Human serum albumin promotes self-renewal and expansion of umbilical cord blood CD34+ hematopoietic stem/progenitor cells
Highlight box
Key findings
• Human serum albumin has an effect on human umbilical cord blood CD34+ hematopoietic stem/progenitor cells.
What is known and what is new?
• Human serum albumin maintains CD34+ cell expansion and short-term colony formation in vitro.
• Human serum albumin optimizes multilineage reconstitution in immunodeficient mice in vivo.
What is the implication, and what should change now?
• Human serum albumin plays an important role in efficient hematopoietic stem/progenitor cell transplantation strategy.
Introduction
Hematopoietic stem/progenitor cells (HSPCs) play an important role in the hematopoietic system by maintaining adult hematopoiesis through self-renewal capability and multipotent differentiation. Self-renewal capability is the ability of HSPCs to give rise to themselves without differentiation, while multipotent differentiation is the ability to differentiate into all blood cell types. In 1988, Spangrud’s group determined the surface marker phenotype of mouse hematopoietic stem cells as Thy-1lowLin-Sca-1+. In lethally irradiated mice, hematopoietic stem cells were the main population with functional long-term hematopoietic reconstitution capability (1). Rapid engraftment of HSPCs in syngeneic and allogeneic hosts was also possible at radioprotective doses (2).
In the human adult hematopoietic system, the turnover of blood cells is estimated to be more than 1 million per second (3). Because mature blood cells are predominantly short lived, HSPCs are required to continuously provide more differentiated blood cells (4).
HSPCs represent only 0.5–5% of mononuclear cells in human fetal liver, umbilical cord blood, and adult bone marrow (5,6). CD34 is the first surface marker used to enrich human HSPCs. In addition, populations with different levels of CD38 expression play different roles in CD34+ HSPCs. Although the majority of CD34+ cells express CD38, mainly CD38−/lowCD90+ HSPCs induce differentiation into phenotypic and functional multilineage blood cells, including lymphoid and myeloid cells (7,8).
HSPC transplantation is an effective therapeutic strategy for a variety of malignant and nonmalignant diseases, including hematological malignancies such as acute myeloid leukemia and acute lymphoblastic leukemia (8-11). Since HSPCs are increasingly being used, some obstacles have emerged, including limited sources of HSPCs and insufficient doses in clinical treatment. Human umbilical cord blood (UCB) provides a valuable source of HSPC acquisition and is superior to bone marrow (BM) and peripheral blood (PB) mobilization. Several studies have aimed to increase the yield of HSPCs derived from UCB by improving in vitro expansion to overcome the insufficient progenitor cells in adult HSPC transplants (12,13). HSPCs derived from UCB and PB can also be used for culturing in serum-free medium in vitro (14). Red blood cells (RBCs) or platelets generated from UCB-HSPCs in vitro have been shown to have potential for clinical applications (15,16). Although preclinical experiments showed that the in vitro expansion of HSPCs is feasible and safe for transplantation (17), transplantation treatment remains limited by HSPC expansion in vitro. Therefore, it is necessary to enhance cultivation strategies to improve the yield and quality of HSPCs in vitro. Recently, studies have demonstrated that StemRegenin 1 (SR1), a purine derivative obtained by high-throughput screening, can support the in vitro expansion ability of CD34+ cells in the presence of cytokines (18,19). In addition, screened pyrimidine indole derivatives were further modified to obtain UM171, which also supported the expansion of hematopoietic progenitor cells (20,21).
Human serum albumin (HSA) is a group of plasma proteins produced by the human liver. HSA consists of a polypeptide chain that binds to a variety of hydrophobic ligands and maintains blood osmotic pressure. As an important protein in human plasma, HSA accounts for about 50–60% of plasma protein content. Clinical trials have shown that expanded HSPCs can enhance the therapeutic effect significantly after being washed, concentrated, and resuspended in a solution containing HSA for transplantation and injection (22). However, there is no research on the effect of HSA in HSPC culture in vitro. Therefore, we added a small dose of HSA into serum-free medium to explore the effect of HSA in HSPC expansion in vitro and engraftment in vivo. We present the following article in accordance with the ARRIVE reporting checklist (available at https://atm.amegroups.com/article/view/10.21037/atm-22-6383/rc).
