Evaluating the relationship between induced aniseikonia and distance stereopsis
Highlight box
Key findings
• The stereoacuity decreased with increasing aniseikonia. Overall aniseikonia affected stereopsis more seriously than meridional aniseikonia. As the angle changed from 180º to 90º, the trend of stereoacuity was improved gradually in the contour-based pattern and deteriorated gradually in the random-dot-based pattern.
What is known and what is new?
• Stereoacuity was decreased by optically induced anisometropia and aniseikonia.
• The method to create aniseikonia is innovative. Aniseikonia in oblique meridians was added in this study. The difference between contour-based and random-dot-based patterns was also elucidated.
What is the implication, and what should change now?
• The induced additional disparity with the traditional method to induce aniseikonia may interfere with the result of stereoacuity. A new method designed by us may change the situation but still have limitations. A better method should be explored in the future.
Introduction
Aniseikonia is a condition in which the apparent sizes of the images seen by the two eyes are unequal (1). The etiology of aniseikonia may include optical, retinal, or cortical factors (1,2). Stereopsis refers to the function of precisely judging distance with the help of horizontal retinal parallax and may be affected by factors related to binocular vision, such as amblyopia, strabismus, uncorrected refractive errors, unilateral ocular pathology, etc. (3). The relationship between aniseikonia and stereopsis has been discussed for several decades.
The most common reason for aniseikonia is the correction of anisometropia. Lenses with different spherical diopters lead to different magnifications of the optical systems of both eyes. Correcting astigmatism with a cylinder could result in meridional aniseikonia (4). Lovasik and Szynkiw (3) utilized afocal magnifiers to induce aniseikonia ranging from 1.2% to 32.3%, and measured stereopsis using Titmus and Randot Tests. All participants in their study demonstrated stereopsis of up to 13.3% aniseikonia in both tests. In the field of aniseikonia between 13.3% and 22.3%, an average of 82% showed stereopsis. Meanwhile, for aniseikonia above 22.3%, 34% showed stereopsis. Hess et al. (5) utilized an Apple iPod (model A1367; Apple, Inc., Cupertiono, CA, USA) and red-green anaglyph glasses to carry out aniseikonia and stereotest. They found that every 1% difference in image size between the eyes resulted in a 33% loss of stereopsis. Atchison et al. (6) confirmed that the stereoacuity was decreased by optically induced anisometropia and aniseikonia, with the reduction in stereoacuity increasing as the interocular difference increased. They found that the stereopsis threshold at baseline averaged 1.37 log sec arc, and increased to 1.93 log sec arc with 12% overall aniseikonia. The threshold of stereopsis for overall aniseikonia was significantly higher than that of meridional aniseikonia. No significant difference was observed between the meridional aniseikonia of 180º, 45º, and 90º. The meridional aniseikonia had 64% the effect of overall aniseikonia.
With the development of retinal surgery, research on aniseikonia caused by retinal factors has been carried out in recent years (7-13). In contrast to the aniseikonia caused by optical factors, which are often symmetrical, aniseikonia caused by retinal factors tends to be asymmetrical. Okamoto et al. (7) reported that unilateral idiopathic epiretinal membrane (ERM) surgery significantly improved stereopsis but not aniseikonia. The postoperative Titmus and The Netherlands Optical Society (TNO) test scores showed a marked correlation with preoperative aniseikonia. Khanna et al. (14) conducted a 2-year observation of the effect of unilateral idiopathic ERM removal on monocular and binocular visual functions. Notable progress was observed between stereoacuity evaluated using the TNO stereotest preoperatively and at 2 years postoperatively. Vertical and average aniseikonia improved significantly, whereas horizontal aniseikonia did not.
Although the relationship between aniseikonia and stereopsis has been studied over an extended period, research findings on the interference of aniseikonia with stereopsis are not consistent (15-18). Different stereopsis test materials, contour-based symbols or random-dot-based symbols (19,20), and test conditions of real circumstances or by dissociating the two eyes (21-26), etc. may affect test results. Moreover, owing to the method adopted to induce aniseikonia, it is difficult to evaluate this perfectly. Size lenses were the most commonly used method to induce aniseikonia by enlarging the image in front of one eye. Nevertheless, enlarging the entire test image may introduce additional disparity, especially when the smallest test units inside the test material are arranged horizontally. Enlarging itself may make the left part of the image extend more to the left, and the right part extends more to the right, and the same is true for the up and down parts.
