Ozone reduces ischemic damage after a stroke by regulating the autophagy of astrocytes
Highlight box
Key findings
• In-vivo and in-vitro studies showed that ozone (O3) reduced stroke damage.
What is known and what is new?
• O3 treatment reduced hypoxia-reperfusion injury in a mouse model.
• O3 reduced brain damage by reducing astrocyte apoptosis.
What is the implication, and what should change now?
• Medical O3 can be applied in ischemic stroke therapy, and its therapeutic mechanism is related to apoptosis.
Introduction
In vascular disease, stroke is caused by cerebral atherosclerosis or atrial fibrillation embolism (1). It is a common disease among the elderly and has high morbidity, disability, and mortality rates. The rapid infusion of the tissue plasminogen activator by triggering thrombolysis has a significant therapeutic effect, but only about 5–10% of patients can dissolve thrombolysis and reach the stroke unit within 4.5 hours of their initial symptoms (2). In the remaining 90% of patients, anti-coagulant, anti-hypertensive, and anti-polymerizable drugs can only be used with caution (3). Thus, new treatment methods for stroke patients need to be explored.
Chen et al. found that ozone (O3) treatment reduced hypoxia-reperfusion injury in a mouse model (4). This treatment method not only rapidly improves the oxygenation of the penumbra area, but also induces many metabolic modifications, such as the upregulation of antioxidant enzymes, which enhances the release of nitric oxide (NO) and carbon monoxide (CO) as vasodilators and enhances local adenosine release (5-8). Thus, preliminary preclinical studies need to be conducted to examine the role of gaseous oxygen-O3 mixtures as potential therapeutic agents for stroke.
A study has shown that cerebral ischemia can open the hemispherical channel of connexin in the astrocyte network and damage the integrity of the blood-brain barrier by losing ion gradients and releasing “danger signals” [including adenosine triphosphate (ATP) and uridine triphosphate (UTP)] (9). Thus, the upregulation of glial fibrillary acidic protein (GFAP) protein can serve as a sign of nerve damage. As the largest number of cells in the brain, astrocytes not only protect and support the central nervous system, but also participate in a series of functions such as water transport, neurotransmitter metabolism and release, and synthesis of neural active substances. In the event of ischemic stroke, astrocytes can produce a variety of protective effects on injured neurons. However, a study has shown that the proliferation of astrocytes after ischemia has a “double-edged sword” effect (10). Additionally, Han et al. found that the autophagy of astrocytes is also a sign of astrocyte activation; thus, the autophagy of astrocytes can serve as a sign of nerve damage (11).
In this study, we established brain injury models to verify the effect of O3 therapy on stroke brain injury. Our experimental data show that O3 therapy significantly reduced brain damage after stroke. Further, we found that B-cell lymphoma 2 (Bcl-2) inhibitors had certain practical significance in the treatment of stroke. We present the following article in accordance with the ARRIVE reporting checklist (available at https://atm.amegroups.com/article/view/10.21037/atm-22-6456/rc).
Methods
Cell culture
The human astrocytoma cell line (A172) was purchased from ATCC® and cultured in Dulbecco’s Modified Eagle Medium (Gibco) containing 10% fetal bovine serum (Hyclone) at 37 °C with 5% carbon dioxide (CO2).
Construction of the OGD/R cell model
The A172 cells were inoculated in 96-well plates at 2.5×104 cells/mL. After 24 h of normal culture, the cells were washed with sugar-free Earle’s solution and cultured at 37 °C under hypoxic conditions (95% nitrogen + 5% CO2). After 0, 2, 4, 8, and 12 h, the sugar-free Earle’s solution was replaced with normal cell culture medium, and the cells continued to be cultured for 24 h under normoxic conditions.
O3 preparation
A high-precision medical O3 meter (HUMAZON, Germany) was used to obtain the O3. The concentration range was 1–56 µg/mL, and the gas flow rate was 0–1,300 mL/min, with a minimum adjustable concentration of 1 µg/mL.
Cell treatment
The OGD/R A172 cells were treated with 20, 30, 60, 90, 120, or 150 µg/mL of O3.
