Comprehensive analysis of immune-related genes associated with the microenvironment of patients with unexplained infertility
Highlight box
Key findings
• We studied the immune microenvironment of unexplained infertility and identified key immune genes associated with microenvironment disorders.
What is known and what is new?
• In recent years, researchers have found that the changes of immune cells and immune factors could break maternal immune tolerance and lead to infertility, especially in unexplained infertility patients.
• In this study, CD40, PRF1 and EDN3 were found to affect the immune microenvironment of infertile patients through multiple biological processes and molecular functions.
What is the implication, and what should change now?
• In the future, these three genes are expected to become new therapeutic targets and may guide the immunotherapy of unexplained infertility patients.
Introduction
Infertility is a subject of worldwide concern, affecting 10–15% of couples of childbearing age (1). Infertility is defined as the inability to conceive after at least 12 months of unprotected sex (2). The etiology of infertility can be broken down into 4 main groups including male factors, female factors, combined male and female factors, or unexplained causes (3). Infertility patients with the absence of definite causes are classified as unexplained infertility (UEI). The diagnosis of UEI requires the presence of a normal tubal patency test (at least one tubal patency), regular ovulatory cycles, and no abnormality in spouse semen analysis (4). After a standard screening, approximately 30% of couples experiencing infertility are diagnosed with UEI (4). Clinical practitioners are aware of the limitations of this diagnostic assessment. A number of factors may contribute to infertility, including age, quality of the oocytes, cervical mucus, genetics, immune factors, fertilization failure, and others. It is not possible to exclude all the above reasons using sensitive and specific examination methods, so they come into play as potential factors in UEI. In this case, intervention measures are frequently taken without determining the causes of infertility, which can lead to frustration for both patients and clinicians. In vitro fertilization (IVF) has also been employed to treat UEI, but among UEI patients aged ≤40 years, the live birth rate of IVF per cycle was between 25% and 43% (5).
There is a growing bulk of evidence that aberrant alterations in the balance of immunosuppressive arms or immune inflammatory factors are associated with various types of infertility (6-8). Pregnancy involves immune cells, immunoglobulins, cytokines, and other factors, and the coordination of these factors is critical for successful implantation and conception. Shakerian et al. revealed a significant drop in the percentage of CD8 T cells and CD56 NK cells in infertile patients compared to the controls, indicating that adaptive immunity plays a vital role in the etiology of infertility (9). Regulatory T cells (Tregs) inhibited effector immunity, mediated maternal immune tolerance and supported maternal vascular adaptations. Inadequate functional competence or insufficient Treg numbers are related to infertility (10). Bai et al. reported that the presence of such antibodies as antiendometrium (AEmAb), antisperm (AsAb), antiovary (AoAb), and antitrophoblast (ATB) may affect the fertilization process, resulting in infertility (11). Brazdova et al. showed that lower expression of Foxp3, failure of lymphocyte chemotaxis and adherence, and diminished CD4+CD25+ Treg population seemed to be precursors of infertility (12). In recent years, researchers have paid attention to immune cells and immune factors and found that their changes could break maternal immune tolerance and lead to infertility, especially UEI. For example, Ehsani et al. suggested that CD8+ Human leukocyte antigen (HLA)-G+ regulatory T cell, a distinct subset of regulatory T cells, is involved in the pathogenesis of UEI (13). Madendag et al. found that secretory leukocyte protease inhibitor (SLPI) levels in patients with UEI are significantly elevated, which triggers an immune response that may be one of the causes of UEI (14). A study even showed that the immune system plays an important role in pregnancy outcome, and immune imbalance is considered to be the main cause of 50% UEI (15).
Despite recent advancements in assisted reproductive technology (ART) and other treatments, some patients still experience intractable infertility. Therefore, it is imperative to better understand disease mechanisms and to search for biomarkers to assess infertility risks and predict pregnancy outcome. Multiple factors can cause infertility. Some studies focus on infertility with a clear etiology. According to Lőrincz et al., polypectomy can boost the fertility rate in the following year in infertile women regardless of the surgical resection methods (16). Using multivariate logistic regression analysis, Adedigba et al. found that women who did not have hydrosalpinx were less likely to be infertile than those who did. Immune dysfunction may be a part of the pathogenesis (17). Thus, some researchers attempt to identify immune biomarkers for guidance on clinical practice in order to optimize the chances of successful pregnancy by reversing the imbalance in immune players. For instance, Huang et al. analyzed the differential expression and immune infiltration of endometriosis (EMS) related genes and provided a framework for developing molecular networks related to EMS pathology (18). However, there is still little clear evidence about how immune-related gene (IRG) expression and immune landscapes are altered in patients with UEI. So far, UEI is still a challenging “diagnosis” in reproductive medicine. In addition, after taking various treatment measures, the pregnancy outcome is not satisfactory. Therefore, it is urgent to better explore the underlying etiology and disease mechanism of infertility. It is important in reproductive medicine to clarify the immune mechanism of the endometrium, leading to the success of implantation and pregnancy. Identifying immune checkpoints responsible for immune imbalances in women who suffer from UEI was the purpose of this study, which examined infiltrating immune cells and immune-associated genes in the microenvironment of UEI. The results of this study provided novel and reliable biomarkers for UEI patients, which may aid in personalizing treatments and promoting a successful pregnancy. We present the following article in accordance with the MDAR reporting checklist (available at https://atm.amegroups.com/article/view/10.21037/atm-22-5810/rc).
Methods
Patients
The endometrial tissues of infertile and pregnant women under 40 years of age were obtained from The First Hospital of Lanzhou University between September 2021 and September 2022. The inclusion criteria for studies were: (I) embryo transfer ≥2 times; (II) normal uterine cavity; (III) normal ovulation; (IV) at least one patent fallopian tube; (V)male partner with normal semen analysis. Exclusion criteria included obesity, uterine malformation or adhesion, EMS, polycystic ovarian syndrome, chromosomal abnormalities, and autoimmune disorders. We collected 12 fresh endometrial tissue and 12 formalin-fixed paraffin-embedded tissue specimens from UEI patients. Additionally, 12 fresh tissue and 12 formalin-fixed paraffin-embedded samples were collected from the endometrium of pluripara women. The study was conducted in accordance with the Declaration of Helsinki (as revised in 2013). This study was conducted with approval by the Ethics Committee of The First Hospital of Lanzhou University (approval No. LDYYLL2022-246) and informed consent was taken from all the patients.
