Optimal radiotherapy modality sparing for cardiac valves in left-sided breast cancer
Highlight box
Key findings
• Intensity-modulated radiation therapy (IMRT) showed the lowest cardiac valve dose in left-sided breast cancer radiotherapy.
What is known and what is new?
• Radiotherapy is related to cardiac valve toxicity in long-term breast cancer survivors.
• This study explored the optimal radiotherapy modality sparing for cardiac valves in patients with left-sided breast cancer.
What is the implication, and what should change now?
• IMRT might be the optimal modality sparing for cardiac valves in this group of patients. Further studies need to be carried out in order to validate the protective role of IMRT on cardiac valves.
Introduction
Breast cancer is the most frequent carcinoma among women worldwide (1). Radiotherapy for breast cancer is an essential part of adjuvant cancer treatment. Adjuvant radiotherapy reduces breast cancer mortality by one-sixth and local recurrence risk by half for patients with breast cancer (2). However, radiotherapy is associated with long-term cardiac toxicity and in long-term breast cancer survivors, cardiovascular disease after radiation therapy has become the leading cause of non-breast cancer death (3,4). Patients with left-sided breast cancer who underwent adjuvant radiotherapy may have experienced clinically significant cardiac radiation exposure (5) and may be at higher risk of these cardiac complications than patients with right-sided breast cancer. Therefore, the cardiotoxicity caused by radiotherapy in left-sided breast cancer is an important problem that needs to be studied extensively.
It had been reported that the mean heart dose (MHD) was linearly related to the incidence of ischemic heart disease (3,6,7). Therefore, decreasing the MHD is essential for avoiding long-term cardiotoxicity. In recent years, an increasing amount of evidence indicated that the dose of cardiac valves needs to be considered. Some studies have identified the left anterior descending artery (LAD) and the left ventricle (LV) as important parts of the heart that are associated with radiation-induced heart disease (6,8,9). Moreover, compared with right-sided radiotherapy for breast cancer, left-sided radiotherapy has been shown to increase the risk of heart and coronary toxicity (10-13), resulting in excess cardiovascular mortality and morbidity (3,14-16). For precise radiotherapy-induced cardiotoxicity studies, it is necessary to consider the distribution of doses within these cardiac valves in addition to MHD. Therefore, an appropriate technique that could minimize cardiac and substructure doses in breast cancer radiation therapy may be beneficial for breast cancer patients.
Postoperative radiotherapy for left-sided breast cancer is usually delivered using 3-dimensional conformal radiotherapy (3D-CRT), fixed-field intensity-modulated radiation therapy (IMRT), and volumetric-modulated arc therapy (VMAT). Conventional 3D-CRT treatment planning is manually optimized, which indicates that the treatment planner selects all beam parameters, including the quantity, directions, shapes, weights of the beams etc., and the computer calculates the resulting dose distribution (17). IMRT is an advanced technique of high-precision radiotherapy driven by computer-optimized planning that allows modulation of beam intensity within treatment fields to obtain highly conformal dose delivery (18). VMAT is a novel radiation therapy technique that delivers the radiation dose continuously as the treatment machine rotates, which can achieve highly conformal dose distributions with improved target volume coverage and sparing of normal tissues (19). However, the optimal radiotherapy modalities sparing for cardiac valves in left-sided breast cancer is still unclear.
The aim of this study was to evaluate the dosimetric differences of heart and cardiac valves between 3D-CRT, IMRT, and VMAT and to find an optimal postoperative radiotherapy which yielded the least dose exposure to the cardiac valves for patients with left-sided breast cancer. We present the following article in accordance with the MDAR reporting checklist (available at https://atm.amegroups.com/article/view/10.21037/atm-22-6633/rc).
Methods
Patient selection
From January 5, 2021, to March 15, 2021, 21 patients were included in the current study. The age of the included patients ranged from 46 to 68 years at the time of treatment. Patients with any personal history of myocardial or coronary artery disease, echocardiographic abnormalities, or previous radiotherapy to the thorax were excluded from the study. The study was conducted in accordance with the Declaration of Helsinki (as revised in 2013). The study was approved by the Chongqing University Cancer Hospital Ethics Committee (No. CZLS2022251-A). All methods were carried out in accordance with the approved guidelines. As this was a retrospective analysis of routine data, we requested and were granted a waiver for individual informed consent from the ethics committee. Patient records/information was anonymized and deidentified prior to analysis.
Immobilization and simulation
Patients were all immobilized in the supine position, with both arms above the head, and head-in-first position with an addition of a 0.8-cm bolus. The bolus was placed to cover the whole chest wall with 2- to 3-cm margins in every direction. The data sets of the computed tomography (CT) scans were obtained utilizing a Philips Big Bore CT scanner (Philips, Amsterdam, the Netherlands) with contrast and a 5-mm slice thickness. The scan scope was from the mandible to the thorax, and the adjacent organs at risk (OARs), such as the heart, lungs, esophagus, trachea, and contralateral breast, were completely covered. All the images were transferred to the Eclipse treatment planning system (TPS; version 15.6, Varian Medical Systems, Inc., Palo Alto, CA, USA) for planning.
Definition of target volumes and OARs
The target volumes and adjacent normal tissues were contoured on the Eclipse TPS. The clinical target volume (CTV) was delineated on each CT data set. The CTV included the chest wall and supraclavicular (SCV) lymph nodes in all patients, +/− the internal mammary lymph nodes and was contoured according to the RTOG consensus. The planning target volume (PTV) was expanded 5 mm based on the CTV and excluded the heart. Then the PTV was retracted 5 mm from the skin and limited posteriorly by the intercostal front. The adjacent OARs (whole heart, ipsilateral and contralateral lungs, esophagus, trachea, spinal cord, and contralateral breast) were contoured. The cardiac valves, cardiac chambers, and coronary vessels were contoured according to the heart atlas reported by Feng et al. (20).
Radiotherapy plans
Three different radiotherapy plans (3D-CRT, IMRT, and VMAT) were created based on the CT data sets of each the 21 cases on the Eclipse TPS. Dose calculations without or with dose optimization were performed using 6-MV photon beams generated by a Varian IX device for all 63 plans. The algorithms of dose-volume optimizer and the progressive resolution optimizer were used for IMRT and VMAT dose optimizations, respectively. The anisotropic analytical algorithm was used for the final dose calculations for all plans (21,22).
3D-CRT plans
Since the PTV could be divided into the chest wall and the SCV regions, two 3D-CRT plans were made for the two regions respectively. The plans for the chest wall region comprised 2 opposed tangential open beams with suitable physical wedges. The plans for the SCV region owned 2 opposed tangential open beams plus 1 open beam with the angles of 30°, and the 3 beams were appended with appropriate physical wedges as needed.
IMRT plans
The IMRT plans contained 7 fields overall, including 2 opposite tangential fields (θc was used to represent the contralateral tangential angle, and the θi represented the ipsilateral tangential angle), 3 surrounding fields located at 10° angles nearby (as the θc+10° and the θi±10°), and 2 fields located at 330° and 30°, respectively. The angles of the collimator and the position of jaws of the fields at 330° and 30° were adjusted for irradiating the PTV in the SCV region, and similar adjustment was adopted for the field at θi to irradiate the whole PTV as well as for the remaining 4 fields to irradiate the PTV in the chest wall region. All the fields adopted the dynamic sliding-window IMRT delivery technique and fixed-jaw technique to deliver radiation doses at a fixed dose rate of 400 monitor units (MUs)/min.