Evaluation of the stereopsis test is based on the horizontal disparity, so the position in the vertical direction can be ignored. If the image in front of the left eye is enlarged while keeping the image in front of the right eye unchanged, the left part of the image in front of the left eye would extend to the left and an uncrossed disparity would be introduced. Consequently, the left part of the image appears to move backward, and the farther away from the center, the more obvious this effect is. On the contrary, the right part of the image would extend to the right, introducing crossed disparity, and the right part of the image would appear to move forward. In this situation, the image would appear to rotate clockwise along the vertical axis. Although the change in the perpendicular direction of the image may make the situation more complicated, the induced disparity by aniseikonia in the horizontal axis cannot be avoided, especially when using a larger magnification. If the test symbols are arranged horizontally, the induced disparity of aniseikonia would overlap with the setting disparity of the test, which may interfere with the subject’s judgment, especially when using a relatively large magnification. We assumed that this might be a reason for the poor consistency in previous studies.
Previously, we conducted a research to confirm the relationship between induced aniseikonia and near stereopsis and modified the arrangement of test targets to a vertical alignment (27). Although induced disparity by aniseikonia could not be avoided totally, the disparity was transformed into a systemic error, which meant that the position changing of all test symbols was the same; hence, the interference to the judgment of the stereo target could be avoided.
Distance and near stereopsis reflect two aspects of stereopsis. Previous research has shown that these are consistent in the normal population, while others found they were quite different (28,29). The difference between distance and near stereopsis was significant when suffering from diseases (30,31). There is also little literature referring to stereopsis affected by meridional aniseikonia. We aim to evaluate distance stereopsis with induced aniseikonia based on near stereopsis research (27), and aniseikonia in oblique meridians was added in this study. The difference between contour-based and random-dot-based patterns was also elucidated. We present the following article in accordance with the MDAR reporting checklist (available at https://atm.amegroups.com/article/view/10.21037/atm-22-5575/rc).
Methods
Participants
Thirty medical undergraduates and postgraduates (including 17 females and 13 males, aged 20 to 27) were recruited. The visual acuity of each eye was no worse than 0 logMAR, and the stereoacuity of each eye (tested using the Fly Stereo Acuity Test [Vision Assessment Corporation, Elk Grove Village, IL, United States]) was better than 32”. All participants gave their written informed consent before taking part in the study. The study was conducted in accordance with the Declaration of Helsinki (as revised in 2013) and was approved by the ethics committee of the Second Hospital of Jilin University (No. 2020-110).
Test system
Equipment
A three-dimensional (3D) laptop [ASUS G750Y47JX, 17.3" 16:9 full HD 3D (1,920×1,080 120 Hz), ASUSTEK Computer Inc., Taiwan] equipped with NVidia 3D glasses (Expressway Santa Clara, CA, USA) was utilized as the test system, which we had used previously (32,33). The dot pitch of the screen was 0.199 mm, which signifies that a one-pixel distance would create 10 seconds of arc (arcsec, ”) at a checking distance of 4.1 m (Figure 1).
Test symbol
The test symbol was designed according to our previous study on the relationship between aniseikonia and stereopsis at a near distance (27). A contour-based symbol imitated the quantitative test section of the Fly Stereo Acuity Test, whereas the arrangement pattern was adjusted. The four circles in each test unit of the Fly Stereo Acuity Test were arranged evenly in the up, down, left, and right directions, respectively. In our analysis, four test circles were arranged vertically, with reference circles placed on both sides of them (Figure 2). This arrangement aimed to eliminate any additional aniseikonia-induced disparity in the horizontal direction. The random-dot-based pattern was four vertically arranged squares with a stereo circle hidden in one of them randomly (Figure 3).
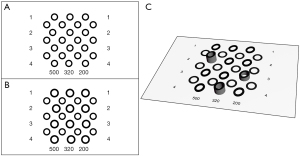
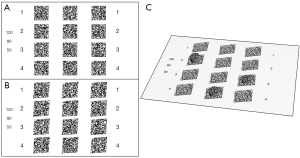
Test page
The disparity setting was the same in both the contour-based and random-dot-based patterns. There were a total of six magnifications of induced aniseikonia, including 5%, 10%, 15%, 20%, 25%, and 30%. The aniseikonia was induced overall or in one of the meridians. The induced meridional aniseikonia included 180°, 30°, 45°, 60°, and 90°. Therefore, taking the combination of magnification and meridian, there were a total of 36 sets of induced aniseikonia. Each set of the test contained three test pages with nine disparity settings: 500” (2.7 log arcsec), 320” (2.5 log arcsec), and 200” (2.3 log arcsec) on test page one; 120” (2.1 log arcsec), 80” (1.9 log arcsec), and 50” (1.7 log arcsec) on test page two; 30” (1.5 log arcsec), 20” (1.3 log arcsec), and 10” (1 log arcsec) on test page three.