Data acquisition and identification
We used the keywords “infertility” to search on the Gene Expression Omnibus (GEO) database (https://www.ncbi.nlm.nih.gov/). All datasets were included according to the following criteria: (I) They used human endometrial tissue; (II) They had case-control groups (UEI and fertility); (III) They contained at least 9 samples. Finally, 3 gene expression profiles (GSE16532, GSE92324, and GSE120103) were downloaded. Specifically, GSE16532 contains gene expression data between 4 infertile samples and 5 fertile samples; GSE92324 contains gene expression data between 10 infertile cases and 8 normal cases; GSE120103 contains gene expression data between 9 infertile samples and 9 normal samples.
Identification of differentially expressed IRGs (DEIRGs)
In GSE16532, the genes with differential expression were analyzed by the GEO2R tool. Genes with |log FC| ≥1 and P<0.05 were selected as candidate genes for next step. The Immunology Database and Analysis Portal (ImmPort) is a biological information database for analysis in immunology. A list of immunologically relevant genes was downloaded from ImmPort on 27 December 2020. Overlapping genes among the immunologically relevant genes and DEGs were identified by Venn diagram (http://bioinformatics.psb.ugent.be/webtools/Venn/) for further analysis, and they were subsequently called DEIRGs.
Functional enrichment analysis of DEIRGs
Gene Ontology (GO) analysis was used to annotate DEIRGs. The results of GO analysis were displayed by 3 domains including biological processes (BP), cellular component (CC), and molecular functions (MF). The Kyoto Encyclopedia of Genes and Genomes (KEGG) analysis was applied for pathway enrichment analysis. Both the GO and KEGG analysis were performed using the “clusterProfiler” R package (The R Foundation for Statistical Computing, Vienna, Austria).
Protein-protein interaction (PPI) network and module analysis
The Search Tool for the Retrieval of Interacting Genes/Proteins (STRING; https://www.strin g-db.org) was used to explore interactions between DEIRGs. PPI with a confidence score ≥0.4 were reserved and further analyzed by Cytoscape software (https://cytoscape.org/). We used the application of molecular complex detection (MCODE) with default parameters in Cytoscape to analyze the hub clustering modules in PPI network. Moreover, hub genes were selected based on maximal clique centrality (MCC) and Degree.
Estimation of tumor-infiltrating immune cells
We used CIBERSORTx (https://cibersort.stanford.edu) to identify the relative percentage of immune infiltrating cells in the immune microenvironment of patients with GSE16532 dataset. The parameters were as follows: signature genes: LM22, batch correction mode: B-mode, and permutations: 1,000. We further applied Wilcoxon’s test to compare the infiltration rates of 22 types of immune cells between infertile and fertile samples. We also detected the correlation between the expression level of the hub genes and the immune cell fraction using Spearman correlation analysis. We screened genes related to immune cells for further research and called them candidate genes.
Gene set enrichment analysis (GSEA)
First, we verified the expression of newly identified candidate genes among the GSE92324 dataset. We choose genes that are statistically different. Then, GSEA was employed to identify the potential function of the genes. Hallmark gene sets (c5.go.v7.2.entrez.gmt/c2.cp.kegg.v7.2.entrez.gmt) were downloaded from Molecular Signatures Database (MSigDB). A P value <0.05 was considered statistically significant.
GSE120103 dataset validation
We also obtained the gene expression from GSE120103 dataset to validate our study results, including 3 IRGs. Moreover, we performed a receiver operating characteristic (ROC) analysis to assess the diagnostic value of 3 DEIRGs. Thus, the ROC curve was built and the area under the curve (AUC) was computed. The AUC value >0.5 was considered a good diagnostic value for infertility.
Western blot analysis
Western blot analysis was applied for determining the levels of CD40, EDN3, and PRF1. Primary antibodies were CD40 (1:1,000, 66965-1-Ig, Proteintech, Rosemont, Il, USA), EDN3 (1:1,000, ab197374, Abcam, Cambridge, UK), and PRF1 (1:1,000, ab256453, Abcam, Cambridge, UK). The tissues of patients were lysed in radioimmunoprecipitation assay (RIPA) buffer. Total tissue protein was extracted and its concentration was quantified. Then, the proteins were transferred onto a polyvinylidene fluoride (PVDF) membrane. We blocked the membrane with 5% nonfat milk powder for 1 hour and added the primary antibody. After that, we incubated the membrane with diluted secondary antibody for 1 hour. Finally, protein bands on membrane were visualized. Glyceraldehyde 3-phosphate dehydrogenase (GAPDH) was used as the endogenous control.
Immunohistochemistry (IHC)
IHC helps to reveal the distribution and expression of relative protein based on specific binding of antigens with antibodies. IHC was conducted to determine the CD40, EDN3, and PRF1 expression in endometrial tissue samples at the protein level of infertile and fertile patients. In brief, samples were prepared into paraffin sections, then deparaffinized and hydrated. They were immersed in sodium citrate buffer and heated at high temperature for antigen unmasking. After adding endogenous peroxidase blocker, the samples then blocked with goat serum. Slides were stained with primary antibodies (1:1,000) under 4 ℃ overnight, followed by an additional 30 minutes at room temperature of incubating by secondary antibodies. After adding 3,3’diaminobenzidine (DAB) staining solution and hematoxylin staining, the sections were sealed and observed under microscope. The stained specimens were independently interpreted by 3 senior pathologists. The staining results were judged by the percentage of cells stained and the intensity of the brown-yellow positive signal. The percentage of stained cells was multiplied by the staining intensity value as a total score. If the product of the 2 scores was greater than 4 points, it was suggested to be positive.
Statistical analysis
All statistical analyses were performed with R software (4.0.3; The R Foundation for Statistical Computing). Group comparisons were performed using independent t-tests or Wilcoxon’s test. Statistical tests were 2-sided and P values <0.05 were considered significant (19). The R packages used in this study included limma, psych, corrplot, pheatmap, ggplot2, ggrepel, ggpubr, reshape, SQLite, org.Hs.eg.db, clusterProfiler, ggnewscale, cowplot, pROC, and gridExtra.
Results
Identification of DEIRGs
In the present study, DEIRGs and their significant biological functions were identified based on GEO datasets through comprehensive bioinformatics analysis of infertile patients (Figure 1). A total of 1,328 DEGs were detected from GSE16532 (Figure 2A). The expression levels of 1,793 IRGs were examined among the previously mentioned 1,328 DEGs (Figure 2B). After analysis, 124 DEIRGs comprising 50 upregulated and 74 downregulated genes were obtained (Figure 2C) and considered according to the criteria of P<0.05 and |log FC| ≥1.
