Impact of carbapenem antibiotics on mycophenolate mofetil in hematopoietic stem cell transplant patients with interruption of the enterohepatic recycling: a retrospective study
Highlight box
Key findings
• After intravenous administration of carbapenem antibiotics (CBP), the pre-dose plasma concentration (C0) of mycophenolic acid (MPA) decreased significantly in hematopoietic stem cell transplant patients (P<0.05) even when mycophenolate mofetil is used in combination with cyclosporine and rifaximin.
What is known and what is new?
• Oral antibiotics can affect MPA concentration by reducing intestinal flora-mediated enterohepatic recycling.
• This study is the first to evaluate the effect of intravenous CBP on MPA C0 in hematopoietic stem cell transplant patients when enterohepatic recycling is interrupted by cyclosporine and gut decontamination. CBP may reduce the C0 of MPA through the mechanism unrelated to the interruption of enterohepatic recycling.
What is the implication, and what should change now?
• If antibiotics must be used, and CBP in particular, therapeutic drug monitoring should be performed to ensure adequate exposure.
Introduction
Mycophenolate mofetil (MMF) is widely used in the prophylaxis for graft-versus-host disease (GVHD) following hematopoietic stem cell transplantation (HSCT) (1,2). It is rapidly hydrolyzed to mycophenolic acid (MPA) in vivo by esterase in the blood, gut wall, liver and tissues, and excreted as the glucuronide conjugate (MPA 7-O-glucuronide, MPAG) in urine and bile (3). MPAG excreted in the bile can be converted back to MPA by endogenous and bacterial (gastrointestinal flora) β-glucuronidase (4,5) in a process termed enterohepatic recycling (EHR). Interruption of EHR leads to a decrease in the area under the total plasma concentration time curve (AUC) of MPA by ≈40% (6).
Pharmacokinetic studies of MPA in HSCT recipients have shown considerable interindividual variability (1,3,7). HSCT patients frequently experience more drug-drug interactions, hypoalbuminemia and gastrointestinal disturbances, all of which can affect the MPA concentration. Unlike solid organ transplant patients, HSCT recipients have more opportunistic infections, resulting in a substantially higher probability of the use of antibiotics. It is known that antibiotic has visible drug–drug interactions with MPA. While it has been reported that the concentration of MPA can be influenced by oral antibiotics via changes the intestinal glucuronidase activity (8-11), very little information is available on the interactions between intravenous (IV) antibiotics and MPA.
MPA has a narrow therapeutic index and EHR plays an essential role in maintaining MPA plasma concentrations (7). The variability in EHR may lead to unpredictable immunosuppression, which may adversely affect engraftment, GVHD protection, and MMF toxicity. Recently, the strategy of gut decontamination with antibiotics such as rifaximin has been implemented routinely in patients undergoing HSCT. Although rifaximin is reported to maintain greater intestinal microbiome diversity (12-15), it may affect the EHR of MPA. In addition, cyclosporine, an immunosuppressant commonly used in combination with MMF, can also significantly interrupt the EHR of MPA (6,7). In the literature, the influence of oral antibiotics on MPA concentration is largely dependent on the existence of EHR. Therefore, it is difficult to predict whether antibiotics will cause a decrease in the MPA plasma concentration in patients whose EHR of MPA is already affected by cyclosporine and gut decontamination.
Carbapenem antibiotics (CBP) are broad-spectrum antibiotics. In clinical practice, IV CBP are widely used in HSCT recipients to treat infections caused by Gram-positive, Gram-negative and anaerobic bacteria. We hypothesized that IV CBP would affect the drug disposition of MPA. Therefore, we conducted a retrospective study to evaluate the extent of variation in the MPA pre-dose plasma concentration (C0) during CBP treatment in combination with cyclosporine and rifaximin in Chinese HSCT patients, to investigate whether IV CBP do lead to a decrease in the MPA C0 when EHR is already affected. We present the following article in accordance with the STROBE reporting checklist (available at https://atm.amegroups.com/article/view/10.21037/atm-22-6341/rc).
Methods
Study design
This single-center, retrospective cohort study evaluated HSCT patients who received IV MMF and CBP concomitantly from July 2018 to September 2021 at Institute of Hematology & Blood Diseases Hospital.