Methods
Animals
The immunodeficient mice used in the experiments were female NOD/Shi-scid/IL2Rγnull (NOG) mice (7–8 weeks). A total of 20 mice were obtained for use in this study. Sixteen mice were included, and 4 mice were excluded because of instrumentation or technical failure during animal preparation. All experimental mice were randomized into different groups. The mice were purchased from Vital River Laboratory Animal Technology (Beijing, China) and housed in specific-pathogen-free (SPF) conditions, with free access to food and water. All animal protocols were approved by the Animal Care and Use Committee of Liaocheng People’s Hospital (No. 370726220100172283), in compliance with institutional guidelines for the care and use of animals.
Human UCB-CD34+ cell isolation
We collected samples from consenting donors at the gynecology and obstetrics department of Liaocheng People’s Hospital. Mononuclear cells (MNC) from umbilical cord blood were isolated by density gradient centrifugation using Ficoll-Lympholyte separation (Cedarlane, Burlington, Canada). After labeling of CD34+ cells by MACS CD34 MicroBeads (Miltenyi Biotec, Auburn, CA, USA), CD34 microbead-labeled cells were isolated using an LS column. We performed as the manufacturer’s protocol to operate QuadroMACS separator (Miltenyi Biotec). The purity of the isolated CD34+ cells was about 86.37%±3.56% (n=6). This study was approved by the Ethics Committee of Liaocheng People’s Hospital and Clinical School of Shandong First Medical University (No. 2012004), and was conducted in accordance with the Declaration of Helsinki (as revised in 2013). Written informed consent was obtained from all participants included in this study.
UCB-CD34+ cell in vitro culture
For phenotypic and functional assays, CD34+ cells were cultured in in vitro expansion medium. A protocol was prepared before the study without registration. Iscove’s Modified Dulbecco’s Medium (IMDM; Life Technologies, Carlsbad, CA, USA) supplemented with 1% penicillin/streptomycin/glutamine (P/S/G, Life Technologies), 1% insulin-transferrin-selenium-ethanolamine (ITSX, Life Technologies), 10 mM HEPES (Life Technologies), 10 ng/mL stem cell factor (SCF; PeproTech, East Windsor, NJ, USA), and 100 ng/mL TPO (PeproTech), with or without 0.1% HSA (Albumin Bioscience, Huntsville, AL, USA) was used as an in vitro expansion medium for CD34+ cells. The absolute number of input cells was calculated based on purity and flow cytometry data of isolated CD34+ cells. CD34+ cells were resuspended in in vitro expansion medium and seeded at 1×104/well into 96-well plates (Corning Life Sciences, Tewksbury, MA, USA). The medium was changed every 4 days. UCB-CD34+ cells were cultured at 37 ℃ in 5% CO2 for 8 days.
Flow cytometric analysis
Cells cultured in vitro were dissociated to form a single-cell suspension and washed with fluorescence-activated single cell sorting (FACS) buffer [1% fetal bovine serum (FBS) and 1 mM ethylenediaminetetraacetic acid (EDTA) in phosphate-buffered saline (PBS)] 3 times. Cells obtained from PB, BM, and spleen (SP) in vivo were dissociated to form a single-cell suspension as above. The cells were stained at 4 ℃ for 30–60 minutes in FACS buffer supplemented with a combination of the antibodies and fluorophores listed in Table 1. The stained cells were then analyzed using an Aria II (BD Biosciences, Franklin Lakes, NJ, USA) flow cytometer. The relative percentages and absolute numbers of different cell subsets were determined by flow cytometry. Data analysis was performed using FlowJo 10 software (Tree Star, Inc., San Carlos, CA, USA).