VMAT plans
The VMAT plans contained 5 coplanar arcs overall, including four 60° arcs rotated in clockwise/counterclockwise fashion within the range of [θc−10°, θc+50°], [θc+50°, θc−10°], [θi+10°, θi−50°], and [θi−50°, θi+10°], and one 50° arc rotated in clockwise fashion from 0° and 50°. The angles of the collimators of the four 60° arcs were designed at 30° or 330°, and the corresponding angle to the 50° arc was set to 0°. The jaws of the 50° arc were adjusted to irradiate only the PTV in SCV regions. All the VMAT plans were optimized and calculated with a maximum dose rate of 600 MUs/min.
The prescribed dose was set as 2 Gy per fraction and a total dose of 50 Gy. For 3D-CRT plans, the prescribed 95% isodose covered at least 95% of the PTV, and the percentage of the PTV receiving a radiation dose greater than 115% of the prescription was less than 2%. For IMRT and VMAT plans, the prescribed 100% isodose covered at least 95% of the PTV, and the percentage of the PTV receiving a radiation dose greater than 110% of the prescription dose was less than 2%. For the 3D-CRT, IMRT, and VMAT plans, the ipsilateral lung V20 (percentage of the volume receiving ≥20 Gy) was limited to 35% while the V5 (percentage of the volume receiving ≥5 Gy) was limited to 75%. The maximum dose of spinal cord was less than 40 Gy. The contralateral breast V5 was limited to 10%.
Dose evaluation for the whole heart and heart substructures
The data derived from dose-volume histograms (DVHs) of the 63 plans were collected and analyzed. Figure 1 shows the representative DVHs of the whole heart and cardiac valves in the 3 different plans. For the whole heart, the dosimetric comparative analysis was conducted on the dosimetric/volumetric factors V5, V30, and V40, and on the near-maximum dose (D0.03cc) and mean dose (Dmean). This dosimetric analysis also compared the D0.03cc and the Dmean of 16 cardiac substructures, including the left atrium, LV, right atrium, right ventricle, left main coronary artery, LAD, left circumflex artery, right coronary artery, ascending aorta, descending aorta, pulmonary artery, superior vena cava, aortic valve, pulmonary valve, tricuspid valve, and mitral valve.
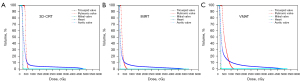
Correlation evaluation
The correlation evaluation was conducted in each radiotherapy modality. The correlations between dosimetric factors of the cardiac valves (Dmean, D0.03) and the dosimetric and volumetric factors of the whole heart (V5, V30, V40, Dmean, and D0.03cc) were analyzed, respectively.
Statistical analysis
Statistical analysis of dosimetric comparisons between groups was carried out using the paired Student t-test in SPSS (IBM Corp., Armonk, NY, USA). A P value <0.05 indicated that the difference was statistically significant. Correlation analysis was conducted with the Pearson correlation coefficient (r) with the corresponding P values. A P value <0.05 was necessary to conclude that 2 variables were correlated. An r value from 0 to 0.39 was considered a weak correlation. Values from 0.4 to 0.59, 0.6 to 0.79, and 0.8 to 1.0 were considered moderate, strong, and very strong correlations, respectively (23).
Results
Demographic profile of patients
The mean age of the patients included in the study was 53.7 years, and the median age was 53 years (range, 46 to 68 years). Additionally, 33.3% of the patients were younger than 50 years, and the remainder were older than 50 years. The most common T stage was T1 (42.8%), followed by T2 (38.1%). N1 (57.1%) was the most common nodal stage, followed by N2 (33.3%). All patients were nonmetastatic (M0). The most frequently encountered stage encountered was stage IIIA (28.6%), followed by stage IIA (23.8%). Other demographic parameters are presented in Table 1. Moreover, 52.4% patients received neoadjuvant therapy and 57.1% patients received adjuvant radiotherapy for the field of the left chest wall and left superior and inferior clavicle.
Table 1
Variable | Parameters (N=21) | Patients, n (%) |
---|---|---|
Age (years) | ≤50 years | 7 (33.3) |
>50 years | 14 (66.7) | |
T stage (according to AJCC 8th edition) | T1 | 9 (42.8) |
T2 | 8 (38.1) | |
T3 | 1 (4.8) | |
T4 | 3 (14.3) | |
N stage (according to AJCC 8th edition) | N1 | 12 (57.1) |
N2 | 7 (33.3) | |
N3 | 2 (9.5) | |
Stagewise distribution | IIA | 5 (23.8) |
IIB | 4 (19.0) | |
IIIA | 6 (28.6) | |
IIIB | 3 (14.3) | |
IIIC | 3 (14.3) | |
Estrogen receptor | Positive | 15 (71.4) |
Negative | 6 (28.6) | |
Progesterone receptor | Positive | 14 (66.7) |
Negative | 7 (33.3) | |
HER2 | Positive | 7 (33.3) |
Negative | 14 (66.7) | |
Target region | Left chest wall field and left superior and inferior clavicle field | 12 (57.1) |
Left chest wall field and internal mammary field and left superior and inferior clavicle field | 9 (42.9) | |
Neoadjuvant therapy | Yes | 11 (52.4) |
No | 10 (47.6) |
AJCC, American Joint Committee on Cancer; HER2, human epidermal growth factor receptor 2.
Dose evaluation for the whole heart and cardiac valves
The average radiation dose to the whole heart and cardiac valves are listed in Table 2. In comparison with IMRT, 3D-CRT and VMAT increased the values of V5, V30, V40, and Dmean of the whole heart (P<0.001). Among the 3 different plans, IMRT had the lowest radiation dose to the Dmean and the D0.03cc of left atrium, right atrium, right ventricle, left main coronary artery, LAD, left circumflex artery, right coronary artery, ascending aorta, pulmonary artery, and superior vena cava (P<0.001).