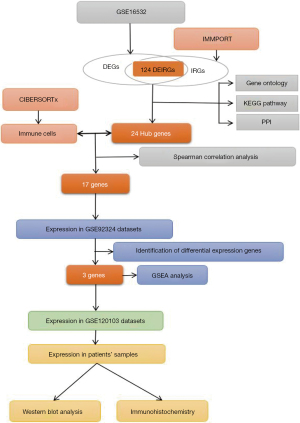
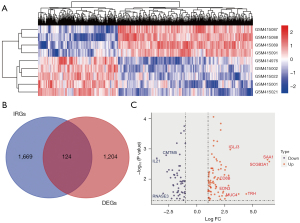
Functional enrichment analysis of DEIRGs
We performed GO and KEGG pathway enrichment analyses to elucidate the potential biological functions of 124 DEIRGs. The results are presented in Figure 3. The GO analysis revealed that the DEIRGs were mainly enriched in BP related to immune mechanism involving regulation of chemotaxis, T cell activation, positive regulation of ERK1 and ERK2 cascade,positive regulation of cytokine production, and leukocyte cell-cell adhesion (Figure 3A). In CC analysis, we found that many gene products were located in the external side of plasma membrane (Figure 3B). In MF analysis, we found that many DEIRGs were involved in receptor ligand activity, signaling receptor activator activity, immune receptor activity and cytokine activity (Figure 3C). The enrichment of MF supports BP. According to KEGG pathway enrichment analysis (Figure 3D), the 124 DEIRGs were primarily associated with antigen processing and presentation, cytokine-cytokine receptor interaction, and intestinal immune network for IgA production. In addition, we found that they played an important role in allograft rejection and graft-versus-host disease.
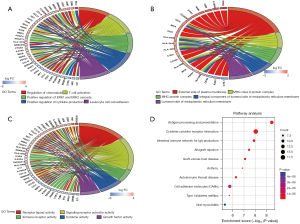
PPI network and module analysis
The relationships and functions of the DEIRGs are revealed using a PPI network consisting of 119 nodes and 380 interactions (Figure 4). Based on highest degree, the top 28 genes were selected. We also chose the 28 genes with the highest MCC scores. As a result, we identified the 24 overlapping genes as the target hub genes (Table 1). The MCODE plugin was applied to detect important modules in the PPI network, which yielded 6 modules. The abovementioned 24 genes were presented in 3 modules (Figure 5A-5C), implying that the modules could significantly represent the important biological features in the PPI network.
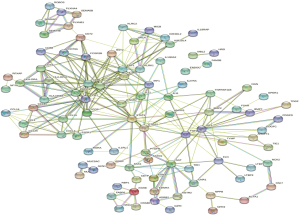
Table 1
Rank | Degree | MCC | Common | Gene title |
---|---|---|---|---|
1 | AGT | AGT | – | – |
2 | CCR7 | SAA1 | – | – |
3 | STAT3 | GPR17 | AGT | Angiotensinogen |
4 | SAA1 | AGTR2 | CCR7 | C-C motif chemokine receptor 7 |
5 | CD86 | CCR7 | STAT3 | Signal transducer and activator of transcription 3 |
6 | FGF2 | S1PR1 | SAA1 | Serum amyloid A1 |
7 | AGTR2 | CCL19 | CD86 | CD86 molecule |
8 | IGF1 | SSTR2 | AGTR2 | Angiotensin II receptor type 2 |
9 | PTGS2 | CCL21 | GPR17 | G protein-coupled receptor 17 |
10 | GPR17 | CXCL3 | SSTR2 | Somatostatin receptor 2 |
11 | SSTR2 | GPER1 | CD40 | CD40 molecule |
12 | CD40 | CCL1 | S1PR1 | Sphingosine-1-phosphate receptor 1 |
13 | S1PR1 | RXFP3 | CCL19 | C-C motif chemokine ligand 19 |
14 | CCL19 | CCL25 | CCL21 | C-C motif chemokine ligand 21 |
15 | CCL21 | CCL16 | IL21 | Interleukin 21 |
16 | IL21 | TRH | CXCL3 | C-X-C motif chemokine ligand 3 |
17 | CXCL3 | EDN3 | GPER1 | G protein-coupled estrogen receptor 1 |
18 | GPER1 | NMBR | PRF1 | Perforin 1 |
19 | PRF1 | EDNRA | CCL1 | C-C motif chemokine ligand 19 |
20 | CCL1 | PTGFR | RXFP3 | relaxin/insulin like family peptide receptor 3 |
21 | RXFP3 | CD86 | CCL25 | C-C motif chemokine ligand 25 |
22 | CCL25 | CD40 | CCL16 | C-C motif chemokine ligand 16 |
23 | CCL16 | PRF1 | KLRK1 | Killer cell lectin like receptor K1 |
24 | KLRK1 | PDCD1 | PDCD1 | Programmed cell death 1 |
25 | PDCD1 | STAT3 | TRH | Thyrotropin releasing hormone |
26 | TRH | IL21 | EDN3 | Endothelin 3 |
27 | FCGR2B | KLRK1 | – | – |
28 | EDN3 | ICOS | – | – |
MCC, maximal clique centrality.
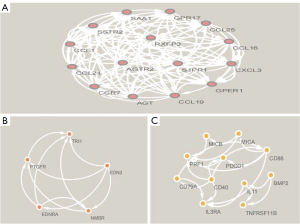
Analysis of immune cells
We presented the infiltration of immune cells into each sample by a heat map (Figure 6A). We found that natural killer (NK) cells activated, macrophages M0, and mast cells (MCs) resting showed changes in infiltration between groups. We removed both T cells gamma delta and MCs activated because they did not infiltrate in both infertile and fertile groups. We used Wilcoxon’s test to compare the levels of penetration of 20 immune cells in different groups (Figure 6B). We found that macrophages M0 (P=0.016) and MCs resting (P=0.032) were more abundant in the infertile group. Nevertheless, fewer NK cells activated (P=0.032) were identified in the infertile group. To further understand the role of 24 hub genes in immune cells infiltration, we evaluated the relationship between the hub genes and 3 types of immune cells (Figure 6C). We found that 17 hub genes were related to macrophages M0, MCs resting, or NK cells activated.