First, the changes in the MPA C0 before and during CBP use were evaluated in the whole study cohort. We aimed to detect an assumed reduction of 30% in MPA C0 before and during CBP use, based on previous studies of the renal or liver transplants (10,11). Sample size calculations determined that at least 41 subjects were required in a paired t test to provide an 80% power at a significance level of 5% (two-tailed). Considering the larger interindividual variation of HSCT patients, this study included as many subjects as possible.
The patients were studied as two groups when analyzing the impact of gut decontamination. One group comprised the patients who received rifaximin for gut decontamination, the other group comprised the patients who did not receive gut decontamination.
In order to eliminate the interference of other factors on the analysis results, the influence of related clinical factors was also estimated. The variables were selected based on scientific interest or prior knowledge of any possible relationship with MPA concentration.
Study population
The study was conducted in accordance with the Declaration of Helsinki (as revised in 2013). The study was approved by Ethics Committee of Blood Diseases Hospital, Chinese Academy of Medical Sciences (No. IIT2021011-EC-1) and individual consent for this retrospective analysis was waived. Study exclusion criteria included: (I) patients who lacked measurement of their MPA C0 before or during CBP use; (II) patients who switched from IV to oral MMF during the study period; (III) combination therapy of rifampin with MMF; (IV) the immunosuppressive regimen, and the use of rifaximin and proton pump inhibitors did not meet the requirements (eligibility criteria are described in the ‘GVHD prophylaxis and other treatment’ section). In addition, the MPA C0 during diarrhea (watery stools or daily defecation times >3) and during the use of montmorillonite powder was not collected for the analysis, to avoid the confounding effect of diarrhea on the MPA C0.
Blood concentration monitoring
The MPA C0 was determined weekly by an enzyme multiplied immunoassay technique (EMIT, Viva-ETM, Siemens, Germany). Blood samples were collected prior to administration of the morning medications, and at least 4 days after MMF initiation to avoid analysis of samples taken before steady-state conditions. Samples for the MPA assay were drawn through indwelling catheters not used for infusion of MMF and were placed in EDTA-K2 anticoagulant tubes.
The calibration curve for the Viva-E instrument was linear over a range of 0.1–15 µg/mL. The accuracy of the monitoring results was determined by comparing the observed concentration with the nominal concentration of the quality control samples at three concentration levels. The accuracy deviation values were all within 100±15% of the actual values at each quality control level.
Data collection
Patients’ information was followed up during the sample collection period. The follow-up information was obtained from the medical record and included liver/renal function, demographic information, albumin (ALB)/cyclosporine concentration, MPA C0 and medication information.
For each patient, the MPA C0 was divided into two separate time periods according to the administration of CBP. In the period without concomitant use of CBP, MPA steady-state samples were collected and the mean of C0 was calculated, and this acted as the patient’s own control. Once CBP therapy was initiated, the mean of C0 was calculated from subsequently recorded MPA blood samples and used to measure the influence of CBP.
The indexes of liver and renal function were measured at least twice weekly during weeks 1–4 post-transplant, and once weekly after 4 weeks for two months. During the sample collection period, the normal ranges of serum creatinine and aminotransferases were variable. Consequently, liver and renal functions were compared using the ratio of measured value to the corresponding upper limit of normal as the index (the ratio of measured creatinine value to the upper limit of normal, Cr-R; the ratio of measured alanine transaminase value to the upper limit of normal, ALT-R; the ratio of measured aspartate transaminase value to the upper limit of normal, AST-R).
GVHD prophylaxis and other treatment
The day of HSCT was designated day 0. Patients received MMF, cyclosporine and methotrexate as a triple immunosuppressive regimen for GVHD prophylaxis. The patients in our study were administered lyophilized powder of MMF for injection (Cellcept®, Roche Pharmaceuticals Ltd., Rochester, MI, USA, 0.5 g; Jianlin®, Shuanghe Pharmaceuticals Ltd., Hainan, China, 0.5 g) at a fixed dose of 500 mg every 12 h from day –9. IV cyclosporine was adjusted to maintain therapeutic levels of 150–350 ng/mL early after transplantation, and then administered orally when tolerated. Methotrexate at a dose of 15 mg/m2 was given intravenously on day 1, followed by 10 mg/m2 on days 3, 6 and 11.
