance, abnormal lipid metabolism, the release of inflammatory cytokines, and oxidative stress are closely associated with the initiation and process of DN (7-9). Currently, the therapeutic strategies for DN include hypoglycemic, anti-hypertensive, and hypolipidemic therapies, but the treatment of DN remains challenging and troublesome, and no definitive therapies are currently available (10). A deeper understanding of the cause of DN is crucial to improving the efficacy of DN therapies.
Peroxiredoxins (PRDXs) are a highly-conserved selenium-independent peroxidase family of PRDXs that maintain oxidative homeostasis (11). Peroxiredoxin 6 (PRDX6), which belongs to the PRDX family, is a recently discovered PRDX ubiquitously expressed in the lung, brain, testis, kidney, and liver with glutathione peroxidase and phospholipase A2 activities (12,13). PRDX6 activities play critical roles in antioxidant defense and the maintenance of phospholipid metabolism (14). Extensive research has shown that PRDX6 is involved in the incidence and progression of inflammatory and neurodegenerative diseases; for example, the loss of PRDX6 alleviates acute and chronic dextran sodium sulfate-triggered colitis (15). PRDX6 has also been shown to be involved in cerebral ischemia/reperfusion inflammatory injury (16). Additionally, research has shown that PRDX6 plays a role in the pathogenesis of diabetes (17) and protects against type 1 diabetes (18). Notably, PRDX6 elevation suppresses oxidative stress and ferroptosis to ease podocyte injury in DN. However, further research needs to be conducted into the more specific effects of PRDX6 on DN and the potential regulatory mechanism related to mitochondrial dysfunction, oxidative stress, inflammation and apoptosis as well as the potential signaling pathways.
Toll-like receptor 4 (TLR4)/nuclear factor-kappa B (NF-κB) signaling is a classic inflammatory signaling pathway in which the activation of TLR4 binds to its ligand (such as, lipopolysaccharide and heat shock protein 60) to stimulate the release of the downstream transcription factor NF-κB, thereby eliciting an inflammatory response (19). There is extensive evidence that TLR4/NF-κB signaling is involved in sepsis-induced myocardial dysfunction, endometritis, and osteoarthritis via the modulation of inflammation (20-22). Further, TLR4/NF-κB signaling has been shown to drive inflammation in DN (23). Notably, PRDX6 exerts a radioprotective effect by interacting with TLR4/NF-κB signaling (24). However, it is unclear whether PRDX6 similarly controls biological functions in DN by modulating TLR4/NF-B signaling.
This study sought to determine the effects of PRDX6 on DN processes and the relationship between PRDX6 and TLR4/NF-B signaling. We present the following article in accordance with the MDAR reporting checklist (available at https://atm.amegroups.com/article/view/10.21037/atm-22-6063/rc).
Methods
Cell culture and treatment
The human renal tubular epithelial [human kidney 2 (HK-2)] cells were supplied by BeNa Culture Collection and maintained in Roswell Park Memorial Institute Medium-1640 (RPMI-1640; Beijing Solarbio Science and Technology Co., Ltd.) with 10% fetal bovine serum (Hunan Auragene Biotechnology Co., Ltd.) and 1% penicillin-streptomycin solution at 37 ℃ with 5% carbon dioxide. The cells were treated with 5.5 mM of glucose, 5 mM of glucose plus 24.5 mM of mannitol (MA), or 30 mM of glucose and divided into the Control, MA, and HG groups, respectively. Additionally, the TLR4 activator CRX-527 (0.5 ng/mL) (25) and the NF-κB activator phorbol 12-myristate 13-acetate (PMA; 1 µg/mL) (26) were used to treat the HK-2 cells.
Cell transfection
PRDX6 overexpression was achieved in the HK-2 cells by transfection with recombinant pcDNA3.1-PRDX6 plasmids (Oe-PRDX6, YouBio, Chongqing, China), and those transfected with pcDNA3.1 empty plasmids were used as the negative controls (Oe-NC). The procedure was conducted with Lipofectamine 2000 (Thermo Fisher) in accordance with the manufacturer’s instructions.