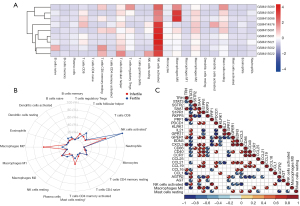
Validation in GSE92324 dataset
We employed the GSE92324 dataset as an independent data for testing. We did a gene difference analysis and found that there were 1,149 different genes in infertile and healthy fertile control tissues (Figure 7A). We intersected the 1,149 genes with the above 17 genes and found 3 common genes including CD40, PRF1, and EDN3 (Figure 7B). As shown in Figure 7C, we found that the 3 genes were significantly different in infertile and healthy fertility groups. The infertile patients exhibited significantly higher EDN3 expression (P=0.00021), and lower CD40 (P=0.024) and PRF1 (P=0.0082) expression. The results demonstrated that these 3 genes were related to infertility. Furthermore, we also found that 3 genes had good diagnostic values for infertility. The AUCs of CD40, EDN3, and PRF1 were 0.951, 1.000, and 0.778, respectively (Figure 7D-7F).
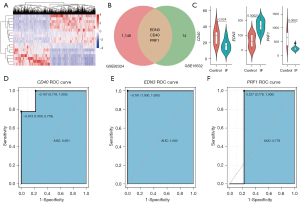
CD40, PRF1, and EDN3 GSEA in GSE92324 dataset
In order to explore the biological functions related to CD40, PRF1, and EDN3, other genes in the GSE92324 data set were sequenced according to their expression correlation with the 3 genes, and then GSEA was performed. We confirmed that GO terms such as adaptive immune response, cell adhesion molecule binding, cell substrate junction, collagen containing extracellular matrix, external side of plasma membrane were associated with CD40 (Figure 8A). We obtained the GO enrichment in which EDN3 was involved in including adaptive immune response, mitochondrial protein complex, positive regulation of cell activation, positive regulation of lymphocyte activation, and t cell activation (Figure 8B). We found that these GO terms included collagen containing extracellular matrix, extracellular structure organization, phagocytosis, platelet degranulation, and vesicle lumen related to PRF1 (Figure 8C). The GSEA analysis revealed 5 significant KEGG pathways associated with CD40, including complement and coagulation cascades, focal adhesion, oxidative phosphorylation, pathways in cancer, and the transforming growth factor-beta (TGF-β) signaling pathway (Figure 8D). As shown in Figure 8E, pathways including cell adhesion molecules (CAMS), cytokine-cytokine receptor interaction, primary immunodeficiency, starch and sucrose metabolism, and terpenoid backbone biosynthesis were enriched in the EDN3 expression phenotype. GSEA revealed that dilated cardiomyopathy, focal adhesion, graft-versus-host disease, hypertrophic cardiomyopathy (HCM), and NK cell-mediated cytotoxicity were significantly enriched in the PRF1 expression phenotype (Figure 8F).
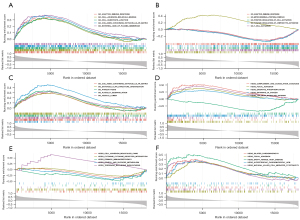
Validation in GSE120103 dataset
We further verified CD40, PRF1, and EDN3 in GSE120103. Gene differential expression analysis displayed that CD40 and PRF1 were downregulated. Although EDN3 was up-regulated, there was no significant difference (Figure 9A-9C). In addition, the results of the GSE120103 dataset showed that 3 genes had good diagnostic values for infertility. The AUCs of CD40, EDN3, and PRF1 were 1.000, 0.778, and 0.951, respectively (Figure 9D-9F).
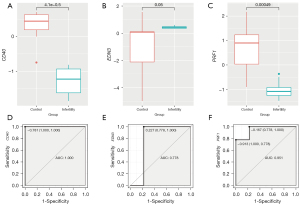
Validation of differentially expressed levels for 3 key genes by western blot
A total of 12 infertile patients and 12 healthy female controls were included in this study. Western blot detection was performed on the expression proteins of CD40, EDN3, and PRF1 in the 2 groups, and the results showed that they were downregulated in infertile tissues compared with normal samples (Figure 10).
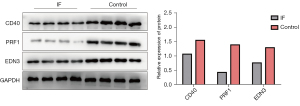
Validation of differentially expressed levels for 3 key genes by IHC
We validated the expression of CD40, EDN3, and PRF1 proteins in clinical specimens by IHC. As shown in Figure 11, the relative expression levels of the 3 genes in infertile samples were lower than in fertile samples.
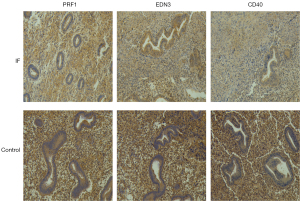
Discussion
In our study, the analyses of DEGs from the GEO database and IRGs from the ImmPort database were combined to identify biomarkers associated with UEI. We used CIBERSORT to evaluate immune cell infiltration into the microenvironment of UEI patients. Then, the correlations between DEIRGs and infiltration of 3 types of immune cells in UEI were estimated. Finally, on the basis of independent dataset and clinical specimen verification, CD40, PRF1, and EDN3 were confirmed as potential biomarkers of UEI. Mechanistically, the 3 genes may function through multiple immune-related BP and MF.
Our study analyzed the difference between the immune infiltrating cells in unexplained infertile tissue and normal endometrial tissue. The results revealed the imbalance of resting MCs, M0 macrophages, and activated NK cells. This implies that these 3 types of cells may be related to the disorder of the immune microenvironment in unexplained infertile patients. MCs have been recognized as important effector cells involved in innate immunity, allergic reactions, and immune surveillance (20-22). They are found in the cervix, myometrium, and endometrium of rodents and humans, and are closely associated with sensory nerves and blood vessels (22). In the resting phase, MCs develop cytosolic granules containing cytokines, peptides, and biogenic amines, as well as proteases (chymase, tryptase, and carboxypeptidase A3), which are released upon activation (23). A study has revealed a central role for MCs in implantation and fetal survival through mediating placentation and spiral artery formation, both key events that ensure optimal development of the fetus (22). Another study has shown that MCs are crucially involved in EMS-associated infertility. They found that stem cell factor, the main chemoattractant and growth differentiation factor for MC, is at a higher concentration in the peritoneal fluid of EMS patients (21). Our findings are consistent with these reports. We found that the extent of resting MCs infiltration in the endometrial tissue of infertile patients was up-regulated. Macrophages are involved in various immune responses through antigen presentation, phagocytosis, and secretion of a variety of growth factors and cytokines (24,25). Macrophages play crucial roles in pregnancy. Li et al. found significantly elevated proportions of CD68+ macrophages in recurrent reproductive failure patients with chronic endometritis (CE) (8). Increased macrophages have been observed in the peritoneal fluids from women with EMS, implicating that these cells could be associated with the occurrence of EMS-associated infertility (21,26). A study reported that the number of stromal macrophage in endometrial functional layer increased in patients with implantation failure (27). Our results confirmed that the infiltration level of M0 macrophages was elevated in patients with infertility. The exact mechanisms by which the observed increase in macrophage density may inhibit fertility are still a matter of debate. A study has shown that macrophages have the capability to secrete cytokines and reactive oxygen species, all of which are potentially toxic to embryos (27). NK cells are considered the most important cells in our immune defense system (28). They exist in the blood circulation and peripheral organs to perform the function of innate immune response (7). Human endometrium contains an unusual type of NK cells, termed uterine NK (uNK) cells, which have been the most investigated of the endometrial population of leucocytes (29). uNK cells can produce cytokines creating a pro-invasive environment and promoting spiral artery remodeling and supporting trophoblast implantation (30). uNK cells are very important for pregnancy. Dysregulated uNK cells may be harmful to implantation and pregnancy. The quantitative and qualitative imbalance of NK cells may lead to the disruption of implantation and placental processes, causing an adverse obstetric outcome (31). A study demonstrated that lower endometrial NK cell percentages were detected in primary infertility women (32). Infertile patients had a lower frequency of NK T-like cells than normal subjects (33). Our result shows that activated NK cells were decreased in endometrial tissue of infertile patients, and could be regarded as a potential biological marker.