Every patient received meropenem or imipenem as infection treatment, typically 1 g every 8 h, except for two patients who received meropenem 500 mg every 8 h or imipenem 500 mg every 6 h. Rifaximin 0.4 g twice daily was administered for gut decontamination, starting before CBP and MMF, and then continuously during MMF sampling. All patients received proton pump inhibitors across all data collection periods. In addition, all patients received antifungal and antiviral prophylaxis in accordance with standard practice at the institution.
Statistical analysis
All analysis were performed using SPSS software (Chicago, IL, USA). Data are presented as mean ± standard deviation (s.d.). Each continuous variable was assessed for normality using the Shapiro-Wilk test. The changes in the MPA C0 before and during CBP use were evaluated by the 2-tailed paired t test. Linear regression models were used to examine associations between potential variables and the MPA C0. First, the relationship between the dose-normalized MPA C0 [(µg/mL)/(mg/kg)] and CBP dose per body weight (mg/kg) was evaluated, and second, the individual effect of nine variables (Table 1) on MPA levels was examined by univariate analysis. Finally, a joint examination of the explanatory factors by multivariate analysis was made, checking for collinearity. If there are collinearity independent variables, the less important one will be removed, and then the multiple linear regression model will be developed again. Coefficient was calculated for gut decontamination with further adjustment of sex, age, weight, blood concentration of cyclosporine, ALB, liver and renal functions from multivariate regression. These tests were two-tailed, and a P value at least below 0.05 was assumed to represent statistical significance.
Table 1
Factors | Before CBP use | During CBP use | |||||
---|---|---|---|---|---|---|---|
Coefficient | 95% CI | P value | Coefficient | 95% CI | P value | ||
Univariate analysis | |||||||
Use of rifaximin | −0.049 | −0.049 (−0.259 to 0.161) | 0.641 | 0.11 | 0.110 (−0.080 to 0.301) | 0.249 | |
Male vs. female | −0.073 | −0.073 (−0.263 to 0.118) | 0.118 | 0.086 | 0.086 (−0.088 to 0.260) | 0.325 | |
Age | 0.002 | 0.002 (−0.004 to 0.009) | 0.506 | 0.003 | 0.003 (−0.003 to 0.009) | 0.288 | |
Weight | 0.002 | 0.002 (−0.005 to 0.009) | 0.599 | 0.004 | 0.004 (−0.002 to 0.011) | 0.211 | |
ALB | −0.001 | −0.001 (−0.028 to 0.026) | 0.943 | 0.007 | 0.007 (−0.021 to 0.036) | 0.609 | |
Cyclosporine | 0 | 0 (−0.001 to 0.001) | 0.647 | -0.001 | −0.001 (−0.002 to 0) | 0.112 | |
Cr-R | 0.765 | 0.765 (−0.157 to 1.686) | 0.102 | 0.501 | 0.501 (−0.506 to 1.507) | 0.323 | |
ALT-R | −0.186 | −0.186 (−0.393 to 0.022) | 0.078 | -0.046 | −0.046 (−0.206 to 0.114) | 0.567 | |
AST-R | −0.113 | −0.113 (−0.483 to 0.256) | 0.540 | -0.021 | −0.021 (−0.320 to 0.278) | 0.887 | |
Multivariate analysis | |||||||
Use of rifaximin† | −0.044 | −0.044 (−0.271 to 0.183) | 0.696 | 0.04 | 0.040 (−0.169 to 0.249) | 0.700 |
†, coefficient from the reported multivariate regression is for gut decontamination with rifaximin with further adjustment of sex, age, weight, blood concentration of cyclosporine, ALB, liver and renal functions. MPA, mycophenolic acid; C0, pre-dose concentration; CBP, carbapenem antibiotics; CI, confidence interval; ALB, albumin; Cr-R, the ratio of measured creatinine value to the upper limit of normal; ALT-R, the ratio of measured alanine transaminase value to the upper limit of normal; AST-R, the ratio of measured aspartate transaminase value to the upper limit of normal.
Results
Patients’ characteristics
From July 2018 to September 2021, 52 patients fulfilled the criteria of this retrospective study and were included in the analysis; of them, 38 were administered rifaximin for gut decontamination. Among all patients, the median number of days to starting CBP infusion was day 0 (range, –7 to 7). A total of 57 routinely monitored MPA C0 measurements were collected before CBP treatment. In addition, 76 samples for MPA C0 were obtained at a median of day 7 (range, 1–19) after CBP initiation. For each patient, the mean MPA C0 from multiple measurements was calculated for each time period separately and used for the analysis.
