The combined effect and mechanism of antiangiogenic drugs and PD-L1 inhibitor on cell apoptosis in triple negative breast cancer
Highlight box
Key findings
• Anlotinib and PD-L1 inhibitor can inhibit the proliferation, migration and invasion of TNBC cells in vivo and in vitro, promote apoptosis, and have a combined effect.
What is known and what is new?
• The anti-angiogenic mechanism of anlotinib has been clearly studied. PD-L1 inhibitor treatment of TNBC is the main research field of immunotherapy for breast cancer.
• The important internal signaling role of PD-L1 has been rarely studied. The mechanism of action of anlotinib combined with PD-L1 inhibitor on TNBC is unknown.
What is the implication, and what should change now?
• Our research may provide theoretical and experimental basis for TNBC antiangiogenic therapy combined with immunotherapy, and we hope to have more relevant research reports.
Introduction
Triple-negative breast cancer (TNBC) accounts for 15–20% of breast tumors and is frequent in women under the age of 40 (1). It is characterized by the absence of estrogen receptors (ER), progesterone receptors (PR), human epidermal growth factor receptor 2 (HER-2) and lacks targeted therapies; TNBC presents aggressive histological features, a greater risk of loco-regional and systemic recurrence at 5 years with worse DFS and OS (2,3). Standard systemic treatment includes chemotherapy and immunotherapy. PARP inhibitors are considered in BRCA mutated tumors. Therefore, there is an urgent need for more effective treatments for TNBC in clinical practice, and this has become a hot research topic in recent years (4).
Studies have shown angiogenesis plays a key role in breast cancer (5,6). Anti-angiogenesis drugs inhibit tumor growth and metastasis by preventing vascular endothelial growth factor (VEGF) from binding to vascular endothelial growth factor receptor (VEGFR) (7). Current anti-angiogenesis drugs include biological monoclonal antibodies and small molecule drugs. Anlotinib, is a novel small molecule multi-target tyrosine kinase. Anlotinib can strongly inhibit multiple targets such as VEGFR, where its inhibitory effect is strongest, as well as PDGFR (7-9). It can inhibit the VEGFR2/PI3K/AKT signaling pathway, down-regulating downstream Bcl-2 molecules, promote cell apoptosis, and inhibit cell proliferation, invasion, and metastasis (10). Bcl-xl is a member of the Bcl-2 family which inhibits cell apoptosis, and is closely related to tumor cell apoptosis (11,12). To date, vast majority of anti-angiogenesis targeted therapy has focused on the ligand/receptor interaction in the vascular endothelial cells, while these signaling can occur in cancer cells as well, which were often ignored. Anlotinib can inhibit the activation of the VEGFR2/PI3K/AKT pathway and further down-regulate Bcl-xl, but whether anlotinib can produce the same effect on TNBC cells has not been reported. We hypothesized that anlotinib inhibit the PI3K/AKT/Bcl-xl signaling pathway through its site of action and promotion of TNBC cell apoptosis.
Like targeted therapy, immunotherapy is a rapidly developing tumor treatment method, and has been shown to hold superior efficacy in the treatment of various tumors (13,14). The application of PD-1/PD-L1 inhibitor to treat TNBC is currently the main research field of breast cancer immunotherapy (15). Research shows that the expression of PD-L1 in triple negative breast cancer is related to the prognosis of patients (16). When PD-L1 is positive, immunotherapy can significantly improve the overall survival of advanced triple negative breast cancer (17). PD-L1 is expressed in most malignant solid tumors including breast cancer (18), and by inhibiting the binding of PD-1 and PD-L1, PD-1/PD-L1 inhibitors can activate the immune response of T cells to tumors and inhibit tumor growth (19-21). Most research on the PD-L1 signaling pathway has focused on the binding of PD-L1 to PD-1 to negatively regulate T cells, while PD-L1 also has important internal signaling effects, although these effects have rarely been studied. Research has shown that after PD-L1 inhibitor treatment, the phosphorylation levels of mTOR and AKT in tumor cells are reduced (22), and PD-L1 can prevent tumor cell apoptosis (19). PD-L1-deficient MDA-MB-231 TNBC cells have increased apoptosis compared with wild-type after application of cisplatin, and this is associated with decreased Bcl-2 expression (23). Therefore, PD-L1 inhibitors can also inhibit the phosphorylation of AKT in tumor cells and reduce the expression of Bcl-2, promoting tumor cell apoptosis.
In this study we explored the effects of anlotinib and PD-L1 inhibitors on the biological functions of TNBC, proliferation, migration, invasion, and cell apoptosis through in vitro and in vivo experiments, and explored their molecular mechanism in the apoptosis of TNBC cells by studying the specific molecular signaling pathways of PI3K/AKT/Bcl-xl. The results may provide a theoretical and experimental basis for TNBC anti-angiogenesis therapy combined with immunotherapy. We present the following article in accordance with the ARRIVE reporting checklist (available at https://atm.amegroups.com/article/view/10.21037/atm-22-6446/rc).
Methods
Cell lines and animals
A protocol was prepared before the study without registration. The human TNBC MDA-MB-468 (Procell CL-0290) cell line and BT-549 (Procell CL-0041) used in our experiment was provided by Wuhan Procell Life Technology Co., Ltd. We cultured MDA-MB-468 and BT-549 cells in DMEM or RPMI medium containing 10% fetal bovine serum (FBS) and placed them in a constant temperature and humidified incubator at 37 ℃ containing 5% CO2. The 24 SPF-grade BALB/c nude female mice aged 5–6 weeks used in this experiment were purchased from Changzhou Cavens Experimental Animal Co. Ltd.
