Effect of Aspergillus fumigatus on infection in immunosuppressed rats
Highlight box
Key findings
• The increased expression of the NLRP3/caspase-1/GSDMD pathway was associated with susceptibility to invasive Aspergillus fumigatus infection in severely immunosuppressed rats.
What is known and what is new?
• The classical pathway of cell death, NLRP3/caspase-1/GSDMD, is highly expressed in sepsis patients and animals, and the use of related blockers of this pathway or the knockout of the genes of these factors can reduce the systemic inflammatory response, and alleviate organ damage to some extent.
• IPA is a serious, life-threatening lung infection occurring primarily in patients with severe immunodeficiency. Our findings highlight the role of the NLRP3/caspase -1/GSDMD pyrogenic pathway in IPA.
What is the implication, and what should change now?
• NLRP3/caspase-1/GSDMD involved in the pathogenesis of pulmonary aspergillosis has been identified, and it is necessary to continue to explore the application of pathway blockers as disease prevention.
Introduction
Invasive pulmonary aspergillosis (IPA) is a serious life-threatening lung infection occurring primarily in patients with severe immunodeficiency. When the host is immunocompromised or the number of inhaled spores exceeds the clearance ability of airway defense barrier, Aspergillus fumigatus spores can invade the body and lead to systemic infection. According to the latest epidemiological survey of invasive fungal diseases in China, Aspergillus infection accounted for 37.9% of all confirmed cases of pulmonary fungal infection, and was the most common pathogenic fungus (1,2).
In recent years, due to the widespread use of broad-spectrum antibiotics, anticancer drugs and immunosuppressive agents, invasive diagnosis and treatment technologies, increased organ transplantation, increasing numbers of AIDS patients, aging populations and various other reasons, the incidence rate of IPA has increased dramatically (3-5), and the mortality rate is high at 80–90% (6).
Aspergillus fumigatus is a ubiquitous, opportunistic saprophytic fungal pathogen that frequently invades the upper and lower respiratory tracts of immunocompromised individuals (7-9), resulting in severe pulmonary disease (10,11). About 95.2% of the patients received medical treatment and 4.8% received surgical treatment, the patients who underwent operation were mainly lung cancer, organ transplantation, liver disease, human immunodeficiency virus (HIV) infection, blood disease, chronic lung disease and so on. The mortality of these patients was higher (12). Both cyclophosphamide (CTX) and dexamethasone (DXM) are crucial immunosuppressive agents widely used in clinical settings to mitigate complications and death (13). The combination of DXM, CTX, etoposide, and cisplatin is less toxic and has been shown to be more effective than high-dose CTX for peripheral stem cell mobilization in multiple myeloma (14).
Pyroptosis differs from apoptosis and other cell death modes as it is an inflammatory form of cell death, which can lead to sepsis if overactivated (15,16). A growing body of evidence suggests the factors involved in the classic pathway NLRP3/caspase-1/GSDMD process of focal death are highly expressed in people and animals with sepsis, and the use of related blockers of factors in this pathway or the knockout of genes of these factors can reduce the systemic inflammatory response, and alleviate organ damage to some extent (17-19).
To further elucidate the role of the pyroptosis pathway, NLRP3-caspase-1-GSDMD, we established rat models of immunosuppression and IPA to provide a new treatment for patients with IPA and immunosuppressive patients. We present the following article in accordance with the ARRIVE reporting checklist (available at https://atm.amegroups.com/article/view/10.21037/atm-22-6600/rc).
Methods
Experimental animals
A total of 85 male pathogen-free Sprague-Dawley (SD) rats aged 8 weeks old weighing (200±20 g) were provided by Zhejiang Wei Tong Li Hua Experimental Animal Technology Co., Ltd. (animal license number: scxk; Zhejiang; 2019-0001).
The animals were housed under standard conditions and allowed access to food and water ad libitum. Animal experiments procedures were approved by the Animal Research Ethics Committee of Guangxi Zhuoqiang Technology Co. Ltd (No. ZQIA-2022-017), in compliance with Animal Research Ethics Committee of Guangxi Zhuoqiang Technology Co. Ltd guidelines for the care and use of animals. A protocol was prepared before the study without registration.