Test procedure
The test sequences of both the contour-based and random-dot-based patterns were random, as was the test sequence of each meridian or overall. After determining the pattern and meridian, the test sequence of magnification was sequentially fixed from 30% to 5%. The participants were asked to point out which circle stood out of the plane in the contour-based test or which square contained a protruding circle in the random-dot test. The test was started at 500” and moved backward to 10”. The last correct choice was recorded as the stereopsis threshold of the subject. If the participant failed at a test of 500”, their stereoacuity was recorded as 800” (2.9 log arcsec).
Statistical analysis
SPSS (version 26.0; IBM Corp., Armonk, NY, USA) was used to analyze the data. All of the stereopsis values were transformed from arcsec to log arcsec. The Shapiro-Wilks test was utilized to explore the data distribution; if the data satisfied a normal distribution, the parameter test was adopted, which detected differences between groups using analysis of variance (ANOVA) and explored these differences using the least significant difference (LSD) method. However, if the data was not normally distributed, the non-parametric test was adopted, which detected differences between groups using the Friedman test and explored the differences between two groups using the Wilcoxon signed-rank test.
Results
According to the Shapiro-Wilks test, most of the data did not meet a normal distribution. Therefore, the non-parametric test was adopted to analyze the data. The medians and interquartile ranges are shown in Table 1. The boxplot of the data is shown in Figures 4,5.
Table 1
Meridians | Magnification | |||||
---|---|---|---|---|---|---|
5% | 10% | 15% | 20% | 25% | 30% | |
Contour-based | ||||||
Overall | 1.59 (0.22) | 1.70 (0.47) | 1.99 (0.38) | 2.30 (0.40) | 2.30 (0.00) | 2.30 (0.40) |
180° | 1.30 (0.23) | 1.59 (0.22) | 1.70 (0.20) | 1.90 (0.23) | 2.30 (0.22) | 2.30 (0.00) |
30° | 1.48 (0.22) | 1.48 (0.22) | 1.70 (0.22) | 1.70 (0.20) | 1.90 (0.38) | 2.30 (0.22) |
45° | 1.48 (0.18) | 1.48 (0.06) | 1.48 (0.22) | 1.70 (0.38) | 1.90 (0.60) | 2.08 (0.45) |
60° | 1.48 (0.40) | 1.70 (0.22) | 1.70 (0.43) | 1.70 (0.47) | 1.70 (0.38) | 1.90 (0.38) |
90° | 1.48 (0.18) | 1.48 (0.22) | 1.48 (0.22) | 1.70 (0.22) | 1.70 (0.05) | 1.90 (0.38) |
Random-dot-based | ||||||
Overall | 1.48 (0.00) | 1.59 (0.22) | 1.90 (0.44) | 2.08 (0.18) | 2.30 (0.62) | 2.90 (0.60) |
180° | 1.30 (0.18) | 1.48 (0.22) | 1.48 (0.43) | 1.70 (0.26) | 1.90 (0.18) | 2.08 (0.06) |
30° | 1.39 (0.18) | 1.48 (0.04) | 1.70 (0.43) | 1.90 (0.20) | 1.90 (0.18) | 2.08 (0.00) |
45° | 1.48 (0.18) | 1.48 (0.22) | 1.70 (0.27) | 1.80 (0.20) | 1.90 (0.18) | 2.08 (0.45) |
60° | 1.30 (0.23) | 1.48 (0.43) | 1.59 (0.47) | 1.70 (0.38) | 1.90 (0.40) | 2.51 (0.62) |
90° | 1.48 (0.25) | 1.48 (0.22) | 1.80 (0.43) | 1.90 (0.38) | 2.08 (0.27) | 2.70 (0.82) |
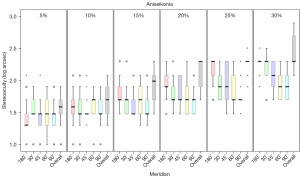
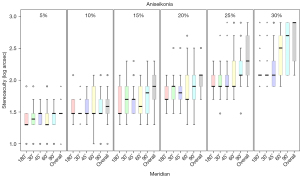
The Friedman test was used to analyze all six groups in the contour-based pattern, (including induced meridional aniseikonia at 180º, 30º, 45º, 60º, 90º, and overall, respectively) in each magnification (including 5%, 10%, 15%, 20%, 25%, and 30%, respectively). The test results showed that a difference existed in each magnification group (Table 2). Combined with the boxplot, the largest value was always in the overall group, which was one of the main factors contributing to the difference. In the random-dot-based pattern, the same trend still existed in the 15%, 20%, 25%, and 30% groups, respectively (Table 2). These results indicated that overall aniseikonia affected stereopsis more than aniseikonia in any one of the meridians.