The patients’ characteristics are summarized in Table 2. The median age and weight were 34.5 (range, 9–56) years and 61.50 (range, 36.60–97.36) kg, respectively. Most of the patients underwent HSCT for the treatment of hematologic malignancies, with the exception of 4 patients who had aplastic anemia, 1 patient who had Fanconi anemia and 1 patient who had paroxysmal nocturnal hemoglobinuria. Transplants were from a family HLA-haploidentical donor for 51 patients and a 10/10 HLA-matched unrelated donor for 1 patient. During the course of therapy, serum creatinine did not increase abnormally in any of the 52 patients, and the overall median Cr-R was 0.49 (range, 0.30–0.85). Aminotransferase levels more than 1.5-fold of the upper limit of normal only occurred in 5 patients. The median ALT-R and AST-R were 0.34 (range, 0.07–3.01) and 0.31 (range, 0.13–1.52), respectively.
Table 2
Characteristic | N | % |
---|---|---|
Sex | ||
Male | 32 | 61.54 |
Female | 20 | 38.46 |
Age, years | ||
≤18 | 10 | 19.23 |
19–29 | 10 | 19.23 |
30–39 | 10 | 19.23 |
40–49 | 12 | 23.08 |
50–59 | 10 | 19.23 |
Weight, kg | ||
≤70 | 38 | 73.08 |
>70 | 14 | 26.92 |
Disease | ||
ALL | 16 | 30.77 |
AML | 16 | 30.77 |
MDS | 9 | 17.31 |
AA | 4 | 7.69 |
Other | 7 | 13.46 |
Carbapenem antibiotic | ||
Meropenem | 46 | 88.46 |
Imipenem | 6 | 11.54 |
ALL, acute lymphoblastic leukemia; AML, acute myelogenous leukemia; MDS, myelodysplastic syndrome; AA, aplastic anemia.
MPA C0 before and during CBP use
Figure 1 shows the effect of concomitant administration of CBP on the MPA C0. There were wide inter- and intra-individual variations of the MPA C0 after IV administration in these Chinese HSCT patients.
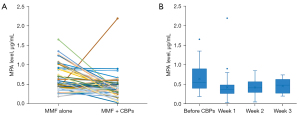
Intra-individual comparison of the MPA C0 revealed an obvious decrease in the MPA C0 after co-administration with CBP when compared with MMF alone (Figure 1A). In the whole study cohort, the average MPA C0 before CBP use was 0.65±0.33 µg/mL, and during the course of CBP therapy it was 0.43±0.30 µg/mL, which represented a decrease of 16.06%±78.54% (Table 3). Compared with the pre-CBP period, 69.23% (36 of 52) patients had a reduction in their MPA C0 during CBP use. MPA C0 <1 µg/mL was found in 80.70% patients before CBP use and in 98.68% patients during CBP use. The two-tailed paired t test indicated that the MPA C0 changed significantly as a result of CBP treatment (P<0.05; Table 3).
Table 3
Group | Concentration (± s.d.) μg/mL | P value | Concentration change, % | |
---|---|---|---|---|
Before CBP use | During CBP use | |||
Gut decontamination group (N=38) | 0.64 (0.35) | 0.46 (0.33)* | 0.034 | 6.60 (87.84) |
Non-gut decontamination group (N=14) | 0.69 (0.30) | 0.35 (0.22)* | 0.003 | 41.73 (35.60) |
Total (N=52) | 0.65 (0.33) | 0.43 (0.30) * | 0.001 | 16.06 (78.54) |
*, P value, <0.05 by two-tailed paired t test, compared with MPA C0 before the use of CBP. CBP, carbapenem antibiotics; s.d., standard deviation; MPA, mycophenolic acid; C0, pre-dose concentration.