Our study also identified 3 genes (CD40, PRF1, and EDN3) associated with the microenvironment of patients with UEI. CD40 is a member of the tumor necrosis factor receptor (TNF-R) family (34,35). CD40 is widely distributed in many normal cells, such as epithelial cells, macrophages, B cells, dendritic cells, smooth muscle cells, fibroblasts, and activated platelets (35-37). CD40 plays a critical role in adaptive and innate immunity and activates immune responses after interacting with its ligand, CD40L (CD154). CD40 promotes B cells development and proliferation by increasing the production of Interleukins and chemokines and induces B cells to differentiate into plasma cells that secrete antibodies (38). CD40 is also an effective inducer of Th1 (T-helper type 1) cytokines (39). Activated CD40 can enhance transcription factors including STAT-3 and NF-κB, which leads to the induction of cytokines such as IL-6 and IL-10 (39). Recently, it was reported that CD40 up-regulation and CD40-CD40L cross-talk are related to Th17/Treg imbalance (40). Another study showed that CD40-CD40L interaction induced the production of TGF-β leads to the enhancement of immunosuppression (38). The expression of CD40 on uterine epithelial cells suggests that the CD40 may have a role in the pathogenesis of pregnancy (41). Our results indicate that the levels of CD40 is decreased in infertile patients compared with the normal pregnancy. In a microarray study, CD40 and its ligand CD40L appear to be highly dysregulated in the endometrium of infertile patients, and it is assumed to interact with G-CSF (36). Polymerase chain reaction (PCR) array data analysis identified significantly lower expression of CD40 and greater expression of CD40L in unexplained repeated implantation failure women than in healthy fertile women (42). CD40 ligation-induced innate immune activation is related to early pregnancy loss and endocrine dysfunction (43). At present, the specific role of CD40 in the mechanism of infertility is unclear. However, Laskowska et al. found that a higher levels of soluble CD40L could prevent maternal immune cells carrying CD40 receptor from Fas-mediated apoptosis (44). Another study indicated that, during normal pregnancy, the CD40-CD40L interaction between CD4+ T lymphocytes and antigen presenting cells is reduced, which may be one of the mechanisms that protect the developing fetus from the activation of the maternal immune system (45). Endothelins (EDNs), found in humans, participate in a variety of physiological events (46). EDNs and their G protein-coupled receptors A and B negatively regulate the immune response. Available evidence shows that endothelin promotes the polarization of macrophages to the M2 phenotype, and stimulates the secretion of the immunosuppressive cytokines TGF-β and IL-10 by macrophages (47). Yan et al. revealed that EDNRA is correlated with interleukin 1 receptor binding, activation of NK cells involved in immune response, and immunoregulatory interactions between non-lymphoid and lymphoid cell pathways (48). The EDN3 protein is a member of the endothelin/sarafotoxin family and is a secreted vasoactive peptide. Looking up a lot of literature, the relationship between EDN3 and immunity response is not clear. However, it was found that EDN3 increased the level of FOXP3+ Tregs, which indicated that EDN3 promoted Tregs proliferation, increasing FOXP3 expressing cells (49). Both Ednrb−/− and Edn3−/− enterocolitis mice showed a significant decrease in thymus volume, mature B cells, and CD4 and CD8 T cells (50). A study showed that EDN3 was associated with epithelial-mesenchymal transition (EMT) and TGF-β signaling, and may regulate immune cell infiltration in prostate cancer (51). Liu et al. identified that EDN3 is a key immune gene related to Alzheimer’s Disease (AD), which may become a new target of immunotherapy for AD patients (52). EDN3 is essential in ovulation, folliculogenesis, and embryo implantation. An early study has shown that EDN3 regulates oviductal secretions (53). In addition, it may also affect ciliary activity in oviductal epithelial cells (53). Mice treated with endothelin receptor antagonists excreted fewer 2-cell embryos from the oviduct (54). Several studies have reached a consistent conclusion that EDN3 is downregulated in endometrial tissue during the window of implantation, which promotes embryo implantation (55-58). Some studies have provided evidence that EDN3 and EDNRA are localized in endometrial vascular smooth muscle cells, suggesting that this vasoactive agent may play a role in the local control of the uterine vascular bed (59-61). Our gene differential expression revealed that EDN3 is up-regulated in UEI patients, but this has not been verified in independent validation data sets, and the opposite conclusion has been reached in clinical trials. However, combined with other existing research conclusions, the effect of EDN3 on UEI patients deserves further study. The PRF1 gene encodes perforin (PRF1), which plays a crucial role in the granule-dependent killing activities of NK cells and cytotoxic T lymphocytes (CTLs) (62). Fan et al. findings displayed that high PRF1 expression had elevated levels of M1 macrophage, NK cell, CD4+ T cell, and CD8+ T cell infiltration in head and neck squamous cell carcinoma (63). Sidore et al. discovered that PRF1 mutation, PRF1:p.A91V, is helpful to regulate different immune subtypes, especially to enhance the memory cytotoxic T cell compartment: effector-memory CD8bright, CD8dim, TCR γδ+, and CD8dimCD28− T cell pool (64). Perforin pathway interruption leads to autoimmune disorders, neoplastic disease, transplant rejection, and prolonged inflammation (64-68). Our study revealed that PRF1 was downregulated in unexplained infertile patients. A recent study reported that miR-30e can reduce the cytotoxicity in NK cells by targeting PRF1, which inhibits Th1 tolerance phenotype and induces Th2 immunodominance, consequently forming an immune tolerance microenvironment at the maternal-fetal interface (69). Another study declared that the high messenger RNA (mRNA) expression of PRF1 in peripheral CD300a+CD8+ T lymphocytes may be related to the anti-fetal cellular rejection of the chorioamniotic membranes (70). At present, the potential role of PRF1 in UEI has not been clearly studied. Relevant evidence and research are scarce. Therefore, the influence of PRF1 on the occurrence and progression of infertility needs to be further clarified.