Experimental animals
A total of 30 specific pathogen free (SPF)-grade male Sprague-Dawley rats (weighing 300±20 g) were purchased from the Animal Center. The animals were fed in strict accordance with the animal husbandry rules. The animals were housed at a room temperature of 24±2 °C with 50–60% humidity, and had free access to water and food.
Construction of the MCAO rat model
The experiments began after 7 days of adaptive feeding. The rats were anesthetized with 3% sodium pentobarbital (50 mg/kg) by injection. The middle cerebral artery occlusion (MCAO) model was established in all the rats except those in the sham-operated group. The hair on the neck of each rat was removed, after which the skin was disinfected with 75% ethanol, and a 2-cm incision was made in the midline of the neck. Subsequently, the muscles were bluntly separated. A “V”-shaped incision was made at a distance of about 6 mm from the bifurcation of the common carotid artery (CCA), and a wire plug was inserted from the CCA to the internal carotid artery and into the cerebral artery. The plug was fixed for 2 h and then removed, and blood flow was restored to complete the modeling. The sham-operated group was operated on as described above, but no bolus was inserted. After waking up, the rats could not fully extend their left forelimbs when lifting their tails, and turned to their opposite side when crawling, which indicated the success of the model. This animal experiment was approved by the Institutional Animal Ethics Committee (No. 2003008) and in compliance with the Animal Care Guidelines for the Care and Use of Guizhou Medical University. A protocol was prepared before the study without registration.
Animal grouping
The SD rats were randomly divided into the sham-operated (control) group, MCAO model group, MCAO + O3 group, MCAO + propofol group, and MCAO + O3 + propofol group. O3 concentration selection 20 µg/mL, if the concentration is higher than that, the body of rats may be damaged. Propofol was intraperitoneally injected with 30 mg/kg for 30 min.
2,3,5-triphenyte-trazoliumchloride (TTC) staining
After anesthesia, the rat brain tissues were isolated, and the brain tissues were cut into 2-mm-thick coronal sections by freezing. Subsequently, the brain sections were processed with 2% TTC for 30 min, protected from light at 37 °C. After staining, the brain sections were placed in 4% paraformaldehyde and fixed overnight at 4 °C. The following day, the sections were observed under the microscope and photographed. The white area indicated the infarcted area, and the red area indicated the non-infarcted area. The infarct area of the sections was calculated using IPP6.0.
ELISA analysis
After anesthesia, fresh cerebral cortical tissue on the ischemic side was taken from the rats after cardiac perfusion, the surface appendages were removed, then it was washed with cold saline to make brain homogenate at a concentration of 10%. For the cells, we collected the supernatant of the cell culture fluid. The lactate dehydrogenase (LDH) and glutamate content were measured as per the instructions of each kit.
Western blot
The total protein was extracted with radio-Immunoprecipitation Assay (RIPA) lysate from the brain tissue or A172 cells of each group. The BCA method was used to determine the concentration of the proteins, and 30 µg of the protein samples was separated by sodium dodecyl-sulfate polyacrylamide gel electrophoresis and transferred to the polyvinylidene fluoride (PVDF) membranes using the wet-transfer method. The membranes were blocked with 5% skim milk powder and incubated with primary antibody (1:1,000) at 4 °C overnight. The primary antibody was then washed away, the membranes were invubated with the goat anti-rabbit secondary antibody (Abcam, 1:5,000) at room temperature for 1h. After electrochemical luminescence (ECL) visualization, the relative expression levels of the target bands were derived by the gray scale ratio with the internal reference. The primary antibodies included anti-glial fibrillary acidic protein (GFAP) (Abcam, ab4674), anti-GAPDH (Abcam, ab76523), anti-Bcl-2 (Abcam, ab692), and anti-LC3B (Abcam, ab192890).