The results also showed that the maximum-state of the interaction between MMF and CBP was achieved by the first week after CBP initiation. The MPA C0 decreased obviously during the first week after CBP administration and subsequently stabilized (Figure 1B). The mean weekly MPA C0 at weeks 1, 2 and 3 after CBP use was 0.42 (range, 0.03–2.19; sample number: 46), 0.42 (range, 0.05–0.85; sample number: 25) and 0.46 (range, 0.2–0.74; sample number: 5) µg/mL, respectively. Boxplots show the 25th and 75th percentile (upper and lower boundaries of box), median (solid lines in box), mean (× symbols), and distribution of individual concentrations (circles).
To further illustrate the influence of CBP, we then calculated the correlations between the dose-normalized MPA C0 [(µg/mL)/(mg/kg)] and the CBP dose per body weight (mg/kg). As shown in Figure 2, there was a significant linear correlation between these parameters (r2=0.129, P=0.009). The dose-normalized MPA C0 decreased close to a linearity relation as the CBP dosage per body weight increased.
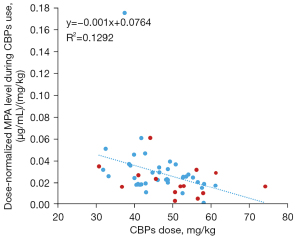
Univariate analysis of factors related to MPA C0
Potential influences associated with the MPA C0 in patients undergoing HSCT were analyzed (Table 1). Two analysis periods were prepared separately to include the time before and during CBP use. Univariate analysis indicated that many factors, including age, sex, weight, ALB levels, liver and renal function, the use of rifaximin, and cyclosporine levels did not affect the MPA C0 in either time period (P>0.05, Table 1).
Comparison of MPA C0 between non-gut decontamination and gut decontamination groups
After administration of CBP, the mean C0 of MPA decreased significantly in both the non-gut decontamination and gut decontamination group (P<0.05, Table 3). Inclusion of 14 subjects in the paired t test analysis of the non-gut decontamination group still detected a 0.34 µg/mL mean reduction in the MPA C0 before and during CBP use with α=0.05 and 1-β=0.95. In the gut decontamination group, the statistical power (1-β) was 0.62 for the MPA C0 in the paired t test because of the relatively small mean reduction (0.18 µg/mL) and large variation.
Multivariate analysis also suggested that rifaximin did not significantly affect patients’ MPA C0 either before or during CBP treatment periods (P>0.05; Table 1). Nevertheless, 92.86% (13 of 14) patients in the non-gut decontamination group had a reduction in their MPA C0 during CBP use, which was higher than the proportion of the gut decontamination group (60.53%, 23 of 38). We noticed that compared with the non-gut decontamination group, smaller decreases in the MPA C0 during CBP therapy were seen in the gut decontamination group (Table 3). Meanwhile, there was considerable interindividual variability in terms of the magnitude of the decrease. Among the patients who received rifaximin, the variations in the reduction of the MPA C0 ranged from −447.50% to 92.68% (mean: 6.60%). Non-gut decontamination recipients experienced corresponding variations ranging from −13.89% to 92.67% (mean: 41.73%).
Discussion
Decreases in the MPA concentration caused by administration of oral antibiotics has been reported in renal or liver transplant recipients (10,11). However, HSCT patients have many differences from those undergoing solid organ transplantation (16,17). We believe we are the first to study the effect of IV antibiotics on MPA C0 in the HSCT patients when EHR of MPA is interrupted.
Our research suggested that a significant decrease in the MPA C0 occurs in HSCT patients undergoing concomitant MMF, cyclosporine and CBP treatment, irrespective of gut decontamination. Rifaximin and CBP did not have an additive effect. On the contrary, of the 2 groups of regimens, CBP seemed to be more potent in reducing the MPA C0 when not combined with rifaximin (mean reduction: 0.34 µg/mL vs. 0.18 µg/mL), although the univariate and multivariate analysis confirmed that the difference was not statistically significant. On the other hand, interindividual variabilities in the MPA C0 were larger in the gut decontamination group than in the non-gut decontamination group, which suggested that the administration of rifaximin may lead to variation of the MPA C0.