Chemicals and antibodies
Anlotinib and PD-L1 inhibitor (SHR-1316 injection) were provided by Hengrui Medicine Co. Ltd. Anlotinib was stored at 4 ℃ and was dissolved in a solvent of PBS:DMSO =3:1 which was prepared as a 2 mg/mL solution and stored at −20 ℃ in the dark until further use. PD-L1 inhibitor and glycerin were mixed at a ratio of 1:1 and stored at −80 ℃ in the dark. The following antibodies: P13K, p-P13K, AKT, p-AKT, Bcl-xl, and GAPDH were obtained from Beijing Bioss Biotechnology Co. Ltd.
Cell slide and immunohistochemistry
The expression of PD-L1 in MDA-MB-468 and BT-549 TNBC cells was detected by cell slide and immunocytochemistry (IHC), and PD-L1 primary antibody was used for overnight incubation.
3-(4,5-dimethylthiazol-2-Yl)-2,5-diphenyltetrazolium bromide (MTT) assays
An MTT colorimetric method was used to detect the effect of anlotinib and PD-L1 inhibitor on the proliferation of MDA-MB-468 and BT-549 cells and to verify whether the combination of the two drugs had a synergistic effect.
Cells were plated in 96-well plates in six replicates for 24 h (3×103 cells/well) then cultured with different concentrations of drugs for 48 hours, before 10 µL of MTT working solution was added to each well and cultured for another 4 hours. After removing the liquid, 150 µL DMSO was added to dissolve the formed crystals. The absorbance value of each well at 570 nm was detected by a microplate reader, and the average IC50 values were calculated by SPSS. Drug concentrations for the subsequent experiments were determined based on the IC50 values of anlotinib and PD-L1 inhibitors. Combination Index (CI) was calculated by CompuSyn software to verify whether the combination of the two drugs had a synergistic effect. The graphs were drawn by GraphPad software, and all assays were performed in triplicate.
Cell apoptosis assay
Cell apoptosis assay was used to analyze the effects of anlotinib and PD-L1 inhibitor on cell apoptosis. Cells apoptosis assay, cells were placed into 6-well plates at a density of 2×105 cells/well and incubated with different concentrations of drugs for 24 h. After washing with PBS, 1×106 cells were collected, washed twice with 4 ℃ PBS, then resuspended in 500 mL of annexin binding buffer. FITC-conjugated annexin V (5 mL) and propidium iodide (5 mL) were added to the solution and mixed. After incubation for 15 min at RT in the dark, the cells were analyzed using flow cytometry. The results were analyzed using FlowJo software, and all assays were performed in triplicate.
Wound healing and Transwell matrix assays
Wound healing and Transwell experiments were used to detect the effect of anlotinib and PD-L1 inhibitor on the migration and invasion of MDA-MB-468 and BT-549 cells, respectively.
Cells were cultured in 6-well plates at a density of 1×106 cells/well and were incubated until they reached 90% confluence. A scratch was created across the center of the cell layer using a sterile 200-µL pipette tip, and after each well was washed with PBS three times, photographs were taken under microscope. Each well cell was cultured with different concentrations of drug medium. After 24 h, the same cell section was photographed again, and the cell migration distance was calculated using Image J software. All assays were performed in triplicate.
Transwell matrix assays were performed as described below. One day in advance, the solid matrix glue was placed in a refrigerator at 4 ℃, and the cells were cultured in an FBS-free medium for 24 hours to remove the influence of serum on the results. A total of six Transwell cells were placed in 24-well plates, and the liquid matrigel and pre-cooled PBS were placed on ice at a ratio of 1:5 to prepare a dilution. Pre-cooled pipette tips were used to add 200 µL of matrigel dilution to the upper chamber of each Transwell chamber, and the 24-well plate was placed in the incubator for 1 hour to solidify the matrigel. Following this, 1×106 cells were plated on the matrigel in FBS-free medium and/or different drug concentrations and allowed to invade through the membrane towards medium containing 20% FBS in the bottom chamber. After 24 h, cells and matrigel above the membrane were wiped with cotton swabs, and cells below the membrane were fixed in 4% paraformaldehyde and stained with crystal violet for 30 min. Finally, five random ×100 microscopic fields were selected per sample, and cell numbers were counted by ImageJ software. All assays were performed in triplicate.
Western blotting (WB)
WB was performed to assess the expression of PI3K, p-PI3K, AKT, p-AKT, Bcl-xl in MDA-MB-468, and BT-549 TNBC cells after using anlotinib and PD-L1 inhibitor.
Western blotting was performed using the following standard protocols. Cells were cultured with media containing different concentrations of anlotinib or PD-L1 inhibitor for 24 h. Proteins were extracted with a cytoplasmic and nuclear protein extraction kit (Cowin Bio., Beijing, China) according to the manufacturer’s instructions then transferred on to polyvinylidene difluoride membranes. The membranes were blocked in 5% non-fat dried milk in tris-buffered saline (TBS) and then incubated overnight with the primary antibodies at 4 ℃. The blots were then incubated with the corresponding secondary antibodies conjugated with HRP at RT for 1 h. Visualization was conducted by enhanced chemiluminescence detection, and a gel imager was used for exposure imaging. ImageJ was used to analyze the gray value of the protein band, and SPSS software was used to calculate the P value.