Table 1
Antibody | Clone | Catalog# | Vender |
---|---|---|---|
CD34-APC/Cy7 | 561 | 343614 | BioLegend |
CD49f-PE | eBioGoH3 | 12-0495-82 | eBioscience |
CD90-FITC | 5E10 | 328108 | BioLegend |
CD38-PE/Cy7 | HB7 | 328108 | Invitrogen |
hCD45-APC | 2D1 | 17-9459-42 | Invitrogen |
hCD45-FITC | 2D1 | 11-9459-42 | Invitrogen |
mCD45-PE/Cy7 | 30-F11 | 25-0451-82 | Invitrogen |
mCD45-APC | 30-F11 | 17-0451-82 | Invitrogen |
CD19-FITC | HIB19 | 11-0199-42 | Invitrogen |
CD33-PE | HIM3-4 | 12-0339-42 | Invitrogen |
CD3-Percp/cy5.5 | HIT3 | 300328 | BioLegend |
CD56-APC | CMSSB | 17-0567-42 | Invitrogen |
CD41-PE/Cy7 | HIP8 | 303716 | BioLegend |
CD235a-PE | HIR2 | 12-9987-82 | Invitrogen |
Colony forming cell assay (CFC)
On day 8 of in vitro culture, cells were harvested and resuspended in IMDM. Indicated numbers of cells were transferred to 1 mL of MethoCult H4034 Optimum (STEMCELL Technologies, Vancouver, Canada). The mixture was then transferred to ultra-low attachment 24-well plates (Corning). Cells were cultured at 37 ℃ in 5% CO2 with 100% humidity for 14 days. The plates were then visually scored for colony-forming unit-granulocyte/macrophage (CFU-GM), colony-forming unit-erythrocyte (CFU-E), burst-forming unit-erythroid (BFU-E), colony-forming unit granulocyte (CFU-G), colony-forming unit-macrophage (CFU-M), and colony-forming unit granulocyte/erythrocyte/macrophage/megakaryocyte (CFU-GEMM).
Transplantation and monitoring of human HSCs in NOG mice
Mice (7–8 weeks) were irradiated at a dose of 2 Gy for 4 hours before transplantation. Cells cultured in vitro were harvested and washed 3 times with PBS. They were then resuspended in 50 µL of PBS and injected into the tail vein via intravenous injections. The PB of mice was obtained at 4, 6, 8, 10, 12, 14, and 16 weeks post-transplantation for flow cytometry. At 16 weeks, BP and SP cells were obtained after transplantation for human cell chimera analysis.
Statistical analysis
All data are presented as the mean ± standard deviation (SD). We used the GraphPad Prism software version 7.0 (GraphPad Software, Inc., La Jolla, CA, USA) to do statistical analyses. Statistical differences were evaluated using two-tailed Student’s t-test, with significance at P values <0.05.
Results
MACS-isolated human UCB-CD34+ cells (1×104 cells/well) from were seeded into 96-well plates for in vitro culture for 8 days. The cells were cultured in expansion medium supplemented with or without HSA, respectively. Cells were cultured at 37 ℃ in 5% CO2 for 8 days. The mixture of expanded cells was then obtained and analyzed by flow cytometry. These cells were injected, respectively, into sublethal irradiated severe combined immunodeficient NOG mice via the tail vein. Human cell chimera analysis in PB at 4, 6, 8, 10, 12, 14, and 16 weeks were measured by flow cytometry. Human cell engraftment in BM and SP 16 weeks post-transplantation were also measured by flow cytometry (Figure 1A).
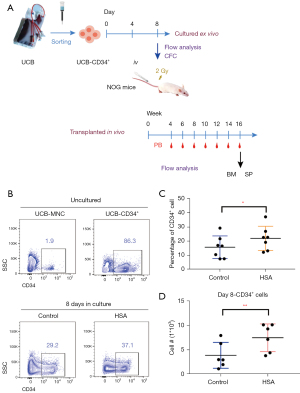
HSA enhanced expansion of CD34+HSPCs in vitro
CD34 is a clinical biomarker for hematopoietic stem cell transplant (HSCT). CD34+ cells are the main source of long-term regenerative potential donor tissue in HSCT (23). As a surface marker, CD34 protein typically diminishes and disappears as cells mature. Thus, we compared the percentage of cells expressing CD34 in live cells among uncultured UCB-MNC, isolated UCB-CD34+ cells, and cultured cells (treated with or without HSA). As shown in Figure 1B, UCB-CD34+ cells account for only about 1–2% of UCB-MNC. We enriched UCB-CD34+ cells by MACS for further culture in vitro (Figure 1B). When in a serum-free culture system (IMDM + 1% ITSX + 1% P/S/G + 10 mM HEPES + 10 ng/mL hSCF + 100 ng/mL hTPO, supplemented with or without HSA) for 8 days, HSA treatment significantly increased CD34+ cells in both percentage and cell number, leading to a twofold increase in the number of CD34+ cells and up to 1.3-fold in percentage (Figure 1B-1D).