Reverse transcription-quantitative polymerase chain reaction (RT-qPCR)
Complementary deoxyribonucleic acid was produced using ReverTra-Plus™ kit (Toyobo Life Science) in accordance with the manufacturer’s instructions after ribonucleic acid (RNA) was isolated. The amplification was performed in the MX3000p PCR system (Agilent, Santa Clara, CA) using the SYBR Premix Ex Taq kit (Shanghai Biosteel Biotechnology) with β-actin for normalization. The 2-ΔΔCt method was used to analyze the messenger RNA levels (27).
Western blot analysis
Following HG stimulation, the HK-2 cells were lysed in lysis buffer (BestBio) and centrifuged (12,000 ×g, 4 ℃) for 20 min to acquire total proteins. The proteins were then transferred to polyvinylidene fluoride membranes after sodium dodecyl-sulfate polyacrylamide gel electrophoresis. The membranes were placed in 5% non-fat milk at room temperature for 1 h before being incubated with the corresponding primary antibodies overnight at 4 ℃, and the goat anti-rabbit horseradish peroxidase secondary antibody (1/2,000; Abcam) at room temperature for 2 h. The signals were developed using the enhanced chemiluminescence reagent (Advansta, Inc.), and the results were measured using ImageJ 1.46 (National Institutes of Health, USA).
JC-1 staining
Mitochondrial membrane potential was analyzed using a tetraethyl benzimidazolyl carbocyanine iodide (JC-1) detection kit (Nanjing KeyGen Biotech Co., Ltd.). Following HG exposure and plasmid transfection, the HK-2 cells were collected from the RPMI-1640 and washed with 5 µmol/L of JC-1 staining buffer at 37 ℃ for half an hour protected from light, and then rinsed in cold phosphate buffered solution twice. The results were photographed with a fluorescence microscope (Olympus Corporation; magnification ×200).
Determination of ATP and ROS contents
The HK-2 cells were lysed in lysis buffer (BestBio) and centrifuged (12,000 ×g, 4 ℃) for 20 min. Adenosine triphosphate (ATP) activity in the cell supernatant was detected using the ATP Bioluminescent Assay Kit (Promega Corporation) in accordance with the manufacturer’s instructions. To examine intracellular reactive oxygen species (ROS), 10 µΜ of dichloro-dihydro-fluorescein diacetate (Sigma-Aldrich) was used to treat the HK-2 cells at 37 ℃ for half an hour in the dark and the cells were then washed thrice with serum-starved RPMI-1640. The results were photographed with a fluorescence microscope (magnification ×200).
Cell Counting Kit-8 (CCK-8) assays
Cell viability was determined using the CCK-8 solution (Nanjing Keygen Biotechnology Co. Ltd.) in strict accordance with the manufacturer’s instructions. The HK-2 cells (5×103 cells/well) were incubated in 96-well plates overnight. CCK-8 solution (10 µL) was added to each well at 37 ℃ for an extra 2 h incubation. Then, the absorbance was measured using a 450-nm microplate reader (Tecan infinite).
Determination of intracellular SOD and MDA
The corresponding kits from Nanjing Jiancheng Bioengineering Co. Ltd. were employed to determine the levels of malonaldehyde (MDA; cat. no. A003-1-2) and superoxide dismutase (SOD; cat. no. A001-1-2) in the cell supernatant in accordance with manufacturer’s instruction. Absorbance was assessed using a microplate reader at 450 nm.
TUNEL
Cell apoptosis was appraised using a terminal-deoxynucleotidyl transferase mediated nick end labeling (TUNEL) Apoptosis kit (Nanjing Biobox Biotech Co., Ltd.). Briefly, 4% paraformaldehyde was used to fix the HK-2 cells, and 0.1% Triton X-100 was then added for permeabilization. Subsequently, the cells were cultivated with TUNEL reaction reagent for 1 h and the nuclei were labeled with 10 mg/mL 4',6-diamidino-2-phenylindole for 10 min. Finally, 5 random fields of view were selected to capture images using a fluorescence microscope (magnification ×200) and analyzed using ImageJ 1.46.
Statistical analysis
All the experiments were repeated 3 times. All the data were reported as the mean ± standard deviation and analyzed with GraphPad Prism 8.0 software. A 1-way analysis of variance followed by Tukey’s post-hoc test was used to compare differences among multiple groups. A P value <0.05 was considered statistically significant.