Table 2
Statistics | Magnification | |||||
---|---|---|---|---|---|---|
5% | 10% | 15% | 20% | 25% | 30% | |
Contour-based | ||||||
Chi-square | 15.008 | 30.533 | 57.984 | 86.725 | 102.139 | 113.103 |
P | 0.010 | <0.001 | <0.001 | <0.001 | <0.001 | <0.001 |
Random-dot-based | ||||||
Chi-square | 6.961 | 5.841 | 13.653 | 31.633 | 53.238 | 85.724 |
P | 0.224 | 0.322 | 0.018 | <0.001 | <0.001 | <0.001 |
Comparison between different meridians
A total of five meridians were involved in this study (180°, 30°, 45°, 60°, and 90°). Horizontal and vertical aniseikonia has been discussed in our previous study (27), while induced oblique aniseikonia was introduced in this study. The difference in stereopsis between the oblique meridians (30°, 45°, and 60°) was analyzed first. A pairwise comparison (Wilcoxon signed-rank test) was carried out three times for three groups, and a significant P value was set as 0.05/3=0.017.
In the contour-based groups, a significant difference was detected with the Freidman test at 25% and 30% magnification. The test value at the 60° meridian was the smallest (Table 3). Meanwhile, in the random-dot-based groups, a marked difference was observed at 30% magnification, and the test value at the 60° meridian was the largest (Table 4).
Table 3
Statistics | Magnification | ||||||||||||||||
---|---|---|---|---|---|---|---|---|---|---|---|---|---|---|---|---|---|
5% | 10% | 15% | 20% | 25% | 30% | ||||||||||||
χ2/Z | P | χ2/Z | P | χ2/Z | P | χ2/Z | P | χ2/Z | P | χ2/Z | P | ||||||
Freidman | χ2=5.474 | 0.065 | χ2=2.935 | 0.230 | χ2=4.441 | 0.109 | χ2=2.211 | 0.331 | χ2=7.969 | 0.019 | χ2=22.182 | 0.001 | |||||
Wilcoxon | |||||||||||||||||
60° vs. 45° | – | – | – | – | – | – | – | – | Z=−2.275 | 0.023 | Z=−3.076 | 0.002 | |||||
30° vs. 45° | – | – | – | – | – | – | – | – | Z=−0.310 | 0.757 | Z=−2.326 | 0.020 | |||||
60° vs. 30° | – | – | – | – | – | – | – | – | Z=2.969 | 0.003 | Z=−3.569 | <0.001 |
Table 4
Statistics | Magnification | ||||||||||||||||
---|---|---|---|---|---|---|---|---|---|---|---|---|---|---|---|---|---|
5% | 10% | 15% | 20% | 25% | 30% | ||||||||||||
χ2/Z | P | χ2/Z | P | χ2/Z | P | χ2/Z | P | χ2/Z | P | χ2/Z | P | ||||||
Freidman | χ2=1.104 | 0.576 | χ2=3.516 | 0.172 | χ2=0.878 | 0.645 | χ2=1.507 | 0.471 | χ2=3.722 | 0.156 | χ2=17.766 | <0.001 | |||||
Wilcoxon | |||||||||||||||||
60° vs. 45° | – | – | – | – | – | – | – | – | – | – | Z=−3.559 | 0.001 | |||||
30° vs. 45° | – | – | – | – | – | – | – | – | – | – | Z=−0.525 | 0.599 | |||||
60° vs. 30° | – | – | – | – | – | – | – | – | – | – | Z=−3.141 | 0.002 |
Three meridians (180°, 45°, and 90°) were chosen for the next analysis. In the contour-based groups, significant differences were detected using the Freidman test at the 10%, 15%, 20%, 25%, and 30% magnifications, respectively (Table 5). The tendency of the test values was 180° > 45° > 90°. In the random-dot-based groups, notable differences were detected at the 25% and 30% magnifications, respectively (Table 6), and the tendency of the test value was 90° > 45° > 180°.