Table 2
Structures | Substructures | Parameters | 3D-CRT (mean ± SD) | IMRT (mean ± SD) | VMAT (mean ± SD) | P value | ||
---|---|---|---|---|---|---|---|---|
3D-CRT vs. IMRT | 3D-CRT vs. VMAT | IMRT vs. VMAT | ||||||
Whole heart | V5 (%) | 0.17±0.07 | 0.14±0.06 | 0.30±0.08 | 0.000 | 0.000 | 0.000 | |
V30 (%) | 0.05±0.04 | 0.02±0.02 | 0.05±0.03 | 0.000 | 0.025 | 0.000 | ||
V40 (%) | 0.04±0.03 | 0.01±0.01 | 0.03±0.02 | 0.000 | 0.000 | 0.000 | ||
Mean dose (Gy) | 5.82±1.85 | 3.55±1.32 | 6.62±1.86 | 0.000 | 0.000 | 0.000 | ||
D0.03cc (Gy) | 49.71±1.27 | 50.69±3.36 | 51.21±2.26 | 0.144 | 0.004 | 0.171 | ||
Chambers | Left atrium | Mean dose (Gy) | 2.18±0.22 | 0.90±0.13 | 1.51±0.27 | 0.000 | 0.000 | 0.000 |
D0.03cc (Gy) | 4.37±1.14 | 2.85±1.20 | 4.47±1.94 | 0.000 | 0.650 | 0.000 | ||
Left ventricle | Mean dose (Gy) | 7.62±2.47 | 5.15±2.12 | 9.09±2.53 | 0.000 | 0.000 | 0.000 | |
D0.03cc (Gy) | 48.55±1.57 | 47.17±5.94 | 49.86±2.99 | 0.231 | 0.024 | 0.004 | ||
Right atrium | Mean dose (Gy) | 2.11±0.42 | 0.80±0.26 | 1.82±0.66 | 0.000 | 0.006 | 0.000 | |
D0.03cc (Gy) | 4.07±1.87 | 2.12±1.19 | 7.27±4.34 | 0.000 | 0.000 | 0.000 | ||
Right ventricle | Mean dose (Gy) | 7.49±4.00 | 4.26±2.24 | 9.38±3.48 | 0.000 | 0.000 | 0.000 | |
D0.03cc (Gy) | 46.04±6.34 | 27.06±6.99 | 41.90±6.81 | 0.000 | 0.000 | 0.000 | ||
Coronary artery | Left main coronary artery | Mean dose (Gy) | 3.57±0.54 | 1.97±0.48 | 3.30±0.82 | 0.000 | 0.019 | 0.000 |
D0.03cc (Gy) | 3.82±0.73 | 2.09±0.52 | 3.45±0.76 | 0.000 | 0.015 | 0.000 | ||
Left anterior descending artery | Mean dose (Gy) | 30.94±9.77 | 18.87±7.32 | 30.96±7.91 | 0.000 | 0.977 | 0.000 | |
D0.03cc (Gy) | 46.93±6.55 | 36.71±8.37 | 44.91±5.37 | 0.000 | 0.053 | 0.000 | ||
Left circumflex artery | Mean dose (Gy) | 2.88±0.29 | 1.44±0.23 | 2.40±0.36 | 0.000 | 0.000 | 0.000 | |
D0.03cc (Gy) | 3.13±0.35 | 1.62±0.26 | 2.68±0.37 | 0.000 | 0.000 | 0.000 | ||
Right coronary artery | Mean dose (Gy) | 3.15±0.73 | 1.51±0.55 | 3.50±1.56 | 0.000 | 0.142 | 0.000 | |
D0.03cc (Gy) | 3.59±0.84 | 1.88±0.66 | 4.27±1.91 | 0.000 | 0.031 | 0.000 | ||
Great vessels | Ascending aorta | Mean dose (Gy) | 2.91±0.36 | 1.36±0.31 | 2.61±0.58 | 0.000 | 0.001 | 0.000 |
D0.03cc (Gy) | 4.13±0.72 | 2.46±0.82 | 5.78±1.82 | 0.000 | 0.000 | 0.000 | ||
Descending aorta | Mean dose (Gy) | 1.96±0.26 | 0.87±0.14 | 1.13±0.15 | 0.000 | 0.000 | 0.000 | |
D0.03cc (Gy) | 3.31±0.56 | 1.86±0.30 | 2.54±1.60 | 0.000 | 0.043 | 0.068 | ||
Pulmonary artery | Mean dose (Gy) | 4.56±1.46 | 2.63±0.81 | 5.16±1.99 | 0.000 | 0.006 | 0.000 | |
D0.03cc (Gy) | 22.05±17.54 | 9.45±3.87 | 20.38±8.20 | 0.001 | 0.453 | 0.000 | ||
Superior vena cava | Mean dose (Gy) | 2.32±0.38 | 0.88±0.19 | 1.53±0.36 | 0.000 | 0.000 | 0.000 | |
D0.03cc (Gy) | 2.64±0.45 | 1.06±0.23 | 1.86±0.47 | 0.000 | 0.000 | 0.000 | ||
Valves | Aortic valve | Mean dose (Gy) | 2.77±0.37 | 1.27±0.28 | 2.39±0.54 | 0.000 | 0.000 | 0.000 |
D0.03cc (Gy) | 3.37±0.63 | 1.75±0.48 | 3.46±1.20 | 0.000 | 0.545 | 0.000 | ||
Pulmonic valve | Mean dose (Gy) | 6.12±2.88 | 3.44±1.17 | 7.68±3.44 | 0.000 | 0.000 | 0.000 | |
D0.03cc (Gy) | 18.09±16.51 | 6.89±2.64 | 15.96±7.00 | 0.002 | 0.346 | 0.000 | ||
Tricuspid valve | Mean dose (Gy) | 2.39±0.38 | 1.02±0.26 | 2.14±0.62 | 0.000 | 0.010 | 0.000 | |
D0.03cc (Gy) | 2.58±0.48 | 1.14±0.31 | 2.53±0.97 | 0.000 | 0.741 | 0.000 | ||
Mitral valve | Mean dose (Gy) | 2.21±0.36 | 0.93±0.22 | 1.77±0.39 | 0.000 | 0.000 | 0.000 | |
D0.03cc (Gy) | 2.33±0.41 | 1.00±0.24 | 1.93±0.41 | 0.000 | 0.000 | 0.000 |
DVH, dose-volume histogram; 3D-CRT, 3-dimensional conformal radiotherapy; IMRT, intensity-modulated radiotherapy; VMAT, volumetric modulated arc therapy.
Compared with IMRT, the values of the Dmean of aortic valve, pulmonary valve, tricuspid valve, and mitral valve with 3D-CRT were significantly increased by 118.11%, 77.91%, 134.31%, and 137.63% (P<0.001; P<0.001; P<0.001; P<0.001), respectively; the values of the Dmean of aortic valve, pulmonary valve, tricuspid valve, and mitral valve with VMAT were significantly increased by 88.19%, 123.26%, 109.80%, and 90.32% (P<0.001; P<0.001; P<0.001; P<0.001), respectively; the values of the D0.03cc of the aortic valve, pulmonary valve, tricuspid valve, and mitral valve with 3D-CRT were significantly increased by 92.57%, 162.55%, 126.32%, and 133.00% (P<0.001; P=0.002; P<0.001; P<0.001), respectively; and the values of the D0.03cc of aortic valve, pulmonary valve, tricuspid valve, and mitral valve with VMAT were significantly increased by 97.71%, 131.64%, 121.93%, and 93.00% (P<0.001; P<0.001; P<0.001; P<0.001), respectively.
Correlation analysis between the heart dose and valves doses
The Pearson correlation coefficient analysis between dosimetric factors of the cardiac valves (Dmean, D0.03cc) and the dosimetric and volumetric factors of the whole heart (V5, V30, V40, Dmean, and D0.03cc) with corresponding P values was performed for 3D-CRT, IMRT, and VMAT, with the relative results being summarized in Tables 3-5, respectively. Strong correlations of dosimetric parameters were found between most cardiac valves and the whole heart. Specifically as it relates to the valve dosimetric study, the relationship between the local parameters (Dmean and D0.03cc) within valves and the global parameters (V5, V30, V40, and Dmean) of the heart were statistically the strongest.