Results
PRDX6 levels decrease upon HG exposure
To show that the DN cellular model had been successfully established, the mitochondrial membrane potential, the content of ATP produced from the mitochondria, and activities of oxidative stress factors were measured. As Figure 1A shows, the experimental results of JC-1 staining showed that relative to the control group, the cells in the HG group emitted green fluorescence with little red fluorescence, suggesting that mitochondrial membrane potential was reduced. ROS activity increased in the HK-2 cells upon HG stimulation (Figure 1B). In addition, HG treatment resulted in a decrease in ATP production (Figure 1C). Additionally, the oxidative stress marker SOD level showed a downward trend while the MDA level was increased in the HG group compared to the control group (Figure 1D,1E). Notably, RT-qPCR and western blot revealed that the level of PRDX6 of the HK-2 cells in the HG group was significantly reduced compared to that of the control group (Figure 1F,1G). Thus, HG stimulation decreased mitochondrial membrane potential and PRDX6 expression but exacerbated oxidative stress in the HK-2 cells, which suggests that PRDX6 might be involved in the DN process.
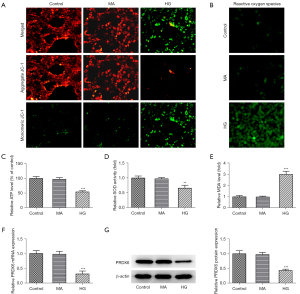
Effects of PRDX6 on oxidative stress during HG exposure
To further examine the effects of PRDX6 on the biological activities of the HG-stimulated HK-2 cells, PRDX6 overexpression was induced. The RT-qPCR and western blot experimental results indicated that PRDX6 expression was significantly increased after the transfection of the Oe-PRDX6 plasmids (Figure 2A,2B). Subsequently, JC-1 staining showed that PRDX6 overexpression alleviated the decrease in the mitochondrial membrane potential caused by HG (Figure 2C). Subsequently, in the Oe-PRDX6 + HG group, it was discovered that the increased ROS content in the HG-stimulated HK-2 cells was suppressed (Figure 2D). Additionally, the HG-suppressed ATP level significantly increased after PRDX6 was overexpressed (Figure 2E). As anticipated, the decreased SOD level and the increased MDA level in the HG-treated HK-2 cells were both reversed on PRDX6 overexpression (Figure 2F,2G). Additionally, HG stimulation decreased the mito-cytochrome c protein level but increased the cyto-cytochrome c protein level, while PRDX6 increased the mito-Cytochrome c protein level and decreased the cyto-cytochrome c protein level (Figure 2H), suggesting that PRDX6 impeded the release of cytochrome c from the mitochondria. Thus, PRDX6 overexpression mitigated mitochondrial dysfunction and oxidative stress in the HG-exposed HK-2 cells.
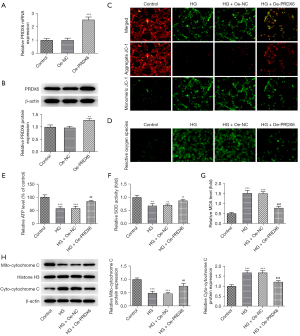
Effects of PRDX6 on apoptosis and the inflammatory response during HG exposure
Further, the CCK-8 assays showed that the suppressed viability of the HG-exposed HK-2 cells was increased when PRDX6 was upregulated (Figure 3A). Conversely, as Figure 3B shows, the TUNEL staining results revealed that HG-induced apoptosis in the HK-2 cells was obstructed by PRDX6 elevation (Figure 3B,3C). The western blot results showed that PRDX6 increased downregulated B-cell lymphoma 2 (Bcl-2) expression and decreased upregulated Bax expression in the HK-2 cells following HG treatment (Figure 3D). An examination of the inflammatory factors by western blot revealed that tumor necrosis factor alpha (TNF-α), interleukin-6 (IL-6), and monocyte chemoattractant protein-1 (MCP-11) protein levels decreased due to PRDX6 elevation (Figure 3E). Thus, PRDX6 exerted inhibitory effects on the apoptosis and inflammation of the HG-treated HK-2 cells.