Experimental strains and drugs
Aspergillus fumigatus strain (CGMCC No. 3.15720), DXM (Merck, D022, 0.6 mg/mL, intramuscular (i.m.) injection, 0.6 mg/kg), CTX (Sigma, lrac0295, 15 mg/mL, intraperitoneal (i.p.) injection, 37.5 mg/kg), normal saline and glucose (Biologics, g708ba0050), peptone (Biologics, G 921ba0010), yeast extract (Biologics, G 916ba0009), and agar (Biologics, g828ba0005).
Equipment
XISEN Meikang automatic biochemical analyzer (XT-2000i automatic five classification blood cell analyzer (Sysmex, XISEN Meikang Co., Ltd., Japan); flow cytometer (Beckman Coulter, USA, cytoflex s); real-time polymerase chain reaction (RT-PCR) instrument (Applied Biosystems 7500, America); Ultra Micro Nucleic Acid Analyzer (Nano-400, Aosen, Hangzhou, China).
Strain culture
Culture medium composition: 1 g glucose, 1 g peptone and 0.5 g yeast extract were added to 100 mL distilled water, mixed evenly, sub packed into 15 mL glass test tubes, sealed with a cotton stopper of appropriate size, and wrapped with kraft paper for sterilization.
Resurrected strains: 0.3 mL of liquid culture medium was added to the purchased strain ampoules, and the bacterial solution was then placed in two test tubes containing culture medium, and incubated in a constant temperature incubator at 25 ℃ for 5 days.
Strain amplification and colony count: 30 µL of the resurrected strain was placed in a test tube containing liquid culture medium and 25 ℃ cultured for 5 days. Next, 10 µL of bacterial solution was diluted 103-fold in a 106-fold gradient, after which 10 µL of bacterial solution was inoculated into sabouraud medium. The colony count was calculated after culturing for 2–3 days. The remaining bacterial solution was maintained at 4 ℃ as standby.
Preparation of colony suspension: cultured colonies were centrifuged at 1,006 ×g for 15 min, and the precipitation was diluted to 1.0×107/mL according to the count concentration.
Experimental groups
The 85 male SD rats were randomly divided into four groups after 1-week acclimation: (I) normal control group (n=15; NS) 3 mL 0.9% saline was administered per kilogramfor 3 days, and then 0.1ml 0.9% saline dripped through the nose on the 4th and 5th days; (II) Immunosuppressed group (n=20; DXM + CTX) 0.3 mg/kg DXM i.m. + 37.5 mg/kg CTX i.p. administered for 3 days, followed by 0.1 mL 0.9% saline nasal drip on the 4th and 5th days; (III) normal infection group (n=20; AF, Aspergillus fumigatus), 3 mL 0.9% saline was administered per kilogram continuously for 3 days, and 0.1 mL 1.0×107 colony-forming units (CFU)/mL spore suspension dripped through the nose on the 4th and 5th days; (IV) Immunosuppressed infection group: (n=30; DXM + CTX + AF) 0.3 mg/kg DXM i.m. + 37.5 mg/kg CTX i.p. administered for 3 days, followed by nasal drip with 0.1 mL 1.0×107 CFU/mL s pore suspension on the 4th and 5th days. Lung tissue and blood were sampled at five separate time points 10 days apart. All of the rats lost >25% of their original body weight and were humanely killed.
Blood biochemical indexes
Two mL venous blood was collected and injected into the collecting vessel containing ETDA anticoagulant. After collecting, the blood was mixed upside down for 3–5 times. The samples were mixed and detected white blood cells (WBC)/red blood cells (RBC)/platelets (PLT) were detected by whole blood classification in 2 hours through XT-2000i automatic five classification blood cell analyzer.
Flow cytometry
For FCM, 100 µL whole blood sample in anticoagulant was obtained, 1µg corresponding antibody solution was added, and the mixture incubated at room temperature in the dark for 30 min, following which erythrocyte lysate was added, the product was mixed well and left to stand in the dark for 10 min prior to centrifugation. The supernatant was discarded and 500 µL phosphate-buffered saline added to the remaining pellet, which was centrifuged again, the supernatant discarded again, and the washing procedure repeated twice. Following resuspension in 500 µL of flow loading buffer, the proportion of T lymphocyte (LYMPH) subsets CD3+, CD4+, and CD8+ was analyzed (20).