HSA sustained short-term colony formation of CD34+HSPC in vitro culture
Based on recent evidence, we also selected CD38, CD49f, and CD90 as common cell surface markers for identifying human HSPCs (24,25). Representative flow cytometry profiles of phenotype-defined subpopulations can be seen in Figure 2A. HSA treatment obviously increased the percentage of CD34+CD38− cells compared with the control group, while there was no significant difference in certain subpopulations’ proportions (CD49f, CD90) of CD34+ cells (Figure 2A and Figure S1A). In addition, we assessed the short-term colony formation capacity of cultured UCB-CD34+ cells with or without HSA treatment by CFC assay. Cultured UCB-CD34+ cells were planted in flat-bottomed 24-well plates in MethoCult H4434 Optimum for 14 days. We scored CFU-GM, CFU-E, BFU-E, CFU-M, and CFU-GEMM visually after 14 days in culture. HSA-treated cells showed a prominent colony-forming potential of BFU-E. A comparable increase with HSA treatment was observed in GM colonies compared to the control group (Figure 2B and Figure S1B). Representative morphological images of BFU-E colonies are shown in Figure S1C. Cultured cells with HSA treatment showed higher potential for short-term colony formation.
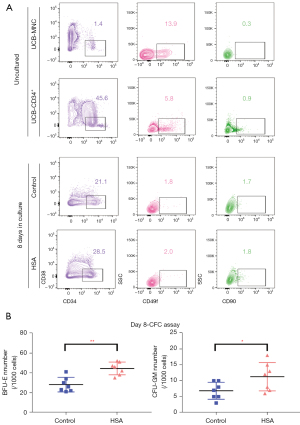
HSA promoted CD34+HSPC engraftment in NOG Mice
Although CFC assays are commonly used to assess the multilineage differentiation potential of HSPCs, xenotransplantation into immunodeficient mice is considered the gold standard for assessing the ability of cultured HSPCs to engraft and reconstitute all blood cell lineages (26). The proportion of human CD45+ (hCD45+) cells in PB of mice gradually increased from week 4 to week 16 post-transplantation. Although human cell engraftment in PB of recipient NOG mice showed no significant difference between 2 groups from week 4 to week 10 post-transplantation, some mice in the HSA group generated a slightly higher level of engraftment at 12 and 14 weeks (Figure 3A). At week 16, the proportion of hCD45+ cells in PB of the mice in the HSA group was higher than that in the control group, demonstrating that HSA-treated cells generated hCD45+ cells more than twofold compared to controls (Figure 3A,3B). These results showed that normal engraftment of human cells could be maintained via HSA treatment in recipient PB.
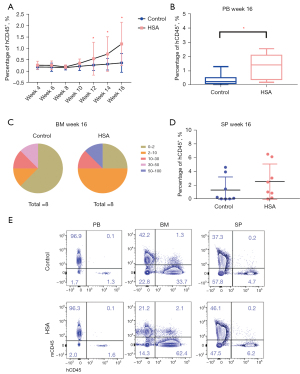
We also detected human cell chimerism in the BM and SP at week 16 post-transplantation. According to the percentage of hCD45+ cells in BM, we regarded a baseline that the level of hCD45+ cells not less than 2% in BM of mice recipients for successful engraftment. These recipients, such as the control group (5 out of 8) and HSA group (2 out of 8) did not achieve positive engraftment (percentage of hCD45+ cells less than 2%). These suggested a slight facilitation in engraftment of human cells after culturing with HSA in vitro (Figure 3C). In addition, there was no significant difference in engraftment in SP between the 2 groups of recipient NOG mice (Figure 3D). Consistent with the results, we showed the flow phenotype of hCD45+ cells analyzed in PB, BM, and SP at week 16 post-transplantation (Figure 3E).
HSA maintained multilineage reconstitution capacity in NOG mice
Assessment of multilineage hematopoietic reconstitution in BM and SP at week 16 post-transplantation was subsequently conducted. We observed that post-transplantation, HSA-treated cells could differentiate into multilineages, including lymphoid and myeloid cells, without significant difference compared to uncultured cells. Therefore, we detected lymphoid cells such as B-lymphoid cells (hCD45+CD19+), T-lymphoid cells (hCD45+CD3+), natural killer cells (NK cells, hCD45+CD56+). We also detected myeloid cells (hCD45+CD33+), megakaryocytes (hCD45−CD41a+), and erythroid cells (hCD45−CD235a+) by flow cytometry. Accordingly, this implied an unaffected multilineage reconstitution of human cells after culturing with HSA in vitro (Figure 4 and Figure S2).