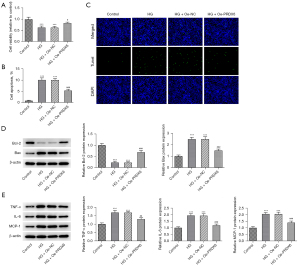
PRDX6 ameliorates apoptosis and the inflammatory response by TLR4/NF-κB signaling
More interestingly, the Western blot results indicated that the level of TLR4/NF-κB signaling-related factors [i.e., TLR4, phosphor (p)-p65, and p-inhibitor of kappa Bα (IκBα)] was more increased in the HG group than the control group. Their expression levels in the HG + Oe-PRDX6 group were significantly reduced compared to those in the HG + Oe-NC group (Figure 4). To further examine the roles of TLR4/NF-κB signaling, the TLR4 activator CRX-527 or the NF-κB activator PMA were used to treat the HK-2 cells. Based on the CCK-8 assay results, the protective role of PRDX6 in HG-induced HK-2 cell viability injury was offset by the administration of CRX-527 or PMA (Figure 5A). Conversely, the decreased apoptosis of the HG-stimulated HK-2 cells induced by PRDX6 upregulation was again promoted by CRX-527 or PMA (Figure 5B,5C). Similarly, the increase in Bcl-2 expression and decrease in Bax expression in the HG-induced HK-2 cells caused by PRDX6 were both counteracted by the addition of CRX-527 or PMA (Figure 5D). Thus, CRX-527 or PMA partially restored TNF-α, IL-6, and MCP-1 expression in HG-induced HK-2 cells with PRDX6 overexpression (Figure 5E). Collectively, PRDX6 inhibited TLR4/NF-κB signaling to ease apoptosis and inflammation in the HG-exposed HK-2 cells.
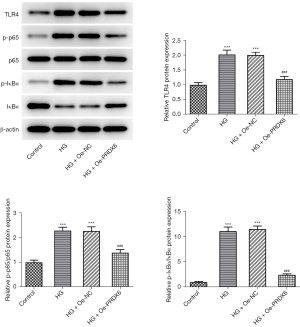
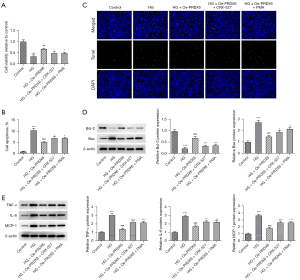
Discussion
DN refers to a series of pathological and physiological changes in diabetes resulting from the mutual effects of multitudinous factors, including environmental, genetic, epigenetic factors, oxidative stress, inflammation, and mitochondrial dysfunction (28,29). Long-term exposure to HG stress is a driving factor triggering DN, which may stimulate oxidative stress by generating massive ROS through enzymatic or non-enzymatic reactions (30). Additionally, the excessive production and accumulation of ROS may lead to mitochondrial dysfunction, which may in turn further aggravate ROS production (31). Thus, mitochondrial function and oxidative stress under HG conditions were used to successfully establish an in vitro DN cell model. In this experiment, the HK-2 cells were stimulated under an HG environment. As anticipated, the results showed that HG treatment significantly damaged mitochondrial membrane potential and exacerbated ROS generation. ATP is thought to be a source of chemical energy in organisms, and ATP is frequently supplied by the mitochondria (32). The experimental data in this study also confirmed that HG exposure resulted in ATP depletion. MDA forms as a decomposition product of lipid peroxidation where ROS attacks free unsaturated fatty acids (33). SOD is a vital antioxidant for ROS elimination, which reflects antioxidant capacity (34). The results of this study showed that the SOD level decreased while the MDA level increased in the HK-2 cells under HG conditions. These results imply that HG treatment contributes to mitochondrial dysfunction and oxidative stress.
It was recently conjectured that the activity regulation of PRDX6 is closely related to the occurrence of a number of human diseases (11). In the PRDX family, PRDX6 is capable of eliminating ROS to defend against oxidative stress-dependent on glutathione (GSH) peroxidase activity (35). Our experimental results first revealed that PRDX6 expression was significantly reduced in the HG-exposed HK-2 cells. The functional experiments showed that in the HG-exposed HK-2 cells, PRDX6 elevation significantly improved mitochondrial membrane potential, suppressed ROS generation, promoted ATP production, and halted oxidative stress. These findings highlighted that PRDX6 alleviated mitochondrial dysfunction and oxidative stress injury in the HK-2 cells exposed to HG, which was in line with the findings of Zhang et al. (36) that Sp1-activated PRDX6 relieves oxidative stress in podocytes under HG stimulation in DN.