Quantitative reverse transcription polymerase chain reaction (RT-qPCR)
Total ribonucleic acid (RNA) was extracted from the brain tissue or A172 cells using the Trizol method. The RNA was reverse transcribed using the SYBR®PremixExTaqTM kit (Takara). Subsequently, PCR amplification was conducted using the SYBR Green PCR kit (Applied Biosystems). The 2-ΔΔCt method was used to analyze the results. The primer sequences were as follows: GFAP: 5'-CACCTACAGGAAATTGCTGGAGG-3' (forward), 5'-CCACGATGTTCCTCTTGAGGTG-3' (reverse); Bcl-2: 5'-CCTGTGGATGACTGAGTACCTG-3' (forward), 5'-AGCCAGGAGAAATCAAACAGAGG-3' (reverse); ATG3: 5'-TAAGGCTGACGCTGGAGGTGAA-3' (forward), 5'-GTGCTCAACTGTTAAAGGCTGCC-3' (reverse); GAPDH: 5'-GAAGGTGAAGGTCGGAGTC-3' (forward), 5'-GAAGATGGTGATGGGATTTC-3' (reverse).
Immunofluorescence staining
Brain tissue sections from each group were fixed with 4% paraformaldehyde and placed in 30% sucrose solution for dehydration. Frozen sections (–20 °C, stored in antifreeze solution) were hydrated with phosphate-buffered saline for 20 min. After rinsing, the sections were blocked with 10% goat serum for 1 h and incubated with the primary antibody (GFAP, 1:20) overnight at 4 °C. After washing, the sections were treated with goat anti-rabbit immunoglobulin G-fluorescein isothiocyanate (lgG-FITC) (1:200) for 1 h. After washing, the sections were sealed with glycerol. The expression and distribution of GFAP were observed under a fluorescence microscope. The results were quantitatively analyzed by ImageJ software.
Statistical analysis
All the above experiments were independently repeated 3 times, and the obtained data are expressed as the mean ± standard deviation (SD). The data were analyzed using SPSS 21.0 software (IBM-SPSS Inc., Chicago, IL, USA). One way ANOVA was used for comparison between multiple groups, and LSD or snk-q was used for pairwise comparison between groups. A P value <0.05 denotes a statistically significant difference.
Results
O3 reduces brain damage in the in-vitro stroke model
We applied the experimental methods of Frosini et al. to establish the in-vitro optimal ischemia-like [oxygen-glucose deprivation and reoxygenation (OGD/R)] experimental conditions (12). As Figure 1A,1B show, by detecting the changes in GFAP expression, we determined that the best time for OGD/R was 8 hours. Consistent with this, the contents of the tissue damage markers of LDH and glutamate increased significantly after OGD/R treatment (figure 1C,1D). We also found that O3 significantly reduced the LDH and glutamate content of the A172 cells treated with OGD/R (figure 1C,1D). Thus, O3 may reduce brain damage after stroke.
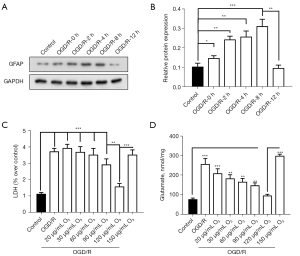
O3 reduces brain infarction after stroke in vivo
To further evaluate the therapeutic significance of O3 for stroke, we used a transient middle cerebral artery occlusion (tMCAO) mouse model to evaluate the effect of O3 on brain damage after stroke in vivo (13). As Figure 2A,2B show, O3 significantly reduced the degree of brain damage in the tMCAO mouse model. In addition, we found that O3 significantly reduced the LDH and glutamate content of the A172 cells treated with OGD/R (figure 2C,2D). Further, the Western blot and immunofluorescence results showed that O3 significantly reduced stroke-induced GFAP activation in vivo (figure 3A-3D). These data indicated that O3 might reduce brain damage after stroke.