We conducted an integrated bioinformatic analysis based on the public database to identify key IRGs associated with UEI. Our findings were also confirmed by practical clinical sample data. However, the present study had several limitations. First, since the GEO database does not provide enough clinical information for our purposes, the association between the key genes and clinicopathological features of infertile was not analyzed. Second, whether the genes have the ability to be used to screen out infertile patients suitable for immunotherapy and use them as targets for immunotherapy has not been confirmed. Finally, due to the scarcity of clinical samples, the analysis results may not accurately reflect the actual clinical value of candidate genes. In future research, we will try to collect the larger clinical sample data to verify our findings and improve the reliability of our conclusion. Further, we will use new statistical methods to build a predictive model to predict the prognostic value of biomarkers for unexplained infertile patients, instructing immunotherapy for these patients.
Conclusions
In conclusion, 3 IRGs associated with UEI were found and validated. They are expected to become new therapeutic targets for unexplained infertile patients. However, further in-depth studies are essential to validate our findings. Ultimately, we expect that these molecular biomarkers will help the screening patients with UEI who are suitable for immunotherapy and to guide immunotherapy.
Acknowledgments
Funding: None.
Footnote
Reporting Checklist: The authors have completed the MDAR reporting checklist. Available at https://atm.amegroups.com/article/view/10.21037/atm-22-5810/rc
Data Sharing Statement: Available at https://atm.amegroups.com/article/view/10.21037/atm-22-5810/dss
Conflicts of Interest: All authors have completed the ICMJE uniform disclosure form (available at https://atm.amegroups.com/article/view/10.21037/atm-22-5810/coif). The authors have no conflicts of interest to declare.
Ethical Statement: The authors are accountable for all aspects of the work in ensuring that questions related to the accuracy or integrity of any part of the work are appropriately investigated and resolved. The study was conducted in accordance with the Declaration of Helsinki (as revised in 2013). This study was conducted with approval by the Ethics Committee of The First Hospital of Lanzhou University (approval No. LDYYLL2022-246) and informed consent was taken from all the patients.
Open Access Statement: This is an Open Access article distributed in accordance with the Creative Commons Attribution-NonCommercial-NoDerivs 4.0 International License (CC BY-NC-ND 4.0), which permits the non-commercial replication and distribution of the article with the strict proviso that no changes or edits are made and the original work is properly cited (including links to both the formal publication through the relevant DOI and the license). See: https://creativecommons.org/licenses/by-nc-nd/4.0/.
References
- Ejzenberg D, Andraus W, Baratelli Carelli Mendes LR, et al. Livebirth after uterus transplantation from a deceased donor in a recipient with uterine infertility. Lancet 2019;392:2697-704. [Crossref] [PubMed]
- Carson SA, Kallen AN. Diagnosis and Management of Infertility: A Review. JAMA 2021;326:65-76. [Crossref] [PubMed]
- Raad G, Tanios J, Azoury J, et al. Neurophysiology of cognitive behavioural therapy, deep breathing and progressive muscle relaxation used in conjunction with ART treatments: a narrative review. Hum Reprod Update 2021;27:324-38. [Crossref] [PubMed]
- Practice Committee of the American Society for Reproductive Medicine. Electronic address: asrm@asrm; . Evidence-based treatments for couples with unexplained infertility: a guideline. Fertil Steril 2020;113:305-22. [Crossref] [PubMed]
- Gunn DD, Bates GW. Evidence-based approach to unexplained infertility: a systematic review. Fertil Steril 2016;105:1566-1574.e1. [Crossref] [PubMed]
- Jafarpour R, Pashangzadeh S, Mehdizadeh S, et al. Functional significance of lymphocytes in pregnancy and lymphocyte immunotherapy in infertility: A comprehensive review and update. Int Immunopharmacol 2020;87:106776. [Crossref] [PubMed]
- Kofod L, Lindhard A, Hviid TVF. Implications of uterine NK cells and regulatory T cells in the endometrium of infertile women. Hum Immunol 2018;79:693-701. [Crossref] [PubMed]
- Li Y, Yu S, Huang C, et al. Evaluation of peripheral and uterine immune status of chronic endometritis in patients with recurrent reproductive failure. Fertil Steril 2020;113:187-196.e1. [Crossref] [PubMed]
- Shakerian B, Irvani S, Mostafavi S, et al. Quantitative serum determination of CD3, CD4, CD8, CD16, and CD56 in women with primary infertility: The role of cell-mediated immunity. Turk J Obstet Gynecol 2022;19:242-5. [Crossref] [PubMed]
- Robertson SA, Care AS, Moldenhauer LM. Regulatory T cells in embryo implantation and the immune response to pregnancy. J Clin Invest 2018;128:4224-35. [Crossref] [PubMed]
- Bai YL, Chen YH, Jiang C, et al. Efficacy and Safety of Traditional Chinese Medicine in the Treatment of Immune Infertility Based on the Theory of "Kidney Deficiency and Blood Stasis": A Systematic Review and Meta-Analysis. Evid Based Complement Alternat Med 2021;2021:9947348. [Crossref] [PubMed]
- Brazdova A, Senechal H, Peltre G, et al. Immune Aspects of Female Infertility. Int J Fertil Steril 2016;10:1-10. [PubMed]
- Ehsani M, Mohammadnia-Afrouzi M, Esmaeilzadeh S, et al. Decreased Frequency of CD8(+)HLA-G(+) T Cell in the Peripheral Blood of Primary Unexplained Infertile Females. Reprod Sci 2021;28:1939-44. [Crossref] [PubMed]