Table 3
Structures | Substructures | Parameters | Heart | |||||||||||||
---|---|---|---|---|---|---|---|---|---|---|---|---|---|---|---|---|
V5 (%) | V30 (%) | V40 (%) | Mean dose (Gy) | D0.03cc (Gy) | ||||||||||||
r | P | r | P | r | P | r | P | r | P | |||||||
Chambers | Left atrium | Mean dose (Gy) | 0.609 | 0.003 | 0.490 | 0.024 | 0.480 | 0.028 | 0.556 | 0.009 | −0.086 | 0.710 | ||||
D0.03cc (Gy) | 0.134 | 0.561 | 0.189 | 0.411 | 0.189 | 0.412 | 0.153 | 0.508 | −0.438 | 0.047 | ||||||
Left ventricle | Mean dose (Gy) | 0.867 | 0.000 | 0.950 | 0.000 | 0.953 | 0.000 | 0.943 | 0.000 | 0.416 | 0.061 | |||||
D0.03cc (Gy) | 0.233 | 0.309 | 0.379 | 0.090 | 0.413 | 0.063 | 0.360 | 0.109 | 0.927 | 0.000 | ||||||
Right atrium | Mean dose (Gy) | 0.874 | 0.000 | 0.711 | 0.000 | 0.680 | 0.001 | 0.791 | 0.000 | 0.078 | 0.738 | |||||
D0.03cc (Gy) | 0.741 | 0.000 | 0.645 | 0.002 | 0.615 | 0.003 | 0.684 | 0.001 | 0.013 | 0.955 | ||||||
Right ventricle | Mean dose (Gy) | 0.906 | 0.000 | 0.895 | 0.000 | 0.877 | 0.000 | 0.912 | 0.000 | 0.265 | 0.247 | |||||
D0.03cc (Gy) | 0.354 | 0.115 | 0.394 | 0.077 | 0.386 | 0.084 | 0.388 | 0.083 | 0.430 | 0.052 | ||||||
Coronary artery |
Left main coronary artery | Mean dose (Gy) | 0.859 | 0.000 | 0.730 | 0.000 | 0.706 | 0.000 | 0.779 | 0.000 | −0.007 | 0.977 | ||||
D0.03cc (Gy) | 0.668 | 0.001 | 0.542 | 0.011 | 0.528 | 0.014 | 0.590 | 0.005 | 0.083 | 0.720 | ||||||
Left anterior descending artery | Mean dose (Gy) | 0.784 | 0.000 | 0.829 | 0.000 | 0.823 | 0.000 | 0.831 | 0.000 | 0.569 | 0.007 | |||||
D0.03cc (Gy) | 0.381 | 0.089 | 0.412 | 0.063 | 0.401 | 0.072 | 0.420 | 0.058 | 0.633 | 0.002 | ||||||
Left circumflex artery | Mean dose (Gy) | 0.603 | 0.004 | 0.482 | 0.027 | 0.464 | 0.034 | 0.513 | 0.017 | −0.111 | 0.631 | |||||
D0.03cc (Gy) | 0.585 | 0.005 | 0.499 | 0.021 | 0.492 | 0.023 | 0.528 | 0.014 | −0.051 | 0.826 | ||||||
Right coronary artery | Mean dose (Gy) | 0.899 | 0.000 | 0.766 | 0.000 | 0.721 | 0.000 | 0.818 | 0.000 | 0.046 | 0.844 | |||||
D0.03cc (Gy) | 0.825 | 0.000 | 0.696 | 0.000 | 0.650 | 0.001 | 0.743 | 0.000 | 0.028 | 0.905 | ||||||
Great vessels |
Ascending aorta | Mean dose (Gy) | 0.868 | 0.000 | 0.726 | 0.000 | 0.690 | 0.001 | 0.789 | 0.000 | 0.009 | 0.968 | ||||
D0.03cc (Gy) | 0.743 | 0.000 | 0.512 | 0.018 | 0.456 | 0.038 | 0.592 | 0.005 | −0.177 | 0.443 | ||||||
Descending aorta | Mean dose (Gy) | 0.213 | 0.353 | 0.161 | 0.485 | 0.178 | 0.440 | 0.216 | 0.348 | 0.209 | 0.362 | |||||
D0.03cc (Gy) | 0.033 | 0.887 | 0.029 | 0.902 | 0.038 | 0.868 | 0.046 | 0.842 | 0.210 | 0.361 | ||||||
Pulmonary artery | Mean dose (Gy) | 0.702 | 0.000 | 0.592 | 0.005 | 0.562 | 0.008 | 0.636 | 0.002 | −0.161 | 0.485 | |||||
D0.03cc (Gy) | 0.579 | 0.006 | 0.501 | 0.021 | 0.467 | 0.033 | 0.538 | 0.012 | −0.104 | 0.655 | ||||||
Superior vena cava | Mean dose (Gy) | 0.647 | 0.002 | 0.544 | 0.011 | 0.529 | 0.014 | 0.613 | 0.003 | 0.088 | 0.703 | |||||
D0.03cc (Gy) | 0.554 | 0.009 | 0.419 | 0.059 | 0.400 | 0.072 | 0.496 | 0.022 | 0.080 | 0.731 | ||||||
Valves | Aortic valve | Mean dose (Gy) | 0.861 | 0.000 | 0.734 | 0.000 | 0.703 | 0.000 | 0.793 | 0.000 | 0.094 | 0.686 | ||||
D0.03cc (Gy) | 0.782 | 0.000 | 0.645 | 0.002 | 0.610 | 0.003 | 0.704 | 0.000 | 0.069 | 0.767 | ||||||
Pulmonic valve | Mean dose (Gy) | 0.720 | 0.000 | 0.676 | 0.001 | 0.651 | 0.001 | 0.702 | 0.000 | 0.034 | 0.883 | |||||
D0.03cc (Gy) | 0.609 | 0.003 | 0.529 | 0.014 | 0.499 | 0.021 | 0.572 | 0.007 | −0.025 | 0.914 | ||||||
Tricuspid valve | Mean dose (Gy) | 0.829 | 0.000 | 0.759 | 0.000 | 0.739 | 0.000 | 0.805 | 0.000 | 0.196 | 0.394 | |||||
D0.03cc (Gy) | 0.829 | 0.000 | 0.781 | 0.000 | 0.760 | 0.000 | 0.815 | 0.000 | 0.237 | 0.302 | ||||||
Mitral valve | Mean dose (Gy) | 0.803 | 0.000 | 0.768 | 0.000 | 0.764 | 0.000 | 0.799 | 0.000 | 0.130 | 0.576 | |||||
D0.03cc (Gy) | 0.814 | 0.000 | 0.774 | 0.000 | 0.767 | 0.000 | 0.804 | 0.000 | 0.146 | 0.528 |
DVH, dose-volume histogram; 3D-CRT, 3-dimensional conformal radiotherapy.