Table 5
Statistics | Magnification | ||||||||||||||||
---|---|---|---|---|---|---|---|---|---|---|---|---|---|---|---|---|---|
5% | 10% | 15% | 20% | 25% | 30% | ||||||||||||
χ2/Z | P | χ2/Z | P | χ2/Z | P | χ2/Z | P | χ2/Z | P | χ2/Z | P | ||||||
Freidman | χ2=0.082 | 0.959 | χ2=7.902 | 0.019 | χ2=31.818 | <0.001 | χ2=31.221 | <0.001 | χ2=38.021 | <0.001 | χ2=47.000 | <0.001 | |||||
Wilcoxon | |||||||||||||||||
180° vs. 45° | – | – | Z=−1.330 | 0.184 | Z=−3.947 | <0.001 | Z=−3.711 | <0.001 | Z=−3.749 | <0.001 | Z=−3.835 | <0.001 | |||||
90° vs. 45° | – | – | Z=−1.690 | 0.091 | Z=−0.787 | 0.431 | Z=−3.279 | 0.001 | Z=−2.836 | 0.005 | Z=−3.866 | <0.001 | |||||
180° vs. 90° | – | – | Z=−1.723 | 0.085 | Z=−3.756 | <0.001 | Z=−4.230 | <0.001 | Z=−4.408 | <0.001 | Z=−4.643 | <0.001 |
Table 6
Statistics | Magnification | ||||||||||||||||
---|---|---|---|---|---|---|---|---|---|---|---|---|---|---|---|---|---|
5% | 10% | 15% | 20% | 25% | 30% | ||||||||||||
χ2/Z | P | χ2/Z | P | χ2/Z | P | χ2/Z | P | χ2/Z | P | χ2/Z | P | ||||||
Freidman | χ2=0.800 | 0.670 | χ2=0.481 | 0.786 | χ2=0.990 | 0.610 | χ2=2.220 | 0.330 | χ2=12.705 | 0.002 | χ2=36.065 | <0.001 | |||||
Wilcoxon | |||||||||||||||||
180° vs. 45° | – | – | – | – | – | – | – | – | Z=−2.186 | 0.029 | Z=−1.017 | 0.309 | |||||
90° vs. 45° | – | – | – | – | – | – | – | – | Z=−1.760 | 0.078 | Z=−4.181 | <0.001 | |||||
180° vs. 90° | – | – | – | – | – | – | – | – | Z=−3.018 | 0.003 | Z=−4.114 | <0.001 |
Comparison between the contour-based and random-dot-based patterns
According to the boxplot, the trend of the test value increasing with the increased magnification of aniseikonia was the same in both the contour-based and random-dot-based patterns. A comparison between the contour-based and random-dot-based patterns was conducted in the 180° and 90° meridians (Table 7). In the 180° meridian, no significant differences were found at 0, 5%, and 10% magnification. However, marked differences were observed at 15%, 20%, 25%, and 30% respectively. The test value of the contour-based pattern was larger than that of the random-dot-based pattern. In the 90° meridian, no significant differences were found at 0, 5%, and 10% magnification. However, notable differences were observed at 15%, 20%, 25%, and 30% respectively. The test value of the random-dot-based was larger than that of the contour-based pattern.
Table 7
Meridians | Magnification | |||||||||||||||||||
---|---|---|---|---|---|---|---|---|---|---|---|---|---|---|---|---|---|---|---|---|
0 | 5% | 10% | 15% | 20% | 25% | 30% | ||||||||||||||
Z | P | Z | P | Z | P | Z | P | Z | P | Z | P | Z | P | |||||||
180° | −0.359 | 0.720 | −1.919 | 0.055 | −1.171 | 0.242 | −2.761 | 0.006 | −3.387 | 0.001 | −3.610 | <0.001 | −3.263 | 0.001 | ||||||
90° | −1.801 | 0.072 | −1.794 | 0.073 | −2.706 | 0.007 | −4.026 | <0.001 | −4.456 | <0.001 | −4.789 | <0.001 |
Discussion
Aniseikonia induced spatial deformation, prism effect, thus affected fusion and stereopsis. Asthenopia and headaches were two main symptoms of patients with aniseikonia (1). The tendency of stereopsis to decrease with the increase of aniseikonia is certain (4,6,27). However, some influencing factors between aniseikonia and stereopsis have not been determined. In this study, overall aniseikonia affected stereopsis more significantly than aniseikonia in all of the meridians. This result was consistent with the study of Atchison et al., which found that meridional aniseikonia had 64% of the effect of overall aniseikonia (6).
In our study, the stereoacuity differences between the oblique meridians (30°, 45°, and 60°) were not significant until the aniseikonia increased up to 20% both in the contour-based or random-dot-based patterns. The difference between the 180°, 45°, and 90° meridians was notable when the aniseikonia increased up to 10% in the contour-based test. In the random-dot-based pattern, a marked difference appeared when the aniseikonia was ≥25%. In Atchison et al.’s study, in which the maximum magnification was 12% (6), no significant difference existed between the three axes (P=0.77–0.85). Also, the stereo test device used in their study, a McGill Vision Research modified random dot stereogram, was a type of random-dot-based test. Therefore, the conclusions of Atchison et al. and those of the present study were consistent.