Animal experiments
Animal experiments tested the effects of anlotinib and PD-L1 inhibitor drugs and the combination of the two on TNBC xenograft. Female thymic BALB/c nude mice, 4–6 weeks old, were housed in a specific pathogen-free (SPF) animal facility (temperature, 20–26 ℃; humidity, 40–60%; 12/12-h light/dark cycle; free access to food and water). Experiments were performed under a project license granted by the Medical Ethics Committee of the First Affiliated Hospital of Henan University of Science and Technology, in compliance with the National institutes of Health Guide for the Care and Use of Laboratory Animals, 8th edition. Random number tables were used as the randomization method to determine the animals allocated to experimental groups. Lymphocytes and 468 cells were mixed at a ratio of 1:4 (total 2.0×106/mL) and subcutaneously injected into the right area where the back meets the right forelimb (six mice per group). The experimental group of mice was treated via daily oral gavage with anlotinib (5 mg/kg/d, d1–d14), or intravenous injection of PD-L1 inhibitor (10 mg/kg, d0, d2, d4), or a combination of both drugs for 21 days, while control mice were treated with PBS. Tumor diameters and the weight of mice were measured every 3 days and tumor volumes (V) were calculated using the formula: V = (length × width2)/2.
Statistical analysis
The above MTT assays, cell apoptosis assays, wound healing assays, Transwell matrix assays, and WB experiment were repeated three times to reduce errors. GraphPad software was used to make graphs, and SPSS software was used for statistical analysis to calculate the P value. The measurement data was represented by “mean ± SD”, and comparisons between multiple groups of data were made by one-way analysis of variance (ANOVA). Comparison between the two groups was tested by independent sample t-test, and statistical significance was considered at P<0.05.
Results
Effects of anlotinib and PD-L1 inhibitor on the proliferation and apoptosis of MDA-MB-468 and BT-549 cells
To assess the effect of anlotinib and PD-L1 inhibitor on cell proliferation, we conducted MTT and CI was calculated to verify whether the combination of the two drugs had a synergistic effect (Figure 1A-1L). As shown in Figure 1A and Figure 1B, as the concentrations of the two drugs increased, the cell survival rate gradually decreased, showing anlotinib and PD-L1 growth inhibitor had inhibitory effects on MDA-MB-468 and BT549 cells.
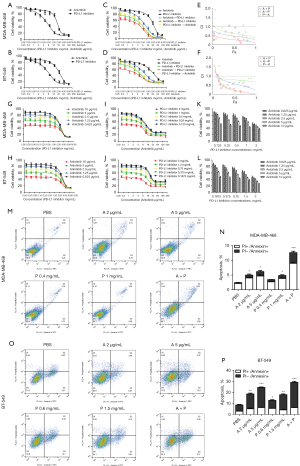
To further study the cell-killing effect of the combination of the two drugs, we conducted different methods of combined administration (Figure 1C,1D). The results showed the simultaneous administration of anlotinib and PD-L1 inhibitor, the addition of PD-L1 inhibitors after 6 hours of anlotinib, and the addition of anlotinib after 6 hours of PD-L1 inhibitor were all more effective in inhibiting cell survival than the single-agent group, and the sequential PD-L1 inhibitor of anlotinib had the strongest inhibitory effect on cell growth. The curves fitted by the CI values of the three combination schemes were between 0 and 1 (Figure 1E,1F). Therefore, it was concluded that the two drugs had a synergistic effect on the growth inhibition of MDA-MB-468 and BT-549 cells. The inhibitory effect was positively correlated with anlotinib or PD-L1 inhibitor concentration (Figure 1G-1L). According to the data, we calculated the IC50 values of anlotinib on MDA-MB-468 and BT-549 cells after 48 hours both as 10 µg/mL, and the PD-L1 inhibitor were 2 and 3 mg/mL, respectively.
To investigate the effect of anlotinib and PD-L1 inhibitor on apoptosis of MDA-MB-468 and BT-549 cell, we conducted cell apoptosis assay. As shown in Figure 1M,1N, in MDA-MB-468 cells, the apoptosis rate of the PBS group, anlotinib 2 µg/mL group and 5 µg/mL group, PD-L1 inhibitor 0.4 mg/mL group and 1 mg/mL group, and anlotinib 5 µg/mL combined with PD-L1 inhibitor 1 mg/mL group were 2.47%±0.56%, 5.01%±0.89%, 6.19%±1.35%, 3.48%±0.38%, 4.87%±0.84%, and 12.60%±1.40%, respectively. In BT-549 cells, the apoptosis rate of the PBS group, anlotinib 2 µg/mL group and 5 µg/mL group, PD-L1 inhibitor 0.6 mg/mL group and 1.5 mg/mL group, and anlotinib 5 µg/mL combined with PD-L1 inhibitor 1.5 mg/mL group were 8.67%±1.24%, 18.60%±2.50%, 24.56%±1.24%, 13.13%±0.96%, 17.94%±1.93%, and 29.07%±2.17%, respectively (Figure 1O,1P). All the treated groups showed significant apoptosis except for PD-L1 inhibitor 0.4 mg/mL group in MDA-MB-468 cells compared to the PBS group, and the combination group had the highest apoptosis rate. This showed both anlotinib and PD-L1 inhibitor could promote the apoptosis of MDA-MB-468 and BT-549 TNBC cells, and the combination group had a better effect on promoting cell apoptosis than the single-agent group.