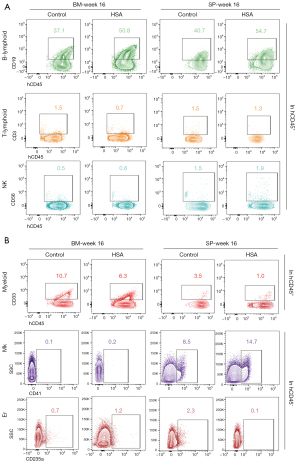
Taken together, these results in vivo suggested that HSA might have slightly promoted the engraftment of cultured UCB-CD34+ cells post-transplantation and retained multilineage potential during culture in vitro.
Discussion
Recently, a number of different approaches have been demonstrated for enhancing efficient expansion of HSPCs. Studies have shown that expansion efficiency can be improved by stromal cocultures, such as autologous umbilical vein endothelial cells (HUVECs) (27), human stromal placenta layer (28), and human mesenchymal stem cells (MSCs) (29). In addition, coating matrix can improve the in vitro expansion efficiency of HSPCs such as collagen I matrix (30) and fibronectin (31,32). Further, molecules for expanding HSPCs have gradually been tapped, including the bioactive peptide SL-13R (33), the activator of Wnt signaling 6-bromoindirubin-3'-oxime (BIO) (34), and Levistilide A (35). It is also feasible to replace FBS with HSA during the process of washing and resuspending HSPCs (22). HSA has multiple functions in in vitro culture. HSA might be used as a “molecular carrier” or provide an amino acid source for HSPCs. In this study, we demonstrated that HSA improved HSPC in vitro expansion. Compared to FBS, HSA as a prescription drug has played an important role in clinical application without immunological rejection. However, HSA with unclear composition has limited study of its mechanism.
Improving the expansion of UCB-derived CD34+ HSPCs in vitro is necessary to meet the demand for clinical transplants and also improve the transplantation reconstruction effect in clinical treatment. There are many methods for improving the expansion and engraftment of HSPCs, including cytokine combinations, small-molecule compounds, coculture systems, and genetic modifications. In our study, we demonstrated that HSA optimized CD34+ cell expansion in vitro and engraftment in vivo. In the future, an efficient HSPC culture strategy could be explored to improve HSPC expansion in vitro and optimize engraftment in vivo.
Conclusions
We demonstrated that HSA was beneficial to maintaining CD34+ cell expansion in vitro. What’s more, HSA optimized the engraftment of cultured UCB-CD34+ cells post-transplantation in vivo and retained multilineage potential during culture in vitro.
Acknowledgments
Funding: This work was supported by the Youth Fund of Liaocheng People’s Hospital (No. LYQN201929).
Footnote
Reporting Checklist: The authors have completed the ARRIVE reporting checklist. Available at https://atm.amegroups.com/article/view/10.21037/atm-22-6383/rc
Data Sharing Statement: Available at https://atm.amegroups.com/article/view/10.21037/atm-22-6383/dss
Conflicts of Interest: All authors have completed the ICMJE uniform disclosure form (available at https://atm.amegroups.com/article/view/10.21037/atm-22-6383/coif). The authors have no conflicts of interest to declare.
Ethical Statement: The authors are accountable for all aspects of the work in ensuring that questions related to the accuracy or integrity of any part of the work are appropriately investigated and resolved. This study was approved by the Ethics Committee of Liaocheng People’s Hospital and Clinical School of Shandong First Medical University (No. 2012004), and was conducted in accordance with the Declaration of Helsinki (as revised in 2013). Written informed consent was obtained from all participants included in this study. All animal protocols were approved by the Animal Care and Use Committee of Liaocheng People’s Hospital (No. 370726220100172283), in compliance with institutional guidelines for the care and use of animals.
Open Access Statement: This is an Open Access article distributed in accordance with the Creative Commons Attribution-NonCommercial-NoDerivs 4.0 International License (CC BY-NC-ND 4.0), which permits the non-commercial replication and distribution of the article with the strict proviso that no changes or edits are made and the original work is properly cited (including links to both the formal publication through the relevant DOI and the license). See: https://creativecommons.org/licenses/by-nc-nd/4.0/.