Oxidative stress is a leading cause of apoptosis (37). In the process of DN, oxidative stress is implicated in the inflammatory response (38). The release of proinflammatory cytokines is also believed to deteriorate renal function (39). The present study showed that PRDX6 hindered HG-triggered viability injury and apoptosis. Similarly, the lessened anti-apoptotic Bcl-2 expression and the augmented pro-apoptotic Bax expression caused by the HG treatment were both reversed by the overexpression of PRDX6. As a dominant inflammatory factor, TNF-α not only activates NF-κB signaling but also stimulates cytotoxicity to induce renal injury in DN. IL-6 and MCP-1 are also prototypical proinflammatory chemokines (40). Similarly, PRDX6 was discovered to decrease the upregulated expression of TNF-α, IL-6, and MCP-1 in the HK-2 cells in a HG environment.
TLR4 is a major receptor in the congenital immune and inflammatory responses (41). NF-κB is the most significant intracellular nuclear transcription factor and is responsible for modulating multiple inflammatory genes and proteins (42). The binding of TLR4 to its ligands pushes the translocation of NF-κB from the cytoplasm into the nucleus, and NF-κB signaling participates in the inflammatory response (19). It’s worth noting that TLR4/NF-κB signaling has a stimulative effect on inflammation in DN (23). Notably, PRDX6 has been shown to exert a radioprotective effect by interacting with TLR4/NF-κB signaling (24). This study showed that HG exposure strengthened the expression of TLR4/NF-κB signaling-associated proteins TLR4, p-p65, and p-IκBα. The subsequent administration of TLR4 activator CRX-527 or NF-κB activator PMA partially counteracted the effects of PRDX6 on the viability, apoptosis, and inflammation of the HG-exposed HK-2 cells. The present study provided experimental support for the use of PRDX6 for the targeted therapy of DN, which laid foundation for the application of PRDX6 in DN treatment.
Conclusions
To summarize, PRDX6 inhibited apoptosis and inflammation in the HG-induced renal tubular epithelial cells in DN via TLR4/NF-B signaling inactivation. Thus, this study identified a novel molecular mechanism underlying the PRDX6-mediated DN process and provides further evidence supporting the use of PRDX6-based targeted therapy in the treatment of DN. However, only in vitro experiments were performed in this paper, and the lack of in vivo animal experiments is a limitation of this study, which will be conducted to support the conclusion of this paper our future research.
Acknowledgments
Funding: None.
Footnote
Reporting Checklist: The authors have completed the MDAR reporting checklist. Available at https://atm.amegroups.com/article/view/10.21037/atm-22-6063/rc
Data Sharing Statement: Available at https://atm.amegroups.com/article/view/10.21037/atm-22-6063/dss
Conflicts of Interest: All authors have completed the ICMJE uniform disclosure form (available at https://atm.amegroups.com/article/view/10.21037/atm-22-6063/coif). The authors have no conflicts of interest to declare.
Ethical Statement: The authors are accountable for all aspects of the work in ensuring that questions related to the accuracy or integrity of any part of the work are appropriately investigated and resolved.
Open Access Statement: This is an Open Access article distributed in accordance with the Creative Commons Attribution-NonCommercial-NoDerivs 4.0 International License (CC BY-NC-ND 4.0), which permits the non-commercial replication and distribution of the article with the strict proviso that no changes or edits are made and the original work is properly cited (including links to both the formal publication through the relevant DOI and the license). See: https://creativecommons.org/licenses/by-nc-nd/4.0/.