Effects of anlotinib and PD-L1 inhibitor on the migration and invasion of MDA-MB-468 and BT-549 cells
Wound healing and Transwell experiments were used to detect the effect of drugs on the migration and invasion of MDA-MB-468 and BT-549 cells. As shown in Figure 2A,2B, after 24 hours of drug treatment the migration rate of MDA-MB-468 cells was 17.16%±1.01% in the PBS group, 12.26%±1.05% in the anlotinib 2 µg/mL group, and 6.53%±1.04% in the anlotinib 5 µg/mL group, while the PD-L1 inhibitor 0.4 and 1 mg/mL groups were 14.56%±0.87% and 7.62±0.78%, and anlotinib 5 µg/mL and PD-L1 inhibitor 1 mg/mL group was 4.08%±0.90%. The migration rate of BT-549 cells in the PBS group was 35.55%±0.53%, in the anlotinib 2 µg/mL group 31.46%±1.22%, in the anlotinib 5 µg/mL group 19.56%±0.63% , in the PD-L1 inhibitor 0.6 mg/mL group 32.39%±0.90%, in the PD-L1 inhibitor 1.5 mg/mL group 25.13%±0.93%, and in the anlotinib 5 µg/mL and PD-L1 inhibitor 1 mg/mL was 0.82%±0.48% (Figure 2C,2D). Compared with the PBS group, the migration rate of each group was statistically significant. The results suggested anlotinib and PD-L1 inhibitor could reduce the mobility and migration of MDA-MB-468 and BT-549 cells, and as the concentration of anlotinib and PD-L1 drugs increased, the migration of cells gradually suppressed, especially in the joint group.
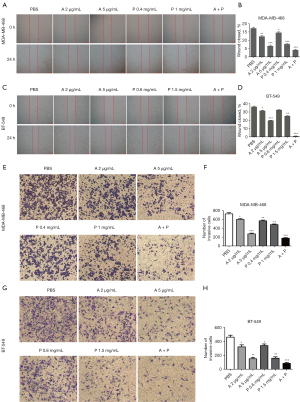
As shown in Figure 2E-2H, the results of the Transwell experiment showed that the number of MDA-MB-468 and BT-549 cells passing through the Transwell membrane decreased significantly compared with the PBS group as the concentration of anlotinib and PD-L1 inhibitor drugs increased. These results showed anlotinib and PD-L1 inhibitor could reduce the invasion ability of MDA-MB-468 and BT-549 cells, and the inhibitory effect of the combined group was better than that of the single-agent group.
Effects of anlotinib and PD-L1 inhibitor on the expression of PI3K, p-PI3K, AKT, p-AKT, and Bcl-xl
To further explore the molecular mechanism of drugs on the apoptosis of MDA-MB-468 and BT-549 cells, we investigated the expression of signaling molecules by Western blots. As shown in Figure 3A-3D, compared with the control group, both anlotinib and PD-L1 inhibitor inhibited the phosphorylation of PI3K and AKT protein in MDA-MB-468 and BT-549 cells, and inhibited the expression of Bcl-xl protein. However, no obvious effect was seen on the expression of PI3K and AKT protein. Based on these findings, we proposed a possible mechanism for the effects of anlotinib and PD-L1 inhibitor on cell apoptosis in breast cancer cells (Figure 3E).
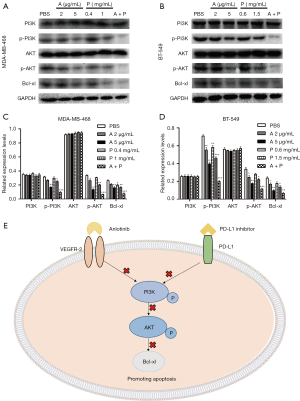
The effects of anlotinib and PD-L1 inhibitor in MDA-MB-468 TNBC xenograft models in vivo
As nude mice are immune-deficient and do not have T lymphocytes, we cultured and expanded the lymphocytes extracted from the fresh human blood of healthy experimental participants. We mixed T cells with MDA-MB-468 cells in a ratio of 1:4 and planted them in mice by subcutaneous injection of 0.2ml of suspension cell liquid (2.5×106 cells) to construct a mouse breast cancer transplantation tumor model. Group 1 was the PBS group, which was fed normally, and group 2 was the anlotinib group, which was given by gavage at a concentration of 5 mg/kg/day from day 1 until day 14 after inoculation. Group 3 was the PD-L1 inhibitor group, where 10 mg/kg was injected through the tail vein on day 0, day 2, and day 4 after inoculation, and group 4 was the anlotinib combined with PD-L1 inhibitor group. The results in Figure 4A-4D show the tumor volume in the PBS group increased significantly, the tumor volume growth rate was significantly higher than in the anlotinib group and the PD-L1 inhibitor group, and the tumor volume in the combined group was smaller than before the treatment. Compared with the control group, the anlotinib group, PD-L1 inhibitor group, and the combination group all had an inhibitory effect on the growth of mouse TNBC xenografts. The inhibitory effect of anlotinib was stronger than that of the PD-L1 inhibitor group, and the combination group had the most significant inhibitory effect on transplanted tumors.