References
- Spangrude GJ, Heimfeld S, Weissman IL. Purification and characterization of mouse hematopoietic stem cells. Science 1988;241:58-62. [Crossref] [PubMed]
- Uchida N, Tsukamoto A, He D, et al. High doses of purified stem cells cause early hematopoietic recovery in syngeneic and allogeneic hosts. J Clin Invest 1998;101:961-6. [Crossref] [PubMed]
- Ogawa M. Differentiation and proliferation of hematopoietic stem cells. Blood 1993;81:2844-53. [Crossref] [PubMed]
- Seita J, Weissman IL. Hematopoietic stem cell: self-renewal versus differentiation. Wiley Interdiscip Rev Syst Biol Med 2010;2:640-53. [Crossref] [PubMed]
- Civin CI, Strauss LC, Brovall C, et al. Antigenic analysis of hematopoiesis. III. A hematopoietic progenitor cell surface antigen defined by a monoclonal antibody raised against KG-1a cells. J Immunol 1984;133:157-65. [Crossref] [PubMed]
- DiGiusto D, Chen S, Combs J, et al. Human fetal bone marrow early progenitors for T, B, and myeloid cells are found exclusively in the population expressing high levels of CD34. Blood 1994;84:421-32. [Crossref] [PubMed]
- Huang S, Terstappen LW. Lymphoid and myeloid differentiation of single human CD34+, HLA-DR+, CD38- hematopoietic stem cells. Blood 1994;83:1515-26. [Crossref] [PubMed]
- Miller JS, McCullar V, Punzel M, et al. Single adult human CD34(+)/Lin-/CD38(-) progenitors give rise to natural killer cells, B-lineage cells, dendritic cells, and myeloid cells. Blood 1999;93:96-106. [Crossref] [PubMed]
- Williams SF, Lee WJ, Bender JG, et al. Selection and expansion of peripheral blood CD34+ cells in autologous stem cell transplantation for breast cancer. Blood 1996;87:1687-91. [Crossref] [PubMed]
- Wagner JE, Barker JN, DeFor TE, et al. Transplantation of unrelated donor umbilical cord blood in 102 patients with malignant and nonmalignant diseases: influence of CD34 cell dose and HLA disparity on treatment-related mortality and survival. Blood 2002;100:1611-8. [Crossref] [PubMed]
- Christopherson KW 2nd, Paganessi LA, Napier S, et al. CD26 inhibition on CD34+ or lineage- human umbilical cord blood donor hematopoietic stem cells/hematopoietic progenitor cells improves long-term engraftment into NOD/SCID/Beta2null immunodeficient mice. Stem Cells Dev 2007;16:355-60. [Crossref] [PubMed]
- Sauvageau G, Iscove NN, Humphries RK. In vitro and in vivo expansion of hematopoietic stem cells. Oncogene 2004;23:7223-32. [Crossref] [PubMed]
- Sorrentino BP. Clinical strategies for expansion of haematopoietic stem cells. Nat Rev Immunol 2004;4:878-88. [Crossref] [PubMed]
- Gilmore GL, DePasquale DK, Lister J, et al. Ex vivo expansion of human umbilical cord blood and peripheral blood CD34(+) hematopoietic stem cells. Exp Hematol 2000;28:1297-305. [Crossref] [PubMed]
- Xie X, Yao H, Han X, et al. Therapeutic use of red blood cells and platelets derived from human cord blood stem cells. Stem Cells Transl Med 2021;10:S48-53. [Crossref] [PubMed]
- Soboleva S, Åkerstrand H, Miharada K. Transcriptomic analysis of functional diversity of human umbilical cord blood hematopoietic stem/progenitor cells in erythroid differentiation. Int J Hematol 2022;115:481-8. [Crossref] [PubMed]
- Jaroscak J, Goltry K, Smith A, et al. Augmentation of umbilical cord blood (UCB) transplantation with ex vivo-expanded UCB cells: results of a phase 1 trial using the AastromReplicell System. Blood 2003;101:5061-7. [Crossref] [PubMed]
- Boitano AE, Wang J, Romeo R, et al. Aryl hydrocarbon receptor antagonists promote the expansion of human hematopoietic stem cells. Science 2010;329:1345-8. [Crossref] [PubMed]