Table 4
Structures | Substructures | Parameters | Heart | |||||||||||||
---|---|---|---|---|---|---|---|---|---|---|---|---|---|---|---|---|
V5 (%) | V30 (%) | V40 (%) | Mean dose (Gy) | D0.03cc (Gy) | ||||||||||||
r | P | r | P | r | P | r | P | r | P | |||||||
Chambers | Left atrium | Mean dose (Gy) | 0.544 | 0.011 | 0.515 | 0.017 | 0.500 | 0.021 | 0.543 | 0.011 | 0.350 | 0.119 | ||||
D0.03cc (Gy) | 0.190 | 0.409 | 0.218 | 0.342 | 0.237 | 0.302 | 0.169 | 0.463 | 0.110 | 0.636 | ||||||
Left ventricle | Mean dose (Gy) | 0.847 | 0.000 | 0.955 | 0.000 | 0.935 | 0.000 | 0.949 | 0.000 | 0.732 | 0.000 | |||||
D0.03cc (Gy) | 0.529 | 0.014 | 0.572 | 0.007 | 0.624 | 0.003 | 0.583 | 0.006 | 0.862 | 0.000 | ||||||
Right atrium | Mean dose (Gy) | 0.820 | 0.000 | 0.528 | 0.014 | 0.453 | 0.039 | 0.696 | 0.000 | 0.370 | 0.099 | |||||
D0.03cc (Gy) | 0.717 | 0.000 | 0.460 | 0.036 | 0.415 | 0.061 | 0.582 | 0.006 | 0.251 | 0.273 | ||||||
Right ventricle | Mean dose (Gy) | 0.906 | 0.000 | 0.784 | 0.000 | 0.620 | 0.003 | 0.885 | 0.000 | 0.518 | 0.016 | |||||
D0.03cc (Gy) | 0.833 | 0.000 | 0.687 | 0.001 | 0.547 | 0.010 | 0.813 | 0.000 | 0.641 | 0.002 | ||||||
Coronary artery | Left main coronary artery | Mean dose (Gy) | 0.827 | 0.000 | 0.620 | 0.003 | 0.523 | 0.015 | 0.727 | 0.000 | 0.354 | 0.115 | ||||
D0.03cc (Gy) | 0.797 | 0.000 | 0.667 | 0.001 | 0.590 | 0.005 | 0.743 | 0.000 | 0.504 | 0.020 | ||||||
Left anterior descending artery | Mean dose (Gy) | 0.805 | 0.000 | 0.812 | 0.000 | 0.742 | 0.000 | 0.857 | 0.000 | 0.677 | 0.001 | |||||
D0.03cc (Gy) | 0.626 | 0.002 | 0.619 | 0.003 | 0.600 | 0.004 | 0.646 | 0.002 | 0.653 | 0.001 | ||||||
Left circumflex artery | Mean dose (Gy) | 0.489 | 0.024 | 0.627 | 0.002 | 0.576 | 0.006 | 0.602 | 0.004 | 0.154 | 0.506 | |||||
D0.03cc (Gy) | 0.515 | 0.017 | 0.706 | 0.000 | 0.668 | 0.001 | 0.660 | 0.001 | 0.252 | 0.270 | ||||||
Right coronary artery | Mean dose (Gy) | 0.849 | 0.000 | 0.556 | 0.009 | 0.487 | 0.025 | 0.703 | 0.000 | 0.314 | 0.165 | |||||
D0.03cc (Gy) | 0.748 | 0.000 | 0.478 | 0.028 | 0.394 | 0.078 | 0.600 | 0.004 | 0.220 | 0.338 | ||||||
Great vessels | Ascending aorta | Mean dose (Gy) | 0.696 | 0.000 | 0.364 | 0.105 | 0.289 | 0.203 | 0.522 | 0.015 | 0.141 | 0.543 | ||||
D0.03cc (Gy) | 0.400 | 0.072 | 0.031 | 0.892 | 0.003 | 0.990 | 0.191 | 0.407 | −0.092 | 0.692 | ||||||
Descending aorta | Mean dose (Gy) | 0.121 | 0.600 | 0.049 | 0.833 | −0.019 | 0.935 | 0.141 | 0.542 | 0.128 | 0.581 | |||||
D0.03cc (Gy) | 0.036 | 0.877 | −0.034 | 0.885 | −0.126 | 0.588 | 0.035 | 0.880 | −0.056 | 0.809 | ||||||
Pulmonary artery | Mean dose (Gy) | 0.587 | 0.005 | 0.438 | 0.047 | 0.380 | 0.089 | 0.517 | 0.016 | 0.124 | 0.593 | |||||
D0.03cc (Gy) | 0.615 | 0.003 | 0.514 | 0.017 | 0.433 | 0.050 | 0.557 | 0.009 | 0.112 | 0.628 | ||||||
Superior vena cava |
Mean dose (Gy) | 0.636 | 0.002 | 0.253 | 0.268 | 0.176 | 0.446 | 0.450 | 0.041 | 0.158 | 0.495 | |||||
D0.03cc (Gy) | 0.543 | 0.011 | 0.139 | 0.547 | 0.068 | 0.768 | 0.354 | 0.115 | 0.141 | 0.542 | ||||||
Valves | Aortic valve | Mean dose (Gy) | 0.776 | 0.000 | 0.522 | 0.015 | 0.424 | 0.055 | 0.656 | 0.001 | 0.183 | 0.428 | ||||
D0.03cc (Gy) | 0.660 | 0.002 | 0.456 | 0.043 | 0.382 | 0.097 | 0.553 | 0.011 | 0.089 | 0.709 | ||||||
Pulmonic valve | Mean dose (Gy) | 0.720 | 0.000 | 0.615 | 0.003 | 0.517 | 0.016 | 0.669 | 0.001 | 0.158 | 0.493 | |||||
D0.03cc (Gy) | 0.620 | 0.003 | 0.525 | 0.014 | 0.446 | 0.043 | 0.573 | 0.007 | 0.097 | 0.675 | ||||||
Tricuspid valve | Mean dose (Gy) | 0.776 | 0.000 | 0.666 | 0.001 | 0.490 | 0.024 | 0.743 | 0.000 | 0.352 | 0.118 | |||||
D0.03cc (Gy) | 0.792 | 0.000 | 0.692 | 0.001 | 0.524 | 0.015 | 0.764 | 0.000 | 0.381 | 0.088 | ||||||
Mitral valve | Mean dose (Gy) | 0.744 | 0.000 | 0.855 | 0.000 | 0.833 | 0.000 | 0.857 | 0.000 | 0.487 | 0.025 | |||||
D0.03cc (Gy) | 0.774 | 0.000 | 0.862 | 0.000 | 0.838 | 0.000 | 0.877 | 0.000 | 0.512 | 0.018 |
DVH, dose-volume histogram; IMRT, intensity-modulated radiotherapy.