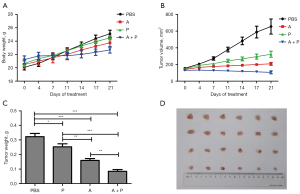
Discussion
Breast cancer ranks first in global cancer incidence, and the incidence rate is increasing (24). Standard systemic treatment includes chemotherapy and immunotherapy. PARP inhibitors are considered in BRCA mutated tumors (25). Immunodrug conjugates such as sacituzumab govitecan (antiTROP2 agent) and trastuzumab deruxtecan (for HER 2 expressing TNBC) are indicated in pretreated metastatic TNBC (26,27). The role of antiangiogenic drugs is controversial. Bevacizumab is approved in some countries but no longer in the United States for the treatment of metastatic TNBC. Anlotinib have shown certain efficacy in clinical studies of advanced TNBC (28-30). Anlotinib showed also anti-tumor effects in a variety of solid tumors (31-33). A trial showed that camrelizumab combined with anlotinib had favorable therapeutic effects and a manageable safety profile in patients with advanced TNBC (34). Recent studies have shown that anlotinib down-regulates Bcl-2 by inhibiting the VEGFR2/PI3K/AKT pathway, promoting apoptosis and inhibit liver cancer cell proliferation, and tumor progression (10,35-38). However, there are few studies that elucidated the mechanism how anlotinib work on TNBC. Bcl-xl is an anti-apoptotic gene where its decreased expression results in suppression of cell growth and proliferation, followed by apoptosis (39). Further, the PI3K/AKT pathway and the anti-apoptotic gene Bcl-xl are important in the process of cell apoptosis.
Immunotherapy has become a hot topic in TNBC research in recent years. PD-L1 can inhibit the expression of AKT and Bcl-2 and promote cell apoptosis (19,22,23), and since both anlotinib and PD-L1 inhibitor can act on the PI3K/AKT pathway in tumor cells, we speculated the two may promote tumor cell apoptosis through PI3K/AKT/Bcl-xl. Therefore, we conducted Western blot experiments, and the results support the hypothesis that anlotinib and PD-L1 inhibitor can further inhibit the activation of AKT and down-regulate the expression of Bcl-xl by inhibiting PI3K and activation and promoting the apoptosis of TNBC cells.
It is known that the expression of PD-L1 is related to the efficacy of PD-1/PD-L1 inhibitors, and the higher the level of PD-L1 expression, the greater the clinical benefit (40). Therefore, we detected the expression of PD-L1, and the results showed its expression in these two cells was positive (Figure S1). This shows PD-L1 inhibitors can bind to PD-L1 on the surface of cells to inhibit the downstream signaling pathways of tumor cells. The results of the MTT experiment showed the PD-L1 inhibitor could also inhibit the proliferation of TNBC tumor cells without the involvement of T lymphocytes. This also shows that in addition to regulating T cell immune response, PD-L1 can inhibit the proliferation of tumor cells by binding to PD-L1inhibitor. We also conducted three different methods of joint experiments, and the results showed anlotinib sequential PD-L1 inhibitor had the best killing effect on cells. Study has shown that inhibiting the activation of AKT can reduce the expression of PD-L1 (41). We speculate this is because anlotinib significantly inhibits the PI3K/AKT pathway, which reduces the expression of PD-L1, and the combination of PD-L1 inhibitors and PD-L1 further inhibits the PI3K/AKT pathway and enhances the inhibition effect.
We also conducted scratch, Transwell, and apoptosis experiments, and the results showed the application of anlotinib and PD-L1 inhibitor could inhibit migration, and invasion, and promote cell apoptosis of TNBC cells. In this study, the experimental results of these two TNBC cells showed anlotinib and PD-L1 inhibitor had good anti-tumor activity in vitro, and the combined anti-tumor effect of the two drugs was more significant.
Immunotherapy combined with anti-angiogenic drugs is expected to be synergistic, because immune checkpoint inhibitors transform the immune microenvironment to inhibit cancer growth, whereas anti-angiogenic drugs cut off the nutrient supply to the tumor at the same time. In addition to promoting tumor angiogenesis, the increased expression of VEGF directly inhibits the trafficking and proliferation of T lymphocytes, inhibits the differentiation and maturation of dendritic cells and antigen presentation, inhibit the activation of T cells and reduce the immune response to tumor cells (42,43). In addition, the overexpression of VEGF can promote the aggregation and proliferation of immunosuppressive cells, promote the formation of blood vessels in tumors, and lead to changes in the tumor microenvironment. This not only promotes immunosuppression locally, but also affects the immune system of the whole body (44-46). Inhibition of VEGF not only inhibits the formation of tumor blood vessels, but promotes the infiltration of tumor CD8+ T lymphocytes, up-regulates the expression of PD-L1, relieves the suppression of immune cells, and enhances the immunotherapy effect of tumors (45). PD-1/PD-L1 inhibitor can normalize tumor blood vessels by activating T cells, promoting the effect of anti-angiogenic drugs and enhancing the killing function of T cells (47). Several studies such as Mpower150 have shown immunotherapy and anti-angiogenesis therapy have a synergistic effect, and PD-1/PD-L1 inhibitor combined with anti-angiogenesis drugs show a synergistic effect in the treatment of TNBC (48-50). Therefore, the combination of immunotherapy and anti-angiogenesis has a synergistic effect in treatment.