- Madendag IC, Sahin ME, Madendag Y, et al. Evaluation of the levels of secretory leukocyte protease inhibitor in the cervical mucus of women with unexplained infertility. J Obstet Gynaecol Res 2020;46:1128-32. [Crossref] [PubMed]
- Kanannejad Z, Jahromi BN, Gharesi-Fard B. Seminal plasma and CD4(+) T-cell cytokine profiles in the in vitro fertilization success. J Res Med Sci 2020;25:26. [PubMed]
- Lőrincz J, Molnár S, Jakab A, et al. The effect of localization and histological verification of endometrial polyps on infertility. Arch Gynecol Obstet 2019;300:217-21. [Crossref] [PubMed]
- Adedigba JA, Idowu BM, Hermans SP, et al. The relationship between hysterosalpingography findings and female infertility in a Nigerian population. Pol J Radiol 2020;85:e188-95. [Crossref] [PubMed]
- Huang Y, Li Q, Hu R, et al. Five immune-related genes as diagnostic markers for endometriosis and their correlation with immune infiltration. Front Endocrinol (Lausanne) 2022;13:1011742. [Crossref] [PubMed]
- Yan P, Yao L, Li H, et al. The methodological quality of robotic surgical meta-analyses needed to be improved: a cross-sectional study. J Clin Epidemiol 2019;109:20-9. [Crossref] [PubMed]
- Wareham KJ, Seward EP. P2X7 receptors induce degranulation in human mast cells. Purinergic Signal 2016;12:235-46. [Crossref] [PubMed]
- Borelli V, Martinelli M, Luppi S, et al. Mast Cells in Peritoneal Fluid From Women With Endometriosis and Their Possible Role in Modulating Sperm Function. Front Physiol 2019;10:1543. [Crossref] [PubMed]
- Woidacki K, Popovic M, Metz M, et al. Mast cells rescue implantation defects caused by c-kit deficiency. Cell Death Dis 2013;4:e462. [Crossref] [PubMed]
- Poerwosusanta H. The effect of laparoscopy on mast cell degranulation and mesothelium thickness in rats. BMC Surg 2020;20:111. [Crossref] [PubMed]
- Jena MK, Nayak N, Chen K, et al. Role of Macrophages in Pregnancy and Related Complications. Arch Immunol Ther Exp (Warsz) 2019;67:295-309. [Crossref] [PubMed]
- Ono Y, Yoshino O, Hiraoka T, et al. CD206+ M2-Like Macrophages Are Essential for Successful Implantation. Front Immunol 2020;11:557184. [Crossref] [PubMed]
- Sekulovski N, Whorton AE, Tanaka T, et al. Niclosamide suppresses macrophage-induced inflammation in endometriosis†. Biol Reprod 2020;102:1011-9. [Crossref] [PubMed]
- Tremellen KP, Russell P. The distribution of immune cells and macrophages in the endometrium of women with recurrent reproductive failure. II: adenomyosis and macrophages. J Reprod Immunol 2012;93:58-63. [Crossref] [PubMed]
- Ulčová-Gallová Z, Pešek M, Chaloupka P, et al. Screening of endometrial NK cells in selected infertile patients First part - Methods and current results. Ceska Gynekol 2017;82:366-71. [PubMed]
- Bulmer JN, Lash GE. The Role of Uterine NK Cells in Normal Reproduction and Reproductive Disorders. Adv Exp Med Biol 2015;868:95-126. [Crossref] [PubMed]
- Freitag N, Pour SJ, Fehm TN, et al. Are uterine natural killer and plasma cells in infertility patients associated with endometriosis, repeated implantation failure, or recurrent pregnancy loss? Arch Gynecol Obstet 2020;302:1487-94. [Crossref] [PubMed]
- Krivonos MI, Kh, Khizroeva J, Zainulina MS, et al. The role of lymphocytic cells in infertility and reproductive failures in women with antiphospholipid antibodies. J Matern Fetal Neonatal Med 2022;35:871-7. [Crossref] [PubMed]
- Rizzo R, Lo Monte G, Bortolotti D, et al. Impact of soluble HLA-G levels and endometrial NK cells in uterine flushing samples from primary and secondary unexplained infertile women. Int J Mol Sci 2015;16:5510-6. [Crossref] [PubMed]
- Hosseini S, Shokri F, Pour SA, et al. Diminished Frequency of Menstrual and Peripheral Blood NKT-Like Cells in Patients With Unexplained Recurrent Spontaneous Abortion and Infertile Women. Reprod Sci 2019;26:97-108. [Crossref] [PubMed]
- Wu CF, Huang FD, Sui RF, et al. Preeclampsia serum upregulates CD40/CD40L expression and induces apoptosis in human umbilical cord endothelial cells. Reprod Biol Endocrinol 2012;10:28. [Crossref] [PubMed]
- Filbert EL, Björck PK, Srivastava MK, et al. APX005M, a CD40 agonist antibody with unique epitope specificity and Fc receptor binding profile for optimal therapeutic application. Cancer Immunol Immunother 2021;70:1853-65. [Crossref] [PubMed]
- Dumas G, Dufresne M, Asselin É, et al. CD40 pathway activation reveals dual function for macrophages in human endometrial cancer cell survival and invasion. Cancer Immunol Immunother 2013;62:273-83. [Crossref] [PubMed]
- Azzam HA, Abousamra NK, Goda H, et al. The expression and concentration of CD40 ligand in normal pregnancy, preeclampsia, and hemolytic anemia, elevated liver enzymes and low platelet count (HELLP) syndrome. Blood Coagul Fibrinolysis 2013;24:71-5. [Crossref] [PubMed]
- Kim H, Kim Y, Bae S, et al. Direct Interaction of CD40 on Tumor Cells with CD40L on T Cells Increases the Proliferation of Tumor Cells by Enhancing TGF-β Production and Th17 Differentiation. PLoS One 2015;10:e0125742. [Crossref] [PubMed]
- Gold JA, Parsey M, Hoshino Y, et al. CD40 contributes to lethality in acute sepsis: in vivo role for CD40 in innate immunity. Infect Immun 2003;71:3521-8. [Crossref] [PubMed]
- Su X, Zhang J, Qin X. CD40 up-regulation on dendritic cells correlates with Th17/Treg imbalance in chronic periodontitis in young population. Innate Immun 2020;26:482-9. [Crossref] [PubMed]
- Fahey JV, Wallace PK, Johnson K, et al. Antigen presentation by human uterine epithelial cells to autologous T cells. Am J Reprod Immunol 2006;55:1-11. [Crossref] [PubMed]