Table 5
Structures | Substructures | Parameters | Heart | |||||||||||||
---|---|---|---|---|---|---|---|---|---|---|---|---|---|---|---|---|
V5 (%) | V30 (%) | V40 (%) | Mean dose (Gy) | D0.03cc (Gy) | ||||||||||||
r | P | r | P | r | P | r | P | r | P | |||||||
Chambers | Left atrium | Mean dose (Gy) | 0.528 | 0.014 | 0.354 | 0.116 | 0.344 | 0.127 | 0.483 | 0.027 | 0.135 | 0.561 | ||||
D0.03cc (Gy) | 0.231 | 0.313 | 0.152 | 0.510 | 0.175 | 0.448 | 0.190 | 0.410 | 0.079 | 0.734 | ||||||
Left ventricle | Mean dose (Gy) | 0.837 | 0.000 | 0.954 | 0.000 | 0.957 | 0.000 | 0.925 | 0.000 | 0.701 | 0.000 | |||||
D0.03cc (Gy) | 0.428 | 0.053 | 0.723 | 0.000 | 0.722 | 0.000 | 0.620 | 0.003 | 0.947 | 0.000 | ||||||
Right atrium | Mean dose (Gy) | 0.839 | 0.000 | 0.719 | 0.000 | 0.690 | 0.001 | 0.826 | 0.000 | 0.431 | 0.051 | |||||
D0.03cc (Gy) | 0.702 | 0.000 | 0.522 | 0.015 | 0.499 | 0.021 | 0.659 | 0.001 | 0.191 | 0.407 | ||||||
Right ventricle | Mean dose (Gy) | 0.917 | 0.000 | 0.810 | 0.000 | 0.756 | 0.000 | 0.896 | 0.000 | 0.435 | 0.049 | |||||
D0.03cc (Gy) | 0.711 | 0.000 | 0.686 | 0.001 | 0.634 | 0.002 | 0.707 | 0.000 | 0.508 | 0.019 | ||||||
Coronary artery | Left main coronary artery | Mean dose (Gy) | 0.801 | 0.000 | 0.664 | 0.001 | 0.603 | 0.004 | 0.784 | 0.000 | 0.370 | 0.098 | ||||
D0.03cc (Gy) | 0.731 | 0.000 | 0.712 | 0.000 | 0.659 | 0.001 | 0.767 | 0.000 | 0.463 | 0.035 | ||||||
Left anterior descending artery | Mean dose (Gy) | 0.810 | 0.000 | 0.879 | 0.000 | 0.864 | 0.000 | 0.878 | 0.000 | 0.572 | 0.007 | |||||
D0.03cc (Gy) | 0.600 | 0.004 | 0.730 | 0.000 | 0.693 | 0.001 | 0.705 | 0.000 | 0.692 | 0.001 | ||||||
Left circumflex artery | Mean dose (Gy) | 0.596 | 0.004 | 0.429 | 0.052 | 0.437 | 0.047 | 0.525 | 0.015 | −0.078 | 0.738 | |||||
D0.03cc (Gy) | 0.637 | 0.002 | 0.558 | 0.009 | 0.575 | 0.006 | 0.614 | 0.003 | 0.050 | 0.830 | ||||||
Right coronary artery | Mean dose (Gy) | 0.833 | 0.000 | 0.665 | 0.001 | 0.629 | 0.002 | 0.801 | 0.000 | 0.223 | 0.330 | |||||
D0.03cc (Gy) | 0.799 | 0.000 | 0.613 | 0.003 | 0.573 | 0.007 | 0.755 | 0.000 | 0.153 | 0.507 | ||||||
Great vessels | Ascending aorta | Mean dose (Gy) | 0.816 | 0.000 | 0.658 | 0.001 | 0.604 | 0.004 | 0.796 | 0.000 | 0.319 | 0.158 | ||||
D0.03cc (Gy) | 0.592 | 0.005 | 0.374 | 0.094 | 0.304 | 0.180 | 0.529 | 0.014 | 0.076 | 0.742 | ||||||
Descending aorta | Mean dose (Gy) | 0.192 | 0.405 | −0.017 | 0.943 | −0.060 | 0.795 | 0.098 | 0.672 | −0.357 | 0.112 | |||||
D0.03cc (Gy) | 0.017 | 0.941 | −0.080 | 0.731 | −0.145 | 0.530 | 0.002 | 0.993 | 0.020 | 0.932 | ||||||
Pulmonary artery | Mean dose (Gy) | 0.627 | 0.002 | 0.430 | 0.052 | 0.380 | 0.089 | 0.568 | 0.007 | 0.077 | 0.740 | |||||
D0.03cc (Gy) | 0.654 | 0.001 | 0.467 | 0.033 | 0.417 | 0.060 | 0.604 | 0.004 | 0.119 | 0.609 | ||||||
Superior vena cava | Mean dose (Gy) | 0.531 | 0.013 | 0.360 | 0.109 | 0.298 | 0.190 | 0.507 | 0.019 | 0.279 | 0.221 | |||||
D0.03cc (Gy) | 0.448 | 0.041 | 0.279 | 0.221 | 0.206 | 0.370 | 0.429 | 0.052 | 0.263 | 0.250 | ||||||
Valves | Aortic valve | Mean dose (Gy) | 0.863 | 0.000 | 0.680 | 0.001 | 0.642 | 0.002 | 0.820 | 0.000 | 0.227 | 0.322 | ||||
D0.03cc (Gy) | 0.807 | 0.000 | 0.618 | 0.003 | 0.598 | 0.004 | 0.745 | 0.000 | 0.082 | 0.723 | ||||||
Pulmonic valve | Mean dose (Gy) | 0.694 | 0.000 | 0.479 | 0.028 | 0.424 | 0.055 | 0.623 | 0.003 | 0.076 | 0.744 | |||||
D0.03cc (Gy) | 0.658 | 0.001 | 0.456 | 0.038 | 0.402 | 0.071 | 0.595 | 0.004 | 0.074 | 0.748 | ||||||
Tricuspid valve | Mean dose (Gy) | 0.825 | 0.000 | 0.720 | 0.000 | 0.689 | 0.001 | 0.801 | 0.000 | 0.368 | 0.101 | |||||
D0.03cc (Gy) | 0.830 | 0.000 | 0.785 | 0.000 | 0.769 | 0.000 | 0.834 | 0.000 | 0.417 | 0.060 | ||||||
Mitral valve | Mean dose (Gy) | 0.842 | 0.000 | 0.788 | 0.000 | 0.781 | 0.000 | 0.842 | 0.000 | 0.452 | 0.039 | |||||
D0.03cc (Gy) | 0.813 | 0.000 | 0.798 | 0.000 | 0.793 | 0.000 | 0.838 | 0.000 | 0.490 | 0.024 |
DVH, dose-volume histogram; VMAT, volumetric modulated arc therapy.
Discussion
For decades, improvements in the curative effects of radiotherapy have resulted in the longer survival time of patients with cancer, and thus the assessment of cardiotoxicity caused by radiotherapy has become increasingly important. The related literature (24-26) has mainly focused on the volumetric dose of the heart, but few studies have examined the cardiac valves specifically. Cardiac valves play a critical role in controlling blood flow through the body. Exposure to radiation increases the risk of cardiac valve damage in breast cancer. Radiotherapy modalities that can decrease the cardiotoxicity have been investigated for a long time. However, the optimal radiotherapy modality sparing for cardiac valves remains unclear. To address this deficit in knowledge, we conducted a comparison of cardiac valve dose among 3D-CRT, IMRT and VMAT in patients with left-sided breast cancer receiving postoperative radiotherapy. The current study revealed that the IMRT had the lowest cardiac valve dose compared with 3D-CRT and VMAT, with better dose distributions and target volume coverage than 3D-CRT. Our findings implied that IMRT might be the optimal modality sparing for cardiac valves in left-sided breast cancer radiotherapy. In addition, correlation analysis demonstrated that the V5, V30, and Dmean of whole heart were strongly associated with cardiac valves dose.
Radiotherapy cardiotoxicity is closely associated with the radiotherapy modality. Compared to 3D-CRT, IMRT has been widely used over the past decade, allowing optimal dose distribution according to individual anatomy (27). It has better dose homogeneity of the target volume and better sparing for the heart and lung. VMAT, as a new radiotherapy technique, was introduced by Otto (28) in 2008 and deliver a intended dose in a single gantry rotation and in a decreased the treatment time compared to IMRT. Numerous studies have compared the influence of the aforementioned 3 modalities on the heart dose distribution. Rastogi et al. (29) demonstrated that the MHD in a 3D-CRT arm was significantly higher than that of an IMRT arm (8.96 vs. 4.57 Gy; P<0.001). Sudha et al.’s study (30) revealed that the MHD value was decreased in the VMAT plan (3D-CRT vs. VMAT: 15.78 vs. 10.86 Gy). Ma et al. (31) conducted a study on a dosimetric comparison of 3D-CRT, IMRT, and VMAT in postoperative radiotherapy for left-sided breast cancer. They found that the MHD in 3D-CRT, IMRT, and VMAT were 7.29, 8.08, and 11.90 Gy, respectively. In our study, we found that the MHD in 3D-CRT, IMRT, and VMAT were 5.82, 3.55, and 6.62 Gy, respectively, which were lower than those of the corresponding values in the 3 studies mentioned above (29-31). Our findings revealed that IMRT had the lowest MHD, and heart V5, V30, and V40 values compared to 3D-CRT and VMAT, which is in line with previous reports (29,32). However, Ma et al. (31) reported that the value of MHD in 3D-CRT was lower than that of IMRT and VMAT, which is inconsistent with our finding. This discrepancy may be due to patient heterogeneity, as well as differences in the requirement of planning target coverage and conformal index, constraints for various OARs, etc.