This study also has certain limitations. Firstly, the PD-L1 expression of the MDA-MB-468 and BT-549 TNBC cell lines we selected were all positive, and there was no TNBC cell line control group with negative PD-L1 expression. Secondly, animal experiments did not involve blood draws and biochemical tests, the effects of the two drugs on the bone marrow hematopoietic system and liver and kidney function of mice were not analyzed, and we did not record the adverse reactions of combining the two drugs. Finally, anlotinib mainly acts on the AKT/PI3K pathway by inhibiting the phosphorylation of VEGFR-2, and the WB experiment did not detect the expression of PD-L1, VEGFR and its phosphorylation level.
In summary, based on the results of cell and animal experiments, this study found anlotinib and PD-L1 inhibitors had significant anti-tumor effects in TNBC cells, and the combined anti-tumor effect of the two drugs was better than that of single drugs. It also revealed that anlotinib and PD-L1 inhibitors promoted TNBC cell apoptosis by inhibiting the PI3K/AKT/Bcl-xl pathway.
Conclusions
Anlotinib and PD-L1 inhibitor can inhibit cell proliferation, migration, and invasion of TNBC and promote cell apoptosis, and the combination group has better anti-tumor effects in vivo and in vitro than the single agent group. The combination of anlotinib and PD-L1 inhibitor may promote apoptosis of TNBC cells through PI3K/AKT/Bcl-xl signaling pathways, which might offer potential clinical treatment roles for these.
Acknowledgments
We are grateful for the support of the Medical Science and Technology project of Henan province and Henan Key Laboratory of Cancer Epigenetics (Henan University of Science and Technology). The authors also appreciate the academic support from the AME Breast Cancer Collaborative Group.
Funding: This work was supported in part by grants from the Science and Technology Department of Henan Province (No. 2018010019). The funding agency only provided funds for experimental expenses, and did not participate in the design, implementation, analysis of data, or writing of manuscripts.
Footnote
Reporting Checklist: The authors have completed the ARRIVE reporting checklist. Available at https://atm.amegroups.com/article/view/10.21037/atm-22-6446/rc
Data Sharing Statement: Available at https://atm.amegroups.com/article/view/10.21037/atm-22-6446/dss
Conflicts of Interest: All authors have completed the ICMJE uniform disclosure form (available at https://atm.amegroups.com/article/view/10.21037/atm-22-6446/coif). All authors report that this work was supported in part by grants from the Science and Technology Department of Henan Province (No. 2018010019), and received receipt of materials (Anlotinib and PD-L1 inhibitor [SHR-1316 injection]) from Hengrui Medicine Co. Ltd for free. AO received consulting fees from Daiichi Sankyo, Novartis, Pfizer, Lilly, and Gilead; payment for lectures and presentations from Daiichi Sankyo, Novartis, Pfizer, Lilly, Gilead, and Amgen; support for attending meetings from Daiichi Sankyo, Novartis, Pfizer, Lilly, and Gilead; participation on a Data Safety Monitoring Board from Daiichi Sankyo, Novartis, Pfizer, Lilly, and Gilead. GJ reports grants, personal fees and non-financial support from Novartis, Roche, Pfizer, Lilly, Amgen, BMS, and Astra-Zeneca; personal fees from Abbvie, Daiichi-Sankyo, and Seagen; non-financial support from Medimmune and MerckKGaA outside the submitted work. The authors have no other conflicts of interest to declare.
Ethical Statement: The authors are accountable for all aspects of the work in ensuring that questions related to the accuracy or integrity of any part of the work are appropriately investigated and resolved. Experiments were performed under a project license granted by the Medical Ethics Committee of the First Affiliated Hospital of Henan University of Science and Technology, in compliance with the National institutes of Health Guide for the Care and Use of Laboratory Animals, 8th edition.
Open Access Statement: This is an Open Access article distributed in accordance with the Creative Commons Attribution-NonCommercial-NoDerivs 4.0 International License (CC BY-NC-ND 4.0), which permits the non-commercial replication and distribution of the article with the strict proviso that no changes or edits are made and the original work is properly cited (including links to both the formal publication through the relevant DOI and the license). See: https://creativecommons.org/licenses/by-nc-nd/4.0/.