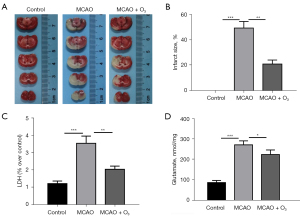
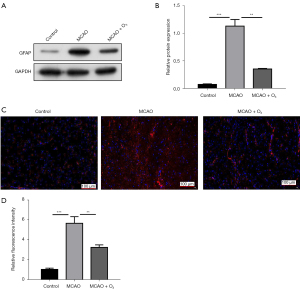
O3 alleviates stroke-induced brain damage by reducing the autophagy of astrocytes
A study (14) has reported that autophagy in astrocytes induces the activation of astrocytes, which is common after stroke and causes brain damage. Thus, we speculated that the effect of O3 in alleviating brain damage might be related to the autophagy of astrocytes. The RT-qPCR results showed that O3 significantly reduced stroke-induced autophagy gene activation (figure 4A). The Western blot experimental results showed that O3 significantly reduced the protein levels of Bcl-2 and LC3II (figure 4B,4C). In addition, the ectopic expression of Bcl-2 reduced the rescuing effect of O3 on brain injury (figure 4D-4F). These results suggested that O3 plays a neuroprotective role by inhibiting stroke-induced autophagy.
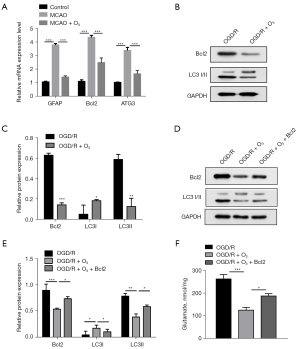
Propofol (the BCL-2 inhibitor) alleviates stroke-induced brain damage
The above experimental data indicated that O3 inhibited the key protein of autophagy, Bcl-2. We speculated that Bcl-2 inhibitors might also be effective in alleviating stroke-induced brain damage. We used the Bcl-2 inhibitor propofol to carry out the relevant experimental verification in the tMCAO mouse model (15). We found that propofol inhibited the increase in LC3II protein expression induced by stroke (figure 5A,5B). In addition, the combined effect of O3 and propofol further inhibited stroke-induced autophagy (figure 5A,5B). The LDH test results showed that propofol significantly alleviated the stroke-induced brain injury (figure 5C). Further, the combined effect of O3 and propofol provided a better cerebral protective effect after stroke (figure 5C).
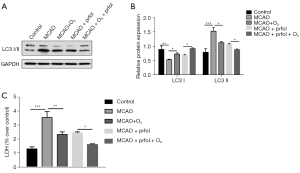
Discussion
Stroke, which is an important part of cardiovascular and cerebrovascular diseases, poses a serious threat to people’s health (15-18). There has been great progress in research on the molecular mechanisms and clinical treatment of stroke in recent years; however, the effective treatment options that can be administered to stroke patients in clinical practice remain very limited. Thus, the study of new and effective treatments for stroke is of great clinical significance.
In this study, consistent with the findings of Clavo et al. (19), we found that O3 significantly alleviated stroke-induced brain damage. In addition, our RT-qPCR and Western blot results suggested that O3 plays a protective role in the brain by regulating the autophagy of astrocytes. However, the relevant molecular mechanisms were not thoroughly examined in this study. The ozone concentration commonly used in the treatment of osteoarthritis and lumbar disc herniation is 30–50 µg/mL. The common concentration of O3 in large autohemotherapy is 20–30 µg/mL. High concentration of O3 may cause damage to neurons in rats, so it is particularly important to select an appropriate concentration of O3 so that the treatment of O3 can play a greater role without harming the body tissues. In this experiment, the concentration of O3 is 20 µg/mL, if the concentration is higher than, the body of rats may be damaged.
Previous studies have shown that O3 is related to the reactive oxygen species (ROS) pathway (20,21). In this present study, we found that O3 had a regulatory effect on Bcl-2 gene expression. Additionally, research has revealed that there is crosstalk between Bcl-2 and the ROS pathway in autophagy (22). Thus, we speculated that O3 may play a protective role after stroke through the ROS pathway. This conjecture has great research value and will be one of the main directions of our follow-up research. Our results also showed that Bcl-2 inhibitors had a neuroprotective effect after stroke. In addition, O3 and Bcl-2 inhibitors had a significant combined neuroprotective effect. However, when evaluating the neuroprotective effect of Bcl-2 inhibitors in this study, the evaluation targets used were insufficient. Our follow-up research will further improve the relevant evaluation indicators.