Quantitative polymerase chain reaction (q-PCR)
Total RNA extraction: 50–80 mg lung tissue samples had 1 mL Trizol ice homogenate added, maintained at room temperature for 5 min before 0.2 mL of 1-bromo-3-chloropropane was added, then vortexed and mixed well, maintained at room temperature for 3 min, followed by centrifugation at 12,000 g at 4 ℃ for 15 min. Next, 500 µL of absorbed colorless supernatant was placed in a new centrifuge tube, 0.5 mL isopropanol was added and the tube gently inverted 10 times to mix before allowing precipitation at room temperature for 10 min and prior to a final 12,000 g chilled 4 ℃ centrifugation for 10 min, following which the supernatant was discarded. Next, 1 mL of 75% ethanol prepared with diethylpyrocarbonate (DEPC) water was added, vortexed and mixed well before 7,500 g chilled 4 ℃ centrifugation for 5 min, discarding of the supernatant and washing twice. The RNA was allowed to dry slightly, then 20 µL DEPC dissolved in water was added. Nano 400 determines RNA 260/230 and 260/280, and RNA purity was detected. The cDNA obtained by reverse transcription was detected and analyzed by fluorescence quantitative PCR using ABI 7500 (Table 1) (21,22).
Table 1
Name | Sense/antisense (S/AS) | Sequence (5'-3') |
---|---|---|
GAPDH | S | GGCAAGTTCAACGGCACAG |
AS | CGCCAGTAGACTCCACGACAT | |
NLRP3 | S | GACCAGCCAGAGTGGAATGATG |
AS | GCTGGGTGTAGCGTCTGTTGAG | |
Caspase-1 | S | TCCAGGAGGGAATATGTGGG |
AS | TGATAACCTTGGGCTTGTCTTT | |
GSDMD | S | CATGACTTTAGTCTGCTTGCCGTAC |
AS | TCCTGTAAAATCCTCCCGATGTCT |
RT-PCR, real-time polymerase chain reaction.
Western blotting
The protein content was evaluated using the bicinchoninic acid commercial protein assay kit (Beyotime Biotechnology, Jiangsu, China). The transferred membrane was blocked with 5% skimmed milk for 2 h at room temperature, then incubated with the primary antibody overnight at 4 ℃. After incubation with the horseradish peroxidase-conjugated secondary antibody for 1 h at room temperature, the immunoblotting signals were visualized using a western luminescent detection kit (23,24).
Histopathological examination
Four rat lungs per group were randomly sampled and fixed in 4% paraformaldehyde, processed into paraffin blocks and a microtome was used to cut thin paraffin slices; xylene was used for dewaxing and an alcohol solution for hydration. Hematoxylin and eosin staining (Beyotime, Shanghai, China) was performed to discern the morphology of the lung tissue samples: the cell nucleus was stained with hematoxylin and the cytoplasm with eosin. The lung tissue samples were also evaluated under light microscopy. The pathological changes were assessed by a pathologist blinded to the allocation groups. Lesions (interstitial and alveolar edema, cellular infiltration, airway epithelial-cell damage, hemorrhage) were graded from 0 (normal), 1 (minimal), 2 (mild), 3 (moderate), 4 (severe), to 5 (very severe) (25).
ELISA
On day 10, rat serum samples were analyzed for inflammatory cytokines, including interleukin (IL)-1β, IL-8, and tumor necrosis factor (TNF)-α, using a commercially available sandwich enzyme-linked immunosorbent assay kit (ELISA Set, mlbio, Shanghai, China) specific for each cytokine processed according to the manufacturer’s instructions.
Statistical analysis
Significance testing was performed using the two-tailed paired Student’s t-test or one-way repeated measures analysis of variance with Dunnett’s post-hoc test. All data are presented as mean ± standard deviation. Statistical significance was set at P<0.05. Data were analyzed and visualized using Microsoft Excel and GraphPad Prism 6.