References
- Deng S, Wang M, Wang C, et al. p53 downregulates PD-L1 expression via miR-34a to inhibit the growth of triple-negative breast cancer cells: a potential clinical immunotherapeutic target. Mol Biol Rep 2022; Epub ahead of print. [Crossref] [PubMed]
- Shadbad MA, Safaei S, Brunetti O, et al. A Systematic Review on the Therapeutic Potentiality of PD-L1-Inhibiting MicroRNAs for Triple-Negative Breast Cancer: Toward Single-Cell Sequencing-Guided Biomimetic Delivery. Genes (Basel) 2021;12:1206. [Crossref] [PubMed]
- Bräutigam K, Kabore-Wolff E, Hussain AF, et al. Inhibitors of PD-1/PD-L1 and ERK1/2 impede the proliferation of receptor positive and triple-negative breast cancer cell lines. J Cancer Res Clin Oncol 2021;147:2923-33. [Crossref] [PubMed]
- Chen J, Jiang P, Wang HJ, et al. The efficacy of molecular subtyping in predicting postoperative recurrence in breast-conserving therapy: a 15-study meta-analysis. World J Surg Oncol 2014;12:212. [Crossref] [PubMed]
- Zhang Z, Ji S, Zhang B, et al. Role of angiogenesis in pancreatic cancer biology and therapy. Biomed Pharmacother 2018;108:1135-40. [Crossref] [PubMed]
- Banerjee S, Dowsett M, Ashworth A, et al. Mechanisms of disease: angiogenesis and the management of breast cancer. Nat Clin Pract Oncol 2007;4:536-50. [Crossref] [PubMed]
- Kazerounian S, Lawler J. Integration of pro- and anti-angiogenic signals by endothelial cells. J Cell Commun Signal 2018;12:171-9. [Crossref] [PubMed]
- Lin B, Song X, Yang D, et al. Anlotinib inhibits angiogenesis via suppressing the activation of VEGFR2, PDGFRβ and FGFR1. Gene 2018;654:77-86. [Crossref] [PubMed]
- Sun Y, Niu W, Du F, et al. Safety, pharmacokinetics, and antitumor properties of anlotinib, an oral multi-target tyrosine kinase inhibitor, in patients with advanced refractory solid tumors. J Hematol Oncol 2016;9:105. [Crossref] [PubMed]
- Song F, Hu B, Cheng JW, et al. Anlotinib suppresses tumor progression via blocking the VEGFR2/PI3K/AKT cascade in intrahepatic cholangiocarcinoma. Cell Death Dis 2020;11:573. [Crossref] [PubMed]
- Breckenridge DG, Xue D. Regulation of mitochondrial membrane permeabilization by BCL-2 family proteins and caspases. Curr Opin Cell Biol 2004;16:647-52. [Crossref] [PubMed]
- Wang X, Zhang J, Kim HP, et al. Bcl-XL disrupts death-inducing signal complex formation in plasma membrane induced by hypoxia/reoxygenation. FASEB J 2004;18:1826-33. [Crossref] [PubMed]
- Lote H, Cafferkey C, Chau I. PD-1 and PD-L1 blockade in gastrointestinal malignancies. Cancer Treat Rev 2015;41:893-903. [Crossref] [PubMed]
- Lim JS, Sundar R, Chénard-Poirier M, et al. Emerging biomarkers for PD-1 pathway cancer therapy. Biomark Med 2017;11:53-67. [Crossref] [PubMed]
- Schmid P, Rugo HS, Adams S, et al. Atezolizumab plus nab-paclitaxel as first-line treatment for unresectable, locally advanced or metastatic triple-negative breast cancer (IMpassion130): updated efficacy results from a randomised, double-blind, placebo-controlled, phase 3 trial. Lancet Oncol 2020;21:44-59. [Crossref] [PubMed]
- Tian Y, Sun X, Cheng G, et al. The association of CMTM6 expression with prognosis and PD-L1 expression in triple-negative breast cancer. Ann Transl Med 2021;9:131. [Crossref] [PubMed]
- Qi Y, Yan X, Wang C, et al. Predictive value of PD-L1 expression to the efficacy of immune checkpoint inhibitors in advanced triple-negative breast cancer: A systematic review and meta-analysis. Front Pharmacol 2022;13:1004821. [Crossref] [PubMed]
- Saresella M, Rainone V, Al-Daghri NM, et al. The PD-1/PD-L1 pathway in human pathology. Curr Mol Med 2012;12:259-67. [Crossref] [PubMed]
- Azuma T, Yao S, Zhu G, et al. B7-H1 is a ubiquitous antiapoptotic receptor on cancer cells. Blood 2008;111:3635-43. [Crossref] [PubMed]
- Teng F, Meng X, Kong L, et al. Progress and challenges of predictive biomarkers of anti PD-1/PD-L1 immunotherapy: A systematic review. Cancer Lett 2018;414:166-73. [Crossref] [PubMed]
- Ghahremanloo A, Soltani A, Modaresi SMS, et al. Recent advances in the clinical development of immune checkpoint blockade therapy. Cell Oncol (Dordr) 2019;42:609-26. [Crossref] [PubMed]
- Chang CH, Qiu J, O'Sullivan D, et al. Metabolic Competition in the Tumor Microenvironment Is a Driver of Cancer Progression. Cell 2015;162:1229-41. [Crossref] [PubMed]
- Wu X, Li Y, Liu X, et al. B7-H1(PD-L1) confers chemoresistance through ERK and p38 MAPK pathway in tumor cells. bioRxiv 2018:308601.