Our findings show that, compared with 3D-CRT and VMAT, IMRT resulted in the lower radiation exposure of the cardiac valves. In this cohort of patients, for 3D-CRT, IMRT, and VMAT, the pulmonary and aortic valves were affected more frequently compared to the tricuspid and pulmonary valves. Radiation exposure is related to a risk of radiation-induced heart valve damage. In addition to leaflet retraction, pathologic alterations also include valve fibrosis and, ultimately, calcification (33). The time of this pathological process from radiation exposure to the onset of clinically severe heart valve disease spans a period of 10 to 20 years (34). Previous research revealed that radiation dose, interval from radiation exposure, and the treatment of chemotherapy were associated with the risk of cardiac valves injuries (35). The findings of our study suggested that radiotherapy modalities are a potential influencing factor for cardiac valve injury. Different treatment modalities can produce variable cardiac valve doses in left-sided breast cancers. Compared with the conventional 3D-CRT technique, the dosimetric advantage of IMRT and VMAT did translate into a significant reduction of Dmean and D0.03cc to the cardiac valves. However, unexpectedly, IMRT provided the optimal sparing of cardiac valves over the VMAT in this study. VMAT demonstrated higher D0.03cc and mean radiation dose for the cardiac valves. VMAT does have technical and dosimetric strength over IMRT, including better conformity of dose distribution, a reduction in MUs administered, and a reduction in treatment time for more comfortable patient care. This implies that a good treatment plan should achieve a balance between target coverage and critical organ protection.
The cardiac valves play an essential role in the heart but are not routinely contoured in the clinical practice. The radiation dose distribution and toxicity risk to the cardiac valves have been largely ignored in clinical practice and research. Several radiobiological models such as normal tissue complication probability (NTCP) have been developed to estimate the risk of radiotoxicity to normal tissue (36). These models are used in treatment planning to minimize adverse effects from irradiation. However, due to a lack of substructure contouring, these conventional models have been unable to accurately predict the risk of radiation-induced valvular heart disease (VHD) using only conventional heart DVHs. To date, no specific radiation-induced cardiac valvular injury model has been developed. To address this issue, it is urgent to clarify whether MHD or dosimetric/volumetric factors of the heart can accurately predict the radiation doses for cardiac valves. Recently, Naimi et al. (37) conducted a study to evaluate whether cardiac valves doses can be safely derived from the MHD or doses to cardiac chambers. MHD and doses to cardiac valves were shown to be significantly correlated, with the Pearson correlation coefficients ranging from 0.51 to 0.63. Consistent with previous study, our results showed that MHD has a strong to very strong correlation with mean valve dose and a moderate to strong correlation with D0.03cc valve dose. The significant correlations were observed in all radiotherapy modalities including 3D-CRT, IMRT, and VMAT. This finding indicates that MHD is a potential predictive factor for cardiac valve dose. Future studies focusing on the development of dose prediction models to predict the risk of heart valve disease are warranted.
The findings in this report are subject to at least three limitations. First, this study was a retrospective study and had a relatively small sample size. Second, for this is a study on radiotherapy planning, the impact of organ motion, including deformation of the heart substructures and the interplay effect of the various actual clinical scenarios on the dose distribution were not considered. Third, the clinical superiority of IMRT over 3D-CRT and VMAT was not validated by the incidence of cardiotoxic events in the patients with left-sided breast cancer who received radiotherapy treatment. Notwithstanding these limitations, this work offers valuable insights into the protection of the cardiac valves through the selection of the optimal radiotherapy modalities in patients with left-sided breast cancer.
Conclusions
In conclusion, IMRT distributed the lowest dose to the cardiac valves as compared with 3D-CRT and VMAT in left-sided breast cancer radiotherapy. The findings indicate that IMRT might be the best technique for optimal modality sparing for cardiac valves in this group of patients. Further studies need to be carried out in order to confirm the relatively protective value of IMRT for the cardiac valves.
Acknowledgments
Funding: This study was supported by grants from the Discipline Construction and Upgrading Project of National Key Clinical Specialty Construction Project, Science and Technology Research Program of Chongqing Municipal Education Commission (grant No. KJZD-K202000104), the Talent Program of Chongqing (grant No. CQYC20200303137), the Chongqing Municipal Health and Health Commission (grant No. 2019NLTS005), the Chongqing Science and Health Joint Medical Research Project (grant No. 2022MSXM004), and the Natural Science Foundation of Chongqing (grant No. cstc2021jcyj-msxmX0029).
Footnote
Reporting Checklist: The authors have completed the MDAR reporting checklist. Available at https://atm.amegroups.com/article/view/10.21037/atm-22-6633/rc
Data Sharing Statement: Available at https://atm.amegroups.com/article/view/10.21037/atm-22-6633/dss
Conflicts of Interest: All authors have completed the ICMJE uniform disclosure form (available at https://atm.amegroups.com/article/view/10.21037/atm-22-6633/coif). The authors have no conflicts of interest to declare.
Ethical Statement: The authors are accountable for all aspects of the work in ensuring that questions related to the accuracy or integrity of any part of the work are appropriately investigated and resolved. The study was conducted in accordance with the Declaration of Helsinki (as revised in 2013). The study was approved by Chongqing University Cancer Hospital Ethics Committee (No. CZLS2022251-A). All methods were carried out in accordance with the approved guidelines. As this was a retrospective analysis of routine data, we requested and were granted a waiver of individual informed consent from the ethics committee. Patient records and information were anonymized and deidentified prior to analysis.
Open Access Statement: This is an Open Access article distributed in accordance with the Creative Commons Attribution-NonCommercial-NoDerivs 4.0 International License (CC BY-NC-ND 4.0), which permits the non-commercial replication and distribution of the article with the strict proviso that no changes or edits are made and the original work is properly cited (including links to both the formal publication through the relevant DOI and the license). See: https://creativecommons.org/licenses/by-nc-nd/4.0/.