- Amjadi F, Zandieh Z, Mehdizadeh M, et al. The uterine immunological changes may be responsible for repeated implantation failure. J Reprod Immunol 2020;138:103080. [Crossref] [PubMed]
- Tanaka H, Maeda K, Nakamura Y, et al. CD40 and IFN-gamma dependent T cell activation by human bronchial epithelial cells. J Med Invest 2001;48:109-17. [PubMed]
- Laskowska M, Laskowska K, Leszczyńska-Gorzelak B, et al. sCD40 ligand determined in maternal and umbilical cord blood in pregnancies complicated by pre-eclampsia with and without intrauterine growth retardation. Gynecol Obstet Invest 2007;64:8-13. [Crossref] [PubMed]
- Darmochwal-Kolarz D, Kolarz B, Surdacka A, et al. The expression and concentration of CD40 ligand in normal pregnancy and pre-eclampsia. J Reprod Immunol 2009;79:215-9. [Crossref] [PubMed]
- Kobayashi Y, Yoshimoto Y, Yamamoto Y, et al. Roles of EDNs in regulating oviductal NO synthesis and smooth muscle motility in cows. Reproduction 2016;151:615-22. [Crossref] [PubMed]
- Soldano S, Pizzorni C, Paolino S, et al. Alternatively Activated (M2) Macrophage Phenotype Is Inducible by Endothelin-1 in Cultured Human Macrophages. PLoS One 2016;11:e0166433. [Crossref] [PubMed]
- Yan Y, Nie K, Zheng J, et al. High Endothelin Receptor Type A Expression as an Independent Prognostic Biomarker and Correlated with Immune Infiltrates in Stomach Adenocarcinoma. Cancer Manag Res 2021;13:5013-26. [Crossref] [PubMed]
- Freitas JT, Lopez J, Llorian C, et al. The immunosuppressive role of Edn3 overexpression in the melanoma microenvironment. Pigment Cell Melanoma Res 2021;34:1084-93. [Crossref] [PubMed]
- Frykman PK, Cheng Z, Wang X, et al. Enterocolitis causes profound lymphoid depletion in endothelin receptor B- and endothelin 3-null mouse models of Hirschsprung-associated enterocolitis. Eur J Immunol 2015;45:807-17. [Crossref] [PubMed]
- Zhang P, Qian B, Liu Z, et al. Identification of novel biomarkers of prostate cancer through integrated analysis. Transl Androl Urol 2021;10:3239-54. [Crossref] [PubMed]
- Liu C, Zhang X, Chai H, et al. Identification of Immune Cells and Key Genes associated with Alzheimer's Disease. Int J Med Sci 2022;19:112-25. [Crossref] [PubMed]
- Akison LK, Boden MJ, Kennaway DJ, et al. Progesterone receptor-dependent regulation of genes in the oviducts of female mice. Physiol Genomics 2014;46:583-92. [Crossref] [PubMed]
- Zhou H, Chen L, Lei Y, et al. Integrated analysis of tumor mutation burden and immune infiltrates in endometrial cancer. Curr Probl Cancer 2021;45:100660. [Crossref] [PubMed]
- Tapia A, Vilos C, Marín JC, et al. Bioinformatic detection of E47, E2F1 and SREBP1 transcription factors as potential regulators of genes associated to acquisition of endometrial receptivity. Reprod Biol Endocrinol 2011;9:14. [Crossref] [PubMed]
- Kao LC, Tulac S, Lobo S, et al. Global gene profiling in human endometrium during the window of implantation. Endocrinology 2002;143:2119-38. [Crossref] [PubMed]
- Mirkin S, Arslan M, Churikov D, et al. In search of candidate genes critically expressed in the human endometrium during the window of implantation. Hum Reprod 2005;20:2104-17. [Crossref] [PubMed]
- Jeoung M, Lee S, Hawng HK, et al. Identification of a novel role for endothelins within the oviduct. Endocrinology 2010;151:2858-67. [Crossref] [PubMed]
- Cameron IT, Plumpton C, Champeney R, et al. Identification of endothelin-1, endothelin-2 and endothelin-3 in human endometrium. J Reprod Fertil 1993;98:251-5. [Crossref] [PubMed]
- Cameron IT, Davenport AP, van Papendorp C, et al. Endothelin-like immunoreactivity in human endometrium. J Reprod Fertil 1992;95:623-8. [Crossref] [PubMed]
- Keator CS, Mah K, Ohm L, et al. Estrogen and progesterone regulate expression of the endothelins in the rhesus macaque endometrium. Hum Reprod 2011;26:1715-28. [Crossref] [PubMed]
- Bolitho P, Voskoboinik I, Trapani JA, et al. Apoptosis induced by the lymphocyte effector molecule perforin. Curr Opin Immunol 2007;19:339-47. [Crossref] [PubMed]
- Fan C, Hu H, Shen Y, et al. PRF1 is a prognostic marker and correlated with immune infiltration in head and neck squamous cell carcinoma. Transl Oncol 2021;14:101042. [Crossref] [PubMed]
- Sidore C, Orrù V, Cocco E, et al. PRF1 mutation alters immune system activation, inflammation, and risk of autoimmunity. Mult Scler 2021;27:1332-40. [Crossref] [PubMed]
- Fink TM, Zimmer M, Weitz S, et al. Human perforin (PRF1) maps to 10q22, a region that is syntenic with mouse chromosome 10. Genomics 1992;13:1300-2. [Crossref] [PubMed]
- Romero CA, Sánchez IP, Gutierrez-Hincapié S, et al. A novel pathogenic variant in PRF1 associated with hemophagocytic lymphohistiocytosis. J Clin Immunol 2015;35:501-11. [Crossref] [PubMed]
- Alcaraz-Sanabria A, Baliu-Piqué M, Saiz-Ladera C, et al. Genomic Signatures of Immune Activation Predict Outcome in Advanced Stages of Ovarian Cancer and Basal-Like Breast Tumors. Front Oncol 2019;9:1486. [Crossref] [PubMed]
- Rodriguez RM, Suarez-Alvarez B, Salvanés R, et al. DNA methylation dynamics in blood after hematopoietic cell transplant. PLoS One 2013;8:e56931. [Crossref] [PubMed]
- Huang Q, Ding J, Gong M, et al. Effect of miR-30e regulating NK cell activities on immune tolerance of maternal-fetal interface by targeting PRF1. Biomed Pharmacother 2019;109:1478-87. [Crossref] [PubMed]
- Xu Y, Tarquini F, Romero R, et al. Peripheral CD300a+CD8+ T lymphocytes with a distinct cytotoxic molecular signature increase in pregnant women with chronic chorioamnionitis. Am J Reprod Immunol 2012;67:184-97. [Crossref] [PubMed]