In our study, the trend of the test value decreased when the meridian changed from 180º to 90º in the contour-based pattern. This may be due to the characteristic of fusing of contour-based circles in this examination. Also, the horizontal part of the circle may play a more important role in fusing and producing stereopsis in a situation where the sizes of two images are different. The more overlapping region in the horizontal direction, the better the stereopsis that may be acquired. Furthermore, the non-overlapping areas in the vertical direction may interfere with the fusing or even result in notable diplopia; however, the depth perception was achieved not in this direction. Matching in the horizontal direction, even if only a small part of the image, may produce the sense of protrusion of a partial section of the circle and help the subject to make a correct choice.
We compared the overlapping areas in the horizontal direction of test symbols between two eyes. The least overlapping appeared in magnification in the 180º meridian, while the most overlapping appeared in magnification in the 90ºmeridian. For the other meridians, the overlapping areas increased gradually as the angle increased from 180º to 90º (Figure 6). The test value change was similar to the change of overlapping areas; that is, the maximum value appeared in the 180º meridian and the minimum value appeared in the 90º meridian, while the other values decreased gradually as the angle increased from 180º to 90º.
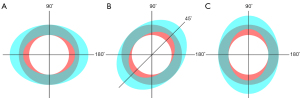
The random-dot-based pattern demonstrated a different tendency. In the contour-based pattern, local stereopsis signified that the key sections matched, as opposed to the whole section matching, which may produce a sense of stereo. However, in the random-dot-based pattern, global stereopsis denotes that the whole section matching could produce a sense of stereo. Generally, fusing in the horizontal direction was much easier than fusing in the vertical direction. Therefore, aniseikonia in the vertical direction may interfere with stereopsis more severely than in the horizontal direction, which is consistent with the experimental results mentioned by Momeni-Moghaddam et al. (34), who reported global stereopsis is more sensitive to vertical misalignment than local stereopsis. The maximum stereopsis value appeared in the 90°meridian, and the minimum value appeared in the 180º meridian, whereas the other values increased gradually as the angle increased from 180º to 90º. However, this tendency was not as obvious as that in the contour-based pattern.
Comparison between the contour-based and random-dot-based patterns showed significant differences in stereoacuity in aniseikonia with 15%, 20%, 25%, and 30% in both the 180° and 90° meridians, respectively. However, the stereopsis value of the contour-based pattern was smaller than that of the random-dot-based pattern in the 90° meridian, while the opposite result was obtained in the 180° meridian. As mentioned, different stereopsis test patterns measure different components of stereopsis; hence, the stereo thresholds of the contour-based and random-dot-based patterns are not in perfect agreement (35).
Local stereopsis refers to the matching of contours or features in two monocular pictures over a limited area of the retina. Without consulting other regions of the retinal field, the disparity between the two monocular perspectives is calculated using the relative locations of these monocular features. Local stereopsis is the process of obtaining depth information from monocularly visible contours by promoting vergence or qualitative depth perceptions, whereas global stereopsis is the process of cross-correlating the left and right images (35,36). Determining which stereotest is "better" is complicated by the fact that different tests assess distinct components of stereopsis. These two types of stereopsis are thought to be mediated by various neuronal populations and are differentially impacted by conditions such as visual disorders, which are one of the most important distinctions (35,37). Typically, local stereopsis results in fusion and calls for a high level of binocular similarity between disparate images. Conversely, global stereopsis does not necessitate similar images in each eye, and these targets typically do not fuse to some extent (38).
Previous studies of aniseikonia commonly used size lenses, which have the advantage of being in accordance with a realistic situation. However, an additional disparity was inevitably introduced and interfered with the accuracy of the judgment. In our test, the additional disparity was converted into a systemic error, which did not affect the judgment of the stereo targets. The limitation was that this situation only appeared in the test with the aid of a computer and did not exist in real life. However, it is difficult to research the relationship between aniseikonia and stereopsis by simulating a situation in real life without introducing extra disparity.
Conclusions
In this study, we observed that the stereoacuity decreased with increasing aniseikonia. Overall aniseikonia affected stereopsis more seriously than meridional aniseikonia. As the angle changed from 180º to 90º, the trend of stereoacuity was improved gradually in the contour-based pattern and deteriorated gradually in the random-dot-based pattern. The stereoacuity of the contour-based pattern was superior to that of the random-dot-based pattern in the 90° meridian, while the opposite result was obtained in the 180° meridian.
Acknowledgments
Funding: This work was supported by the Jilin Provincial Science & Technology Department, China (No. 20190303150SF); the Jilin Provincial Special Fund for Talent Team (No. 2019SCZT030); the Graduate Innovation Fund of Jilin University; and the Innovation and Entrepreneurship Training Program for College Students of Norman Bethune School of Jilin University.