References
- Blair M. Diabetes Mellitus Review. Urol Nurs 2016;36:27-36. [Crossref] [PubMed]
- Harreiter J, Roden M. Diabetes mellitus-Definition, classification, diagnosis, screening and prevention (Update 2019). Wien Klin Wochenschr 2019;131:6-15. [Crossref] [PubMed]
- Saeedi P, Petersohn I, Salpea P, et al. Global and regional diabetes prevalence estimates for 2019 and projections for 2030 and 2045: Results from the International Diabetes Federation Diabetes Atlas, 9(th) edition. Diabetes Res Clin Pract 2019;157:107843. [Crossref] [PubMed]
- Schernthaner G, Schernthaner GH. Diabetic nephropathy: new approaches for improving glycemic control and reducing risk. J Nephrol 2013;26:975-85. [Crossref] [PubMed]
- Sagoo MK, Gnudi L. Diabetic Nephropathy: An Overview. Methods Mol Biol 2020;2067:3-7. [Crossref] [PubMed]
- Warren AM, Knudsen ST, Cooper ME. Diabetic nephropathy: an insight into molecular mechanisms and emerging therapies. Expert Opin Ther Targets 2019;23:579-91. [Crossref] [PubMed]
- Samsu N. Diabetic Nephropathy: Challenges in Pathogenesis, Diagnosis, and Treatment. Biomed Res Int 2021;2021:1497449. [Crossref] [PubMed]
- Stadler K, Goldberg IJ, Susztak K. The evolving understanding of the contribution of lipid metabolism to diabetic kidney disease. Curr Diab Rep 2015;15:40. [Crossref] [PubMed]
- Arora MK, Singh UK. Molecular mechanisms in the pathogenesis of diabetic nephropathy: an update. Vascul Pharmacol 2013;58:259-71. [Crossref] [PubMed]
- Lu Y, Liu D, Feng Q, et al. Diabetic Nephropathy: Perspective on Extracellular Vesicles. Front Immunol 2020;11:943. [Crossref] [PubMed]
- Lee YJ. Knockout Mouse Models for Peroxiredoxins. Antioxidants (Basel) 2020.
- Fisher AB. Peroxiredoxin 6: a bifunctional enzyme with glutathione peroxidase and phospholipase A2 activities. Antioxid Redox Signal 2011;15:831-44. [Crossref] [PubMed]
- Yamada Y, Limmon GV, Zheng D, et al. Major shifts in the spatio-temporal distribution of lung antioxidant enzymes during influenza pneumonia. PLoS One 2012;7:e31494. [Crossref] [PubMed]
- Fisher AB. Peroxiredoxin 6 in the repair of peroxidized cell membranes and cell signaling. Arch Biochem Biophys 2017;617:68-83. [Crossref] [PubMed]
- Melhem H, Spalinger MR, Cosin-Roger J, et al. Prdx6 Deficiency Ameliorates DSS Colitis: Relevance of Compensatory Antioxidant Mechanisms. J Crohns Colitis 2017;11:871-84. [Crossref] [PubMed]
- Shanshan Y, Beibei J, Li T, et al. Phospholipase A2 of Peroxiredoxin 6 Plays a Critical Role in Cerebral Ischemia/Reperfusion Inflammatory Injury. Front Cell Neurosci 2017;11:99. [Crossref] [PubMed]
- Pacifici F, Arriga R, Sorice GP, et al. Peroxiredoxin 6, a novel player in the pathogenesis of diabetes. Diabetes 2014;63:3210-20. [Crossref] [PubMed]
- Novoselova EG, Glushkova OV, Lunin SM, et al. Peroxiredoxin 6 Attenuates Alloxan-Induced Type 1 Diabetes Mellitus in Mice and Cytokine-Induced Cytotoxicity in RIN-m5F Beta Cells. J Diabetes Res 2020;2020:7523892. [Crossref] [PubMed]
- Maher DP, Walia D, Heller NM. Morphine decreases the function of primary human natural killer cells by both TLR4 and opioid receptor signaling. Brain Behav Immun 2020;83:298-302. [Crossref] [PubMed]
- Luo M, Yan D, Sun Q, et al. Ginsenoside Rg1 attenuates cardiomyocyte apoptosis and inflammation via the TLR4/NF-kB/NLRP3 pathway. J Cell Biochem 2020;121:2994-3004. [Crossref] [PubMed]
- Zhang H, Wu ZM, Yang YP, et al. Catalpol ameliorates LPS-induced endometritis by inhibiting inflammation and TLR4/NF-κB signaling. J Zhejiang Univ Sci B 2019;20:816-27. [Crossref] [PubMed]