The exact pharmacological mechanism of the interactions between MMF (or MPA) and antibiotics remains unclear. The most-described explanation is a reduction in the deconjugation of MPAG by intestinal flora-mediated inhibition of β-glucuronidase, reducing the EHR of MPA (7,9-11). However, all patients in this study received cyclosporine, which can inhibit the multidrug resistance protein-2 (MRP-2) and thus substantially interrupt EHR (6,7), weakening the ability of the gastrointestinal flora to transform MPAG to MPA. Furthermore, our study confirmed that CBP can still lead to a decreased MPA C0 even if patients received rifaximin for gut decontamination. Given these data, it is unlikely that CBP substantially reduce the EHR of MPA through suppression of intestinal deconjugation of MPAG. On the other hand, MPA is highly bound to ALB (97–99%) in plasma (7). With plasma protein binding of meropenem or imipenem at <20% (18,19), such an interaction is unlikely to occur.
MPA is mainly metabolized by various members of the UDP-glucuronosyltransferase (UGT) family in the gastrointestinal tract, liver and kidney into the main metabolite MPAG; among them, UGT1A9 accounts for >50% of the biotransformation (3,7,20). After glucuronidation, most MPAG is excreted from the kidney (87%). Thus, there are another two possible causes of a decreased MPA C0: increased conjugation or decreased deconjugation mediated by UGT and endogenous β-glucuronidase. Yamamura et al. found that CBP increased the level of UDP glucuronic acid in rats (21). UDP glucuronic acid is a co-factor for UGT-mediated glucuronidation. Therefore, an increased level of UDP glucuronic acid may influence the pharmacokinetics of MPA. Second, Mori et al. confirmed the direct activation of UGT by CBP (22). This activation was observed after pre-incubation of human liver microsomes with CBP. Third, Nakamura et al. found that CBP can directly inhibit the activity of β-glucuronidase in human liver microsomes (23). Although these studies were not designed to elucidate the effects of CBP on MPA metabolism, they provide directions for finding the possible mechanisms. However, more examinations are required to show the significance of these findings.
The rifaximin plasma concentration and exposure are low and variable because of limited systemic absorption after oral administration (24). Based on our findings, we speculate that the amount of rifaximin entering the systemic circulation can affect the pharmacokinetic behavior of MPA. For example, both MPA and rifaximin are known substrates for P-glycoprotein, and rifaximin may inhibit P-glycoprotein-mediated transport (7,24,25). However, it is possible that the influence of rifaximin was too slight to be demonstrated in our small study group with considerable intra-individual variation in MPA concentrations.
We also cannot ignore that factors other than the use of antibiotics may be involved in the decreased plasma MPA concentrations. Of note, demographics, fluctuation in the plasma ALB and cyclosporine concentrations, and liver and renal functions did not affect the MPA C0 in this study. Diarrhea may affect it (26), so patients with watery stools or daily defecation more than three times were excluded from our analysis. Surprisingly, most patients had a MPA C0 <1 µg/mL, which was significantly lower than the therapeutic range for MPA C0 recommended by guidelines (1–3.5 µg/mL) (7).
There are limitations to the present study that should be highlighted. First, a relatively small number of patients were examined; a larger sample size would enhance the robustness of our findings. Second, our results may not extend to patients receiving tacrolimus rather than cyclosporine, considering the median MPA clearance was 33% higher in patients receiving concomitant cyclosporine compared with patients receiving tacrolimus (27). It is unclear if the extent of CBP influence is similar when MMF is combined with various immunosuppressants. Third, poor correlation has generally been reported between the MPA C0 and the AUC in HSCT patients, and therefore the changes in MPA C0 seen in this study may not represent proportional changes in the AUC (7). In fact, full pharmacokinetic profiles will promote better understanding of the interactions between CBP and MMF in patients undergoing HSCT.
Nonetheless, the findings of this research have potential clinical implications. Clinicians should be aware that IV CBP may reduce the MPA C0, even when MMF is used in combination with cyclosporine and rifaximin. If antibiotics must be used, and CBP in particular, therapeutic drug monitoring should be performed to ensure adequate exposure.
Conclusions
Concomitant CBP treatment with MMF therapy in HSCT patients when EHR was interrupted still resulted in a significant reduction in the MPA C0. Univariate and multivariate analysis confirmed that the MPA C0 had no relation to the use of rifaximin, patient demographics, fluctuation in plasma ALB and cyclosporine concentrations, or liver and renal functions. However, compared with a non-gut decontamination group, larger interindividual variability and smaller decreases in the MPA C0 during CBP therapy were seen in the gut decontamination group, although this was a nonsignificant trend. This study is the first to evaluate the effect of IV antibiotics on the MPA C0 in HSCT patients when the EHR of MPA is interrupted.