In addition, our combination of O3 and propofol provides better brain protection after stroke. Propofol is an antioxidant, which may affect many aspects of oxidative stress. In the free radical reaction system, the phenol structure can provide a hydrogen atom, which can reduce the damage to the body caused by excessive free radicals. The trace plasma concentration of propofol can play an antioxidant role in protecting cell membrane. Because of the fat solubility of propofol, it is easier to accumulate on the lipid bilayer of cells, thus improving the ability of cells to resist oxidative damage. The rat cerebral cortex astrocytes were exposed to peroxide to form an oxidative stress model. After 2 hours of propofol treatment, it was found that the Glu release was significantly lower than that of the untreated group (14,20). Therefore, the combination of O3 and propofol in this study has a better protective effect on stroke.
Conclusions
Overall, this study demonstrated that O3 had a protective effect on the brain after stroke an in vitro and in vivo models. In addition, we verified that O3 plays a neuroprotective role after stroke by regulating autophagy (Figure 6). Our findings may provide a theoretical basis for the treatment of stroke patients in the future.
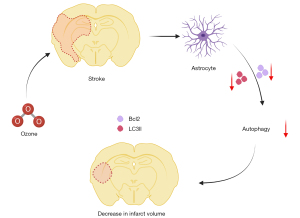
Acknowledgments
Funding: This work was financially supported by the National Natural Science Foundation (No. 81860321), the Science and Technology Department of Guizhou Province (Basic Science and Technology Cooperation [2017]1149), the Technology Project of the Baiyun District of Guiyang City (No. [2019]36), the Guizhou Science and Technology Planning Project Guizhou Science and Technology Integration foundation (No. ZK[2021] General 489), Doctoral Foundation of Guizhou Medical University (No. GYFYBSKY-2021-27), Guiyang Baiyun District Science and technology plan project (No. [2019]36), and the Jingmen 2020 Science and Technology Plan Project (No. 2020YFZD030).
Footnote
Reporting Checklist: The authors have completed the ARRIVE reporting checklist. Available at https://atm.amegroups.com/article/view/10.21037/atm-22-6456/rc
Data Sharing Statement: Available at https://atm.amegroups.com/article/view/10.21037/atm-22-6456/dss
Conflicts of Interest: All authors have completed the ICMJE uniform disclosure form (available at https://atm.amegroups.com/article/view/10.21037/atm-22-6456/coif). The authors have no conflicts of interest to declare.
Ethical Statement: The authors are accountable for all aspects of the work in ensuring that questions related to the accuracy or integrity of any part of the work are appropriately investigated and resolved. This animal experiment was approved by the Institutional Animal Ethics Committee (No. 2003008) and in compliance with the Animal Care Guidelines for the Care and Use of Guizhou Medical University.
Open Access Statement: This is an Open Access article distributed in accordance with the Creative Commons Attribution-NonCommercial-NoDerivs 4.0 International License (CC BY-NC-ND 4.0), which permits the non-commercial replication and distribution of the article with the strict proviso that no changes or edits are made and the original work is properly cited (including links to both the formal publication through the relevant DOI and the license). See: https://creativecommons.org/licenses/by-nc-nd/4.0/.