- Ding Y, Wang L, Zhao Q, et al. MicroRNA-93 inhibits chondrocyte apoptosis and inflammation in osteoarthritis by targeting the TLR4/NF-κB signaling pathway. Int J Mol Med 2019;43:779-90. [PubMed]
- Mudaliar H, Pollock C, Panchapakesan U. Role of Toll-like receptors in diabetic nephropathy. Clin Sci (Lond) 2014;126:685-94. [Crossref] [PubMed]
- Sharapov MG, Glushkova OV, Parfenyuk SB, et al. The role of TLR4/NF-κB signaling in the radioprotective effects of exogenous Prdx6. Arch Biochem Biophys 2021;702:108830. [Crossref] [PubMed]
- Legat A, Thomas S, Hermand P, et al. CD14-independent responses induced by a synthetic lipid A mimetic. Eur J Immunol 2010;40:797-802. [Crossref] [PubMed]
- Duan Y, Luo Q, Wang Y, et al. Adipose mesenchymal stem cell-derived extracellular vesicles containing microRNA-26a-5p target TLR4 and protect against diabetic nephropathy. J Biol Chem 2020;295:12868-84. [Crossref] [PubMed]
- Livak KJ, Schmittgen TD. Analysis of relative gene expression data using real-time quantitative PCR and the 2(-Delta Delta C(T)) Method. Methods 2001;25:402-8. [Crossref] [PubMed]
- Lin TA, Wu VC, Wang CY. Autophagy in Chronic Kidney Diseases. Cells 2019;8:61. [Crossref] [PubMed]
- Papadopoulou-Marketou N, Paschou SA, Marketos N, et al. Diabetic nephropathy in type 1 diabetes. Minerva Med 2018;109:218-28. [Crossref] [PubMed]
- Aluksanasuwan S, Plumworasawat S, Malaitad T, et al. High glucose induces phosphorylation and oxidation of mitochondrial proteins in renal tubular cells: A proteomics approach. Sci Rep 2020;10:5843. [Crossref] [PubMed]
- Wei PZ, Szeto CC. Mitochondrial dysfunction in diabetic kidney disease. Clin Chim Acta 2019;496:108-16. [Crossref] [PubMed]
- Annesley SJ, Fisher PR. Mitochondria in Health and Disease. Cells 2019;8:680. [Crossref] [PubMed]
- Cooley JC, Lunte CE. Detection of malondialdehyde in vivo using microdialysis sampling with CE-fluorescence. Electrophoresis 2011;32:2994-9. [Crossref] [PubMed]
- Wang Y, Branicky R, Noë A, et al. Superoxide dismutases: Dual roles in controlling ROS damage and regulating ROS signaling. J Cell Biol 2018;217:1915-28. [Crossref] [PubMed]
- Manevich Y, Reddy KS, Shuvaeva T, et al. Structure and phospholipase function of peroxiredoxin 6: identification of the catalytic triad and its role in phospholipid substrate binding. J Lipid Res 2007;48:2306-18. [Crossref] [PubMed]
- Zhang Q, Hu Y, Hu JE, et al. Sp1-mediated upregulation of Prdx6 expression prevents podocyte injury in diabetic nephropathy via mitigation of oxidative stress and ferroptosis. Life Sci 2021;278:119529. [Crossref] [PubMed]
- Ogura S, Shimosawa T. Oxidative stress and organ damages. Curr Hypertens Rep 2014;16:452. [Crossref] [PubMed]
- Turkmen K. Inflammation, oxidative stress, apoptosis, and autophagy in diabetes mellitus and diabetic kidney disease: the Four Horsemen of the Apocalypse. Int Urol Nephrol 2017;49:837-44. [Crossref] [PubMed]
- Betjes MG. Immune cell dysfunction and inflammation in end-stage renal disease. Nat Rev Nephrol 2013;9:255-65. [Crossref] [PubMed]
- Panagi L, Poole L, Hackett RA, et al. Happiness and Inflammatory Responses to Acute Stress in People With Type 2 Diabetes. Ann Behav Med 2019;53:309-20. [Crossref] [PubMed]
- Flier S, Concepcion AN, Versteeg D, et al. Monocyte hyporesponsiveness and Toll-like receptor expression profiles in coronary artery bypass grafting and its clinical implications for postoperative inflammatory response and pneumonia: An observational cohort study. Eur J Anaesthesiol 2015;32:177-88. [Crossref] [PubMed]
- Mitchell JP, Carmody RJ. NF-κB and the Transcriptional Control of Inflammation. Int Rev Cell Mol Biol 2018;335:41-84. [Crossref] [PubMed]
(English Language Editor: L. Huleatt)