Acknowledgments
The authors thank the pharmacists of the Institute of Hematology and Blood Diseases Hospital who assisted with data collection.
Funding: None.
Footnote
Reporting Checklist: The authors have completed the STROBE reporting checklist. Available at https://atm.amegroups.com/article/view/10.21037/atm-22-6341/rc
Data Sharing Statement: Available at https://atm.amegroups.com/article/view/10.21037/atm-22-6341/dss
Conflicts of Interest: All authors have completed the ICMJE uniform disclosure form (available at https://atm.amegroups.com/article/view/10.21037/atm-22-6341/coif). The authors have no conflicts of interest to declare.
Ethical Statement: The authors are accountable for all aspects of the work in ensuring that questions related to the accuracy or integrity of any part of the work are appropriately investigated and resolved. The study was conducted in accordance with the Declaration of Helsinki (as revised in 2013). The study was approved by Ethics Committee of Blood Diseases Hospital, Chinese Academy of Medical Sciences (No. IIT2021011-EC-1) and individual consent for this retrospective analysis was waived.
Open Access Statement: This is an Open Access article distributed in accordance with the Creative Commons Attribution-NonCommercial-NoDerivs 4.0 International License (CC BY-NC-ND 4.0), which permits the non-commercial replication and distribution of the article with the strict proviso that no changes or edits are made and the original work is properly cited (including links to both the formal publication through the relevant DOI and the license). See: https://creativecommons.org/licenses/by-nc-nd/4.0/.
References
- Nakane T, Nakamae H, Yamaguchi T, et al. Use of mycophenolate mofetil and a calcineurin inhibitor in allogeneic hematopoietic stem-cell transplantation from HLA-matched siblings or unrelated volunteer donors: Japanese multicenter phase II trials. Int J Hematol 2017;105:485-96. [Crossref] [PubMed]
- Paviglianiti A, Labopin M, Blaise D, et al. Comparison of mycophenolate mofetil and calcineurin inhibitor versus calcineurin inhibitor-based graft-versus-host-disease prophylaxis for matched unrelated donor transplant in acute myeloid leukemia. A study from the ALWP of the EBMT. Bone Marrow Transplant 2021;56:1077-85. [Crossref] [PubMed]
- McCune JS, Bemer MJ, Long-Boyle J. Pharmacokinetics, Pharmacodynamics, and Pharmacogenomics of Immunosuppressants in Allogeneic Hematopoietic Cell Transplantation: Part II. Clin Pharmacokinet 2016;55:551-93. [Crossref] [PubMed]
- Sperker B, Backman JT, Kroemer HK. The role of beta-glucuronidase in drug disposition and drug targeting in humans. Clin Pharmacokinet 1997;33:18-31. [Crossref] [PubMed]
- Simpson JB, Sekela JJ, Graboski AL, et al. Metagenomics combined with activity-based proteomics point to gut bacterial enzymes that reactivate mycophenolate. Gut Microbes 2022;14:2107289. [Crossref] [PubMed]
- van Gelder T. How cyclosporine reduces mycophenolic acid exposure by 40% while other calcineurin inhibitors do not. Kidney Int 2021;100:1185-9. [Crossref] [PubMed]
- Bergan S, Brunet M, Hesselink DA, et al. Personalized Therapy for Mycophenolate: Consensus Report by the International Association of Therapeutic Drug Monitoring and Clinical Toxicology. Ther Drug Monit 2021;43:150-200. [Crossref] [PubMed]
- Naderer OJ, Dupuis RE, Wiwattanawongsa K, et al. Reduction of plasma mycophenolic acid (MPA) and its glucuronide (MPAG) concentrations with antibiotic treatment. Clinical Pharmacology & Therapeutics 1999;65:159. [Crossref]
- Naderer OJ, Dupuis RE, Heinzen EL, et al. The influence of norfloxacin and metronidazole on the disposition of mycophenolate mofetil. J Clin Pharmacol 2005;45:219-26. [Crossref] [PubMed]