Footnote
Reporting Checklist: The authors have completed the MDAR reporting checklist. Available at https://atm.amegroups.com/article/view/10.21037/atm-22-5575/rc
Data Sharing Statement: Available at https://atm.amegroups.com/article/view/10.21037/atm-22-5575/dss
Conflicts of Interest: All authors have completed the ICMJE uniform disclosure form (available at https://atm.amegroups.com/article/view/10.21037/atm-22-5575/coif). The authors have no conflicts of interest to declare.
Ethical Statement: The authors are accountable for all aspects of the work in ensuring that questions related to the accuracy or integrity of any part of the work are appropriately investigated and resolved. All participants gave their written informed consent before taking part in the study. The study was conducted in accordance with the Declaration of Helsinki (as revised in 2013) and was approved by the ethics committee of the Second Hospital of Jilin University (No. 2020-110).
Open Access Statement: This is an Open Access article distributed in accordance with the Creative Commons Attribution-NonCommercial-NoDerivs 4.0 International License (CC BY-NC-ND 4.0), which permits the non-commercial replication and distribution of the article with the strict proviso that no changes or edits are made and the original work is properly cited (including links to both the formal publication through the relevant DOI and the license). See: https://creativecommons.org/licenses/by-nc-nd/4.0/.
References
- Taylor Kulp MA, Raasch TW, Polasky M. Patients with Anisometropia and Aniseikonia. In: Benjamin WJ. Borish's Clinical Refraction. 2 edition. Butterworth-Heinemann, 2006:1479-508.
- South J, Gao T, Collins A, et al. Aniseikonia and anisometropia: implications for suppression and amblyopia. Clin Exp Optom 2019;102:556-65. [Crossref] [PubMed]
- Lovasik JV, Szymkiw M. Effects of aniseikonia, anisometropia, accommodation, retinal illuminance, and pupil size on stereopsis. Invest Ophthalmol Vis Sci 1985;26:741-50. [PubMed]
- Velez FG, Pineles SL, Rosello N, et al. Meridional aniseikonia-causes, symptoms, and therapies. J AAPOS 2021;25:31.e1-5. [Crossref] [PubMed]
- Hess RF, Ding R, Clavagnier S, et al. A Robust and Reliable Test to Measure Stereopsis in the Clinic. Invest Ophthalmol Vis Sci 2016;57:798-804. [Crossref] [PubMed]
- Atchison DA, Lee J, Lu J, et al. Effects of simulated anisometropia and aniseikonia on stereopsis. Ophthalmic Physiol Opt 2020;40:323-32. [Crossref] [PubMed]
- Okamoto F, Morikawa S, Sugiura Y, et al. Preoperative aniseikonia is a prognostic factor for postoperative stereopsis in patients with unilateral epiretinal membrane. Graefes Arch Clin Exp Ophthalmol 2020;258:743-9. [Crossref] [PubMed]
- Morikawa S, Okamoto F, Murakami T, et al. Aniseikonia following intravitreal ranibizumab treatment for branch retinal vein occlusion. Jpn J Ophthalmol 2021;65:672-9. [Crossref] [PubMed]
- Okamoto F, Sugiura Y, Okamoto Y, et al. Time course of changes in aniseikonia and foveal microstructure after vitrectomy for epiretinal membrane. Ophthalmology 2014;121:2255-60. [Crossref] [PubMed]
- Takabatake M, Higashide T, Udagawa S, et al. Postoperative changes and prognostic factors of visual acuity, metamorphopsia, and aniseikonia after vitrectomy for epiretinal membrane. Retina 2018;38:2118-27. [Crossref] [PubMed]
- Ichikawa Y, Imamura Y, Ishida M. Associations of aniseikonia with metamorphopsia and retinal displacements after epiretinal membrane surgery. Eye (Lond) 2018;32:400-5. [Crossref] [PubMed]
- Hirata A, Nakada H, Mine K, et al. Relationship between the morphology of the foveal avascular zone and the degree of aniseikonia before and after vitrectomy in patients with unilateral epiretinal membrane. Graefes Arch Clin Exp Ophthalmol 2019;257:507-15. [Crossref] [PubMed]
- Moon BG, Yang YS, Chung H, et al. Correlation between macular microstructures and aniseikonia after idiopathic epiretinal membrane removal. Retina 2020;40:1160-8. [Crossref] [PubMed]