- Li X, Ma X, Chen Y, et al. Coinhibition of activated p38 MAPKα and mTORC1 potentiates stemness maintenance of HSCs from SR1-expanded human cord blood CD34+ cells via inhibition of senescence. Stem Cells Transl Med 2020;9:1604-16. [Crossref] [PubMed]
- Fares I, Chagraoui J, Gareau Y, et al. Cord blood expansion. Pyrimidoindole derivatives are agonists of human hematopoietic stem cell self-renewal. Science 2014;345:1509-12. [Crossref] [PubMed]
- Csaszar E, Kirouac DC, Yu M, et al. Rapid expansion of human hematopoietic stem cells by automated control of inhibitory feedback signaling. Cell Stem Cell 2012;10:218-29. [Crossref] [PubMed]
- Duchez P, Chevaleyre J, Brunet de la Grange P, et al. Cryopreservation of hematopoietic stem and progenitor cells amplified ex vivo from cord blood CD34+ cells. Transfusion 2013;53:2012-9. [Crossref] [PubMed]
- Shahzad M, Siddiqui RS, Anwar I, et al. Outcomes with CD34-Selected Stem Cell Boost for Poor Graft Function after Allogeneic Hematopoietic Stem Cell Transplantation: A Systematic Review and Meta-Analysis. Transplant Cell Ther 2021;27:877.e1-8. [Crossref] [PubMed]
- Tajer P, Pike-Overzet K, Arias S, et al. Ex Vivo Expansion of Hematopoietic Stem Cells for Therapeutic Purposes: Lessons from Development and the Niche. Cells 2019;8:169. [Crossref] [PubMed]
- Li Y, He M, Zhang W, et al. Antioxidant Small Molecule Compound Chrysin Promotes the Self-Renewal of Hematopoietic Stem Cells. Front Pharmacol 2020;11:399. [Crossref] [PubMed]
- Goyama S, Wunderlich M, Mulloy JC. Xenograft models for normal and malignant stem cells. Blood 2015;125:2630-40. [Crossref] [PubMed]
- Yildirim S, Boehmler AM, Kanz L, et al. Expansion of cord blood CD34+ hematopoietic progenitor cells in coculture with autologous umbilical vein endothelial cells (HUVEC) is superior to cytokine-supplemented liquid culture. Bone Marrow Transplant 2005;36:71-9. [Crossref] [PubMed]
- da Silva CL, Gonçalves R, Crapnell KB, et al. A human stromal-based serum-free culture system supports the ex vivo expansion/maintenance of bone marrow and cord blood hematopoietic stem/progenitor cells. Exp Hematol 2005;33:828-35. [Crossref] [PubMed]
- Xie CG, Wang JF, Xiang Y, et al. Cocultivation of umbilical cord blood CD34+ cells with retro-transduced hMSCs leads to effective amplification of long-term culture-initiating cells. World J Gastroenterol 2006;12:393-402. [Crossref] [PubMed]
- Oswald J, Steudel C, Salchert K, et al. Gene-expression profiling of CD34+ hematopoietic cells expanded in a collagen I matrix. Stem Cells 2006;24:494-500. [Crossref] [PubMed]
- Wilkinson AC, Ishida R, Kikuchi M, et al. Long-term ex vivo haematopoietic-stem-cell expansion allows nonconditioned transplantation. Nature 2019;571:117-21. [Crossref] [PubMed]
- Moghadasi MH, Hajifathali A, Azad M, et al. Expansion of cord blood stem cells in fibronectin-coated microfluidic bioreactor. Hematol Transfus Cell Ther 2022;44:504-11. [Crossref] [PubMed]
- Nii T, Konno K, Matsumoto M, et al. The Bioactive Peptide SL-13R Expands Human Umbilical Cord Blood Hematopoietic Stem and Progenitor Cells In vitro. Molecules 2021;26:1995. [Crossref] [PubMed]
- Sun Q, Zhou Y, Zhu X, et al. Optimizing BIO feeding strategy promotes ex vivo expansion of human hematopoietic stem and progenitor cells. J Biosci Bioeng 2021;131:190-7. [Crossref] [PubMed]
- He M, Xu H, Liu G, et al. Levistilide A Promotes Expansion of Human Umbilical Cord Blood Hematopoietic Stem Cells by Enhancing Antioxidant Activity. Front Pharmacol 2022;13:806837. [Crossref] [PubMed]