- Schmidt LE, Rasmussen A, Nørrelykke MR, et al. The effect of selective bowel decontamination on the pharmacokinetics of mycophenolate mofetil in liver transplant recipients. Liver Transpl 2001;7:739-42. [Crossref] [PubMed]
- Borrows R, Chusney G, Loucaidou M, et al. The magnitude and time course of changes in mycophenolic acid 12-hour predose levels during antibiotic therapy in mycophenolate mofetil-based renal transplantation. Ther Drug Monit 2007;29:122-6. [Crossref] [PubMed]
- Weber D, Oefner PJ, Dettmer K, et al. Rifaximin preserves intestinal microbiota balance in patients undergoing allogeneic stem cell transplantation. Bone Marrow Transplant 2016;51:1087-92. [Crossref] [PubMed]
- Gillis JC, Brogden RN. Rifaximin. A review of its antibacterial activity, pharmacokinetic properties and therapeutic potential in conditions mediated by gastrointestinal bacteria. Drugs 1995;49:467-84. [Crossref] [PubMed]
- Weber D, Hiergeist A, Weber M, et al. Detrimental Effect of Broad-spectrum Antibiotics on Intestinal Microbiome Diversity in Patients After Allogeneic Stem Cell Transplantation: Lack of Commensal Sparing Antibiotics. Clin Infect Dis 2019;68:1303-10. [Crossref] [PubMed]
- Jenq RR, van den Brink MR. Antibiotic prophylaxis in allogeneic stem cell transplantation-what is the correct choice? Bone Marrow Transplant 2016;51:1071-2. [Crossref] [PubMed]
- Khan MH, Onyeaghala GC, Rashidi A, et al. Fecal β-glucuronidase activity differs between hematopoietic cell and kidney transplantation and a possible mechanism for disparate dose requirements. Gut Microbes 2022;14:2108279. [Crossref] [PubMed]
- Saqr A, Carlson B, Staley C, et al. Reduced Enterohepatic Recirculation of Mycophenolate and Lower Blood Concentrations Are Associated with the Stool Bacterial Microbiome after Hematopoietic Cell Transplantation. Transplant Cell Ther 2022;28:372.e1-9. [Crossref] [PubMed]
- Baldwin CM, Lyseng-Williamson KA, Keam SJ. Meropenem: a review of its use in the treatment of serious bacterial infections. Drugs 2008;68:803-38. [Crossref] [PubMed]
- Couffignal C, Pajot O, Laouénan C, et al. Population pharmacokinetics of imipenem in critically ill patients with suspected ventilator-associated pneumonia and evaluation of dosage regimens. Br J Clin Pharmacol 2014;78:1022-34. [Crossref] [PubMed]
- Bowalgaha K, Miners JO. The glucuronidation of mycophenolic acid by human liver, kidney and jejunum microsomes. Br J Clin Pharmacol 2001;52:605-9. [Crossref] [PubMed]
- Yamamura N, Imura-Miyoshi K, Naganuma H. Panipenum, a carbapenem antibiotic, increases the level of hepatic UDP-glucuronic acid in rats. Drug Metab Dispos 2000;28:1484-6. [PubMed]
- Mori H, Takahashi K, Mizutani T. Interaction between valproic acid and carbapenem antibiotics. Drug Metab Rev 2007;39:647-57. [Crossref] [PubMed]
- Nakamura Y, Nakahira K, Mizutani T. Decreased valproate level caused by VPA-glucuronidase inhibition by carbapenem antibiotics. Drug Metab Lett 2008;2:280-5. [Crossref] [PubMed]
- Rivkin A, Gim S. Rifaximin: new therapeutic indication and future directions. Clin Ther 2011;33:812-27. [Crossref] [PubMed]
- Xifaxan (rifaximin) tablets [package insert]. Morrisville, NC: Salix Pharmaceuticals; 2014.
- Tanaka R, Matsumoto A, Tatsuta R, et al. Sustained suppression of enterohepatic circulation of mycophenolic acid by antimicrobial-associated diarrhea in a kidney transplant recipient with Crohn's disease: A case report. Clin Case Rep 2022;10:e05914. [Crossref] [PubMed]
- Li H, Mager DE, Sandmaier BM, et al. Population pharmacokinetics and dose optimization of mycophenolic acid in HCT recipients receiving oral mycophenolate mofetil. J Clin Pharmacol 2013;53:393-402. [Crossref] [PubMed]
(English Language Editor: K. Brown)