- Sung H, Ferlay J, Siegel RL, et al. Global Cancer Statistics 2020: GLOBOCAN Estimates of Incidence and Mortality Worldwide for 36 Cancers in 185 Countries. CA Cancer J Clin 2021;71:209-49. [Crossref] [PubMed]
- Tutt ANJ, Garber JE, Kaufman B, et al. Adjuvant Olaparib for Patients with BRCA1- or BRCA2-Mutated Breast Cancer. N Engl J Med 2021;384:2394-405. [Crossref] [PubMed]
- Bardia A, Hurvitz SA, Tolaney SM, et al. Sacituzumab Govitecan in Metastatic Triple-Negative Breast Cancer. N Engl J Med 2021;384:1529-41. [Crossref] [PubMed]
- Modi S, Jacot W, Yamashita T, et al. Trastuzumab Deruxtecan in Previously Treated HER2-Low Advanced Breast Cancer. N Engl J Med 2022;387:9-20. [Crossref] [PubMed]
- Miles DW, Diéras V, Cortés J, et al. First-line bevacizumab in combination with chemotherapy for HER2-negative metastatic breast cancer: pooled and subgroup analyses of data from 2447 patients. Ann Oncol 2013;24:2773-80. [Crossref] [PubMed]
- Zhu A, Yuan P, Wang J, et al. Apatinib combined with chemotherapy in patients with previously treated advanced breast cancer: An observational study. Oncol Lett 2019;17:4768-78. [Crossref] [PubMed]
- Jiang YZ, Liu Y, Xiao Y, et al. Molecular subtyping and genomic profiling expand precision medicine in refractory metastatic triple-negative breast cancer: the FUTURE trial. Cell Res 2021;31:178-86. [Crossref] [PubMed]
- Shen G, Zheng F, Ren D, et al. Anlotinib: a novel multi-targeting tyrosine kinase inhibitor in clinical development. J Hematol Oncol 2018;11:120. [Crossref] [PubMed]
- Wang G, Sun M, Jiang Y, et al. Anlotinib, a novel small molecular tyrosine kinase inhibitor, suppresses growth and metastasis via dual blockade of VEGFR2 and MET in osteosarcoma. Int J Cancer 2019;145:979-93. [Crossref] [PubMed]
- Ruan X, Shi X, Dong Q, et al. Antitumor effects of anlotinib in thyroid cancer. Endocr Relat Cancer 2019;26:153-64. [Crossref] [PubMed]
- Liu J, Liu Q, Li Y, et al. Efficacy and safety of camrelizumab combined with apatinib in advanced triple-negative breast cancer: an open-label phase II trial. J Immunother Cancer 2020;8:e000696. [Crossref] [PubMed]
- He C, Wu T, Hao Y. Anlotinib induces hepatocellular carcinoma apoptosis and inhibits proliferation via Erk and Akt pathway. Biochem Biophys Res Commun 2018;503:3093-9. [Crossref] [PubMed]
- Beck JT, Ismail A, Tolomeo C. Targeting the phosphatidylinositol 3-kinase (PI3K)/AKT/mammalian target of rapamycin (mTOR) pathway: an emerging treatment strategy for squamous cell lung carcinoma. Cancer Treat Rev 2014;40:980-9. [Crossref] [PubMed]
- Jeong SJ, Pise-Masison CA, Radonovich MF, et al. Activated AKT regulates NF-kappaB activation, p53 inhibition and cell survival in HTLV-1-transformed cells. Oncogene 2005;24:6719-28. [Crossref] [PubMed]
- Daragmeh J, Barriah W, Saad B, et al. Analysis of PI3K pathway components in human cancers. Oncol Lett 2016;11:2913-8. [Crossref] [PubMed]
- Safdari Y, Khalili M, Ebrahimzadeh MA, et al. Natural inhibitors of PI3K/AKT signaling in breast cancer: emphasis on newly-discovered molecular mechanisms of action. Pharmacol Res 2015;93:1-10. [Crossref] [PubMed]
- Patel SP, Kurzrock R. PD-L1 Expression as a Predictive Biomarker in Cancer Immunotherapy. Mol Cancer Ther 2015;14:847-56. [Crossref] [PubMed]
- Cha JH, Chan LC, Li CW, et al. Mechanisms Controlling PD-L1 Expression in Cancer. Mol Cell 2019;76:359-70. [Crossref] [PubMed]
- Voron T, Colussi O, Marcheteau E, et al. VEGF-A modulates expression of inhibitory checkpoints on CD8+ T cells in tumors. J Exp Med 2015;212:139-48. [Crossref] [PubMed]
- Gabrilovich D, Ishida T, Oyama T, et al. Vascular endothelial growth factor inhibits the development of dendritic cells and dramatically affects the differentiation of multiple hematopoietic lineages in vivo. Blood 1998;92:4150-66. [Crossref] [PubMed]
- Maenhout SK, Thielemans K, Aerts JL. Location, location, location: functional and phenotypic heterogeneity between tumor-infiltrating and non-infiltrating myeloid-derived suppressor cells. Oncoimmunology 2014;3:e956579. [Crossref] [PubMed]
- Huang Y, Goel S, Duda DG, et al. Vascular normalization as an emerging strategy to enhance cancer immunotherapy. Cancer Res 2013;73:2943-8. [Crossref] [PubMed]
- Huang Y, Yuan J, Righi E, et al. Vascular normalizing doses of antiangiogenic treatment reprogram the immunosuppressive tumor microenvironment and enhance immunotherapy. Proc Natl Acad Sci U S A 2012;109:17561-6. [Crossref] [PubMed]
- Huang Y, Kim BYS, Chan CK, et al. Improving immune-vascular crosstalk for cancer immunotherapy. Nat Rev Immunol 2018;18:195-203. [Crossref] [PubMed]
- Socinski MA, Jotte RM, Cappuzzo F, et al. Atezolizumab for First-Line Treatment of Metastatic Nonsquamous NSCLC. N Engl J Med 2018;378:2288-301. [Crossref] [PubMed]
- Wang Q, Gao J, Di W, et al. Anti-angiogenesis therapy overcomes the innate resistance to PD-1/PD-L1 blockade in VEGFA-overexpressed mouse tumor models. Cancer Immunol Immunother 2020;69:1781-99. [Crossref] [PubMed]
- Li Q, Wang Y, Jia W, et al. Low-Dose Anti-Angiogenic Therapy Sensitizes Breast Cancer to PD-1 Blockade. Clin Cancer Res 2020;26:1712-24. [Crossref] [PubMed]
(English Language Editor: B. Draper)