References
- Siegel RL, Miller KD, Fuchs HE, et al. Cancer Statistics, 2021. CA Cancer J Clin 2021;71:7-33. [Crossref] [PubMed]
- Early Breast Cancer Trialists' Collaborative Group (EBCTCG). Effect of radiotherapy after breast-conserving surgery on 10-year recurrence and 15-year breast cancer death: meta-analysis of individual patient data for 10,801 women in 17 randomised trials. Lancet 2011;378:1707-16. [Crossref] [PubMed]
- Darby SC, Ewertz M, McGale P, et al. Risk of ischemic heart disease in women after radiotherapy for breast cancer. N Engl J Med 2013;368:987-98. [Crossref] [PubMed]
- Lee MS, Finch W, Mahmud E. Cardiovascular complications of radiotherapy. Am J Cardiol 2013;112:1688-96. [Crossref] [PubMed]
- Chen M, Zang S, Yu H, et al. Immobilization-assisted abdominal deep inspiration breath-hold in post-mastectomy radiotherapy of left-sided breast cancer with internal mammary chain coverage. Quant Imaging Med Surg 2021;11:3314-26. [Crossref] [PubMed]
- van den Bogaard VA, Ta BD, van der Schaaf A, et al. Validation and Modification of a Prediction Model for Acute Cardiac Events in Patients With Breast Cancer Treated With Radiotherapy Based on Three-Dimensional Dose Distributions to Cardiac Substructures. J Clin Oncol 2017;35:1171-8. [Crossref] [PubMed]
- Jacobse JN, Duane FK, Boekel NB, et al. Radiation Dose-Response for Risk of Myocardial Infarction in Breast Cancer Survivors. Int J Radiat Oncol Biol Phys 2019;103:595-604. [Crossref] [PubMed]
- Nilsson G, Holmberg L, Garmo H, et al. Distribution of coronary artery stenosis after radiation for breast cancer. J Clin Oncol 2012;30:380-6. [Crossref] [PubMed]
- Taylor CW, Nisbet A, McGale P, et al. Cardiac exposures in breast cancer radiotherapy: 1950s-1990s. Int J Radiat Oncol Biol Phys 2007;69:1484-95. [Crossref] [PubMed]
- Wennstig AK, Wadsten C, Garmo H, et al. Long-term risk of ischemic heart disease after adjuvant radiotherapy in breast cancer: results from a large population-based cohort. Breast Cancer Res 2020;22:10. [Crossref] [PubMed]
- Chung SY, Oh J, Chang JS, et al. Risk of Cardiac Disease in Patients With Breast Cancer: Impact of Patient-Specific Factors and Individual Heart Dose From Three-Dimensional Radiation Therapy Planning. Int J Radiat Oncol Biol Phys 2021;110:473-81. [Crossref] [PubMed]
- Wennstig AK, Garmo H, Isacsson U, et al. The relationship between radiation doses to coronary arteries and location of coronary stenosis requiring intervention in breast cancer survivors. Radiat Oncol 2019;14:40. [Crossref] [PubMed]
- Carlson LE, Watt GP, Tonorezos ES, et al. Coronary Artery Disease in Young Women After Radiation Therapy for Breast Cancer: The WECARE Study. JACC CardioOncol 2021;3:381-92. [Crossref] [PubMed]
- Clarke M, Collins R, Darby S, et al. Effects of radiotherapy and of differences in the extent of surgery for early breast cancer on local recurrence and 15-year survival: an overview of the randomised trials. Lancet 2005;366:2087-106. [Crossref] [PubMed]
- Darby SC, McGale P, Taylor CW, et al. Long-term mortality from heart disease and lung cancer after radiotherapy for early breast cancer: prospective cohort study of about 300,000 women in US SEER cancer registries. Lancet Oncol 2005;6:557-65. [Crossref] [PubMed]
- Giordano SH, Kuo YF, Freeman JL, et al. Risk of cardiac death after adjuvant radiotherapy for breast cancer. J Natl Cancer Inst 2005;97:419-24. [Crossref] [PubMed]
- Bakiu E, Telhaj E, Kozma E, et al. Comparison of 3D CRT and IMRT Tratment Plans. Acta Inform Med 2013;21:211-2. [Crossref] [PubMed]
- McDonald MW, Godette KD, Butker EK, et al. Long-term outcomes of IMRT for breast cancer: a single-institution cohort analysis. Int J Radiat Oncol Biol Phys 2008;72:1031-40. [Crossref] [PubMed]
- Teoh M, Clark CH, Wood K, et al. Volumetric modulated arc therapy: a review of current literature and clinical use in practice. Br J Radiol 2011;84:967-96. [Crossref] [PubMed]
- Feng M, Moran JM, Koelling T, et al. Development and validation of a heart atlas to study cardiac exposure to radiation following treatment for breast cancer. Int J Radiat Oncol Biol Phys 2011;79:10-8. [Crossref] [PubMed]
- Bragg CM, Wingate K, Conway J. Clinical implications of the anisotropic analytical algorithm for IMRT treatment planning and verification. Radiother Oncol 2008;86:276-84. [Crossref] [PubMed]
- Zhuang M, Zhang T, Chen Z, et al. Advanced nasopharyngeal carcinoma radiotherapy with volumetric modulated arcs and the potential role of flattening filter-free beams. Radiat Oncol 2013;8:120. [Crossref] [PubMed]
- Woon W, Ravindran PB, Ekayanake P, et al. A study on the effect of detector resolution on gamma index passing rate for VMAT and IMRT QA. J Appl Clin Med Phys 2018;19:230-48. [Crossref] [PubMed]
- Lohr F, Heggemann F, Papavassiliu T, et al. Is cardiotoxicity still an issue after breast-conserving surgery and could it be reduced by multifield IMRT?. Strahlenther Onkol 2009;185:222-30. [Crossref] [PubMed]
- Hurkmans CW, Borger JH, Bos LJ, et al. Cardiac and lung complication probabilities after breast cancer irradiation. Radiother Oncol 2000;55:145-51. [Crossref] [PubMed]
- Zhang F, Zheng M. Dosimetric evaluation of conventional radiotherapy, 3-D conformal radiotherapy and direct machine parameter optimisation intensity-modulated radiotherapy for breast cancer after conservative surgery. J Med Imaging Radiat Oncol 2011;55:595-602. [Crossref] [PubMed]
- Das Majumdar SK, Amritt A, Dhar SS, et al. A Dosimetric Study Comparing 3D-CRT vs. IMRT vs. VMAT in Left-Sided Breast Cancer Patients After Mastectomy at a Tertiary Care Centre in Eastern India. Cureus 2022;14:e23568. [Crossref] [PubMed]
- Otto K. Volumetric modulated arc therapy: IMRT in a single gantry arc. Med Phys 2008;35:310-7. [Crossref] [PubMed]
- Rastogi K, Sharma S, Gupta S, et al. Dosimetric comparison of IMRT versus 3DCRT for post-mastectomy chest wall irradiation. Radiat Oncol J 2018;36:71-8. [Crossref] [PubMed]
- Sudha SP, Seenisamy R, Bharadhwaj K. Comparison of dosimetric parameters of volumetric modulated arc therapy and three-dimensional conformal radiotherapy in postmastectomy patients with carcinoma breast. J Cancer Res Ther 2018;14:1005-9. [Crossref] [PubMed]
- Ma C, Zhang W, Lu J, et al. Dosimetric Comparison and Evaluation of Three Radiotherapy Techniques for Use after Modified Radical Mastectomy for Locally Advanced Left-sided Breast Cancer. Sci Rep 2015;5:12274. [Crossref] [PubMed]
- Coon AB, Dickler A, Kirk MC, et al. Tomotherapy and multifield intensity-modulated radiotherapy planning reduce cardiac doses in left-sided breast cancer patients with unfavorable cardiac anatomy. Int J Radiat Oncol Biol Phys 2010;78:104-10. [Crossref] [PubMed]
- Zou B, Schuster JP, Niu K, et al. Radiotherapy-induced heart disease: a review of the literature. Precis Clin Med 2019;2:270-82. [Crossref] [PubMed]
- Carlson RG, Mayfield WR, Normann S, et al. Radiation-associated valvular disease. Chest 1991;99:538-45. [Crossref] [PubMed]
- Gujral DM, Lloyd G, Bhattacharyya S. Radiation-induced valvular heart disease. Heart 2016;102:269-76. [Crossref] [PubMed]
- Patil S, Pingle SR, Shalaby K, et al. Mediastinal irradiation and valvular heart disease. Cardiooncology 2022;8:7. [Crossref] [PubMed]
- Naimi Z, Bennour E, Neji H, et al. Radiation dose distribution in cardiac valves in left-sided breast cancer radiotherapy. Breast 2021;56:S47-8. [Crossref]
(English Language Editor: J. Gray)