References
- Lovett JK, Dennis MS, Sandercock PA, et al. Very early risk of stroke after a first transient ischemic attack. Stroke 2003;34:e138-40. [Crossref] [PubMed]
- Lees KR, Bluhmki E, von Kummer R, et al. Time to treatment with intravenous alteplase and outcome in stroke: an updated pooled analysis of ECASS, ATLANTIS, NINDS, and EPITHET trials. Lancet 2010;375:1695-703. [Crossref] [PubMed]
- Back T. Pathophysiology of the ischemic penumbra--revision of a concept. Cell Mol Neurobiol 1998;18:621-38. [Crossref] [PubMed]
- Chen H, Xing B, Liu X, et al. Ozone oxidative preconditioning inhibits inflammation and apoptosis in a rat model of renal ischemia/reperfusion injury. Eur J Pharmacol 2008;581:306-14. [Crossref] [PubMed]
- Ricci L, Valoti M, Sgaragli G, et al. Neuroprotection afforded by diazepam against oxygen/glucose deprivation-induced injury in rat cortical brain slices. Eur J Pharmacol 2007;561:80-4. [Crossref] [PubMed]
- Ricci L, Valoti M, Sgaragli G, et al. Protection by taurine of rat brain cortical slices against oxygen glucose deprivation- and reoxygenation-induced damage. Eur J Pharmacol 2009;621:26-32. [Crossref] [PubMed]
- Ricci L, Valoti M, Sgaragli G, et al. Taurine-like GABA aminotransferase inhibitors prevent rabbit brain slices against oxygen-glucose deprivation-induced damage. Amino Acids 2012;42:2139-47. [Crossref] [PubMed]
- Peralta C, Xaus C, Bartrons R, et al. Effect of ozone treatment on reactive oxygen species and adenosine production during hepatic ischemia-reperfusion. Free Radic Res 2000;33:595-605. [Crossref] [PubMed]
- Lananna BV, Nadarajah CJ, Izumo M, et al. Cell-Autonomous Regulation of Astrocyte Activation by the Circadian Clock Protein BMAL1. Cell Rep 2018;25:1-9.e5. [Crossref] [PubMed]
- Grafstein B. Subverting the hegemony of the synapse: complicity of neurons, astrocytes, and vasculature in spreading depression and pathology of the cerebral cortex. Brain Res Rev 2011;66:123-32. [Crossref] [PubMed]
- Han B, Zhang Y, Zhang Y, et al. Novel insight into circular RNA HECTD1 in astrocyte activation via autophagy by targeting MIR142-TIPARP: implications for cerebral ischemic stroke. Autophagy 2018;14:1164-84. [Crossref] [PubMed]
- Frosini M, Contartese A, Zanardi I, et al. Selective ozone concentrations may reduce the ischemic damage after a stroke. Free Radic Res 2012;46:612-8. [Crossref] [PubMed]
- Pan N, Lu LY, Li M, et al. Xyloketal B alleviates cerebral infarction and neurologic deficits in a mouse stroke model by suppressing the ROS/TLR4/NF-κB inflammatory signaling pathway. Acta Pharmacol Sin 2017;38:1236-47. [Crossref] [PubMed]
- Su PW, Zhai Z, Wang T, et al. Research progress on astrocyte autophagy in ischemic stroke. Front Neurol 2022;13:951536. [Crossref] [PubMed]
- Adembri C, Venturi L, Pellegrini-Giampietro DE. Neuroprotective effects of propofol in acute cerebral injury. CNS Drug Rev 2007;13:333-51. [Crossref] [PubMed]
- Esenwa C, Gutierrez J. Secondary stroke prevention: challenges and solutions. Vasc Health Risk Manag 2015;11:437-50. [PubMed]
- Dunbar M, Kirton A. Perinatal Stroke. Semin Pediatr Neurol 2019;32:100767. [Crossref] [PubMed]
- Sarikaya H, Ferro J, Arnold M. Stroke prevention--medical and lifestyle measures. Eur Neurol 2015;73:150-7. [Crossref] [PubMed]
- Clavo B, Catalá L, Pérez JL, et al. Ozone Therapy on Cerebral Blood Flow: A Preliminary Report. Evid Based Complement Alternat Med 2004;1:315-9. [Crossref] [PubMed]
- Voter KZ, Whitin JC, Torres A, et al. Ozone exposure and the production of reactive oxygen species by bronchoalveolar cells in humans. Inhal Toxicol 2001;13:465-83. [Crossref] [PubMed]
- Liu HH, Wu YC, Chen HL. Production of ozone and reactive oxygen species after welding. Arch Environ Contam Toxicol 2007;53:513-8. [Crossref] [PubMed]
- Cheng HB, Bo Y, Shen WX, et al. Longikaurin E induces apoptosis of pancreatic cancer cells via modulation of the p38 and PI3K/AKT pathways by ROS. Naunyn Schmiedebergs Arch Pharmacol 2015;388:623-34. [Crossref] [PubMed]
(English Language Editor: L. Huleatt)