- Khanna RK, Dorvault M, Pasco J, et al. Long-term functional outcomes and vision-related quality of life after vitrectomy for epiretinal membrane: a prospective cohort study. Sci Rep 2022;12:2470. [Crossref] [PubMed]
- Krarup T, Nisted I, Kjaerbo H, et al. Measuring aniseikonia tolerance range for stereoacuity - a tool for the refractive surgeon. Acta Ophthalmol 2021;99:e43-53. [Crossref] [PubMed]
- Furr BA. Aniseikonia: A 21st Century Look. J Binocul Vis Ocul Motil 2019;69:43-50. [Crossref] [PubMed]
- Highman VN. Stereopsis and aniseikonia in uniocular aphakia. Br J Ophthalmol 1977;61:30-3. [Crossref] [PubMed]
- Oguchi Y, Mashima Y. The influence of aniseikonia on the VEP by randomdotstereogram. Acta Ophthalmol (Copenh) 1989;67:127-30. [Crossref] [PubMed]
- Zhao L, Wu H. The difference in stereoacuity testing: contour-based and random dot-based graphs at far and near distances. Ann Transl Med 2019;7:193. [Crossref] [PubMed]
- Fawcett SL. An evaluation of the agreement between contour-based circles and random dot-based near stereoacuity tests. J AAPOS 2005;9:572-8. [Crossref] [PubMed]
- Simons K. A comparison of the Frisby, Random-Dot E, TNO, and Randot circles stereotests in screening and office use. Arch Ophthalmol 1981;99:446-52. [Crossref] [PubMed]
- Ateiza A, Davis H. The Effects of Anisometropic Amblyopia on the FNS and TNO Stereotest Thresholds in Four- to Eight-Year-Olds. Br Ir Orthopt J 2019;15:72-81. [Crossref] [PubMed]
- Hartmann EE, Stout AU, Lynn MJ, et al. Stereopsis results at 4.5 years of age in the infant aphakia treatment study. Am J Ophthalmol 2015;159:64-70.e1-2.
- Chung YW, Park SH, Shin SY. Distant stereoacuity in children with anisometropic amblyopia. Jpn J Ophthalmol 2017;61:402-7. [Crossref] [PubMed]
- Fu VL, Birch EE, Holmes JM. Assessment of a new Distance Randot stereoacuity test. J AAPOS 2006;10:419-23. [Crossref] [PubMed]
- Hall C. The relationship between clinical stereotests. Ophthalmic Physiol Opt 1982;2:135-43. [Crossref] [PubMed]
- Xu L, Liu L, Wu H. Evaluation of the Relationship Between Aniseikonia and Stereopsis Using a New Method. Front Med (Lausanne) 2022;9:889398. [Crossref] [PubMed]
- Yildirim C, Altinsoy HI, Yakut E. Distance stereoacuity norms for the mentor B-VAT II-SG video acuity tester in young children and young adults. J AAPOS 1998;2:26-32. [Crossref] [PubMed]
- Wong BP, Woods RL, Peli E. Stereoacuity at distance and near. Optom Vis Sci 2002;79:771-8. [Crossref] [PubMed]
- Stathacopoulos RA, Rosenbaum AL, Zanoni D, et al. Distance stereoacuity: Assessing control in intermittent exotropia. Ophthalmology 1993;100:495-500. [Crossref] [PubMed]
- Seki Y, Wakayama A, Takahashi R, et al. Influence of Test Distance on Stereoacuity in Intermittent Exotropia. Strabismus 2017;25:12-6. [Crossref] [PubMed]
- Wu H, Jin H, Sun Y, et al. Evaluating stereoacuity with 3D shutter glasses technology. BMC Ophthalmol 2016;16:45. [Crossref] [PubMed]
- Zhao L, Wu H. Random Dot E Stereotest underestimates stereopsis at high test distances. Clin Exp Optom 2020;103:918-9. [Crossref] [PubMed]
- Momeni-Moghaddam H, Eperjesi F, Kundart J, et al. Induced vertical disparity effects on local and global stereopsis. Curr Eye Res 2014;39:411-5. [Crossref] [PubMed]
- Vancleef K, Read JCA. Which Stereotest do You Use? A Survey Research Study in the British Isles, the United States and Canada. Br Ir Orthopt J 2019;15:15-24. [Crossref] [PubMed]
- Vancleef K, Read JCA, Herbert W, et al. Overestimation of stereo thresholds by the TNO stereotest is not due to global stereopsis. Ophthalmic Physiol Opt 2017;37:507-20. [Crossref] [PubMed]
- Read JC. Stereo vision and strabismus. Eye (Lond) 2015;29:214-24. [Crossref] [PubMed]
- Richards W, Kaye MG. Local versus global stereopsis: two mechanisms? Vision Res 1974;14:1345-7. [Crossref] [PubMed]
(English Language Editor: A. Kassem)