Results
Pathological examination
The alveolar structure of the control group was complete, the alveolar septum was not widened, there was no obvious edema in the pulmonary stroma, and there were no inflammatory cells or fluid exudation in the alveolar cavities. Pathological changes including pulmonary edema, neutrophil infiltration and bleeding were observed in both the immunosuppressed and normal infection groups. In the immunosuppressed infection group, the alveolar structure was seriously damaged, the alveolar and pulmonary interstitium were seriously edematous, the alveolar septum significantly thickened, and extensive inflammatory cell infiltration was observed, with pulmonary capillary blood flow and bleeding changes. The histopathological lung score showed the severity of injury in the immunosuppressed group, normal infection group and immunosuppressed infection group, which differed significantly from the normal group, and the injury in the immunosuppressed infection group was the most severe. The results are shown in Figure 1.
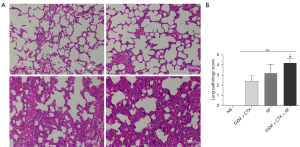
Blood biochemical indexes
The number of WBC in the DXM + CTX + AF and AF groups increased significantly compared with the NS group, and the number of WBC in the DXM + CTX + AF and DXM + CTX groups also increased significantly. The values for RBC, hemoglobin (Hb) decreased and PLT increased Compared with the NS group, platelets hematocrit (PCT), neutrophils (NEUT) and eosinophils (EO) in the DXM + CTX and DXM + CTX + AF groups were significantly increased. Cell types observed increased across the groups, with notable exceptions; the changes in LYMPH were consistent with the flow cytometry measurements. The results are shown in Table 2.
Table 2
Group | WBC (109/L) | RBC (1012/L) | Hb (g/L) | PLT (109/L) | PCT (%) | NEUT (%) | LYMPH (%) | MONO (%) | EO (%) |
---|---|---|---|---|---|---|---|---|---|
NS | 8.28±2.90 | 7.20±0.59 | 146.00±11.58 | 982.80±67.43 | 0.86±0.08 | 19.02±4.00 | 80.36±4.20 | 0.30±0.16 | 0.42±0.30 |
DXM + CTX | 6.67±1.23 | 6.21±0.53a | 130.40±9.35 | 2,348.40±383.91aa | 2.24±0.36aa | 59.72±1.60aa | 37.20±3.15aa | 0.20±0.12 | 2.70±1.90a |
AF | 9.52±1.50a | 7.49±0.39## | 149.40±6.62# | 935.00±122.92## | 0.85±0.11## | 21.65±3.23## | 65.00±21.85# | 0.40±0.00 | 0.75±0.41 |
DXM + CTX + AF | 16.85±5.53a# | 6.47±0.28* | 133.33±2.62* | 2,502.00±333.72aa** | 2.28±0.42aa** | 59.60±6.96aa** | 37.03±7.81aa* | 0.30±0.17 | 2.07±1.45 |
Compared with the NS group, aP<0.05, aaP<0.01; compared with DXM + CTX group, #P<0.05, ##P<0.01; compared with AF group, *P<0.05, **P<0.01, n=12. NS, control group; DXM + CTX, immunosuppressed group; AF, normal infection group; DXM + CTX + AF, immunosuppressed infection group. WBC, white blood cell; RBC, red blood cell; Hb, hemoglobin; PLT, platelets; PCT, platelets hematocrit; NEUT, neutrophils; LYMPH, lymphocyte; MONO, Monocytes; EO, eosinophils; NS, normal saline; DXM, dexamethasone; CTX, cyclophosphamide; AF, Aspergillus fumigatus.
Determination of inflammatory factors
Compared with the NS group, IL-1β in the AF and DXM + CTX + AF groups was significantly higher; increased significantly in the AF and DXM + CTX + AF groups compared with the DXM +CTX group; the DXM + CTX + AF was significantly different from the AF group. IL-8 increased significantly in the DXM + CTX, AF and DXM + CTX + AF groups when compared with the NS group. Significant increases in TNF-α in the DXM + CTX, AF, and DXM + CTX + AF groups were observed when compared with the NS group, results are shown in Figure 2.

q-PCR
The results revealed significantly higher transcription of NLRP3, caspase-1 and Gasdermin D (GSDMD) in the AF and DXM + CTX + AF groups than in the NS and DXM + CTX groups. Additionally, a non-significant increase in the DXM + CTX group was observed. The transcription of NLRP3 and caspase-1 specifically was significantly higher in the DXM + CTX + AF group than in the AF group. The results are shown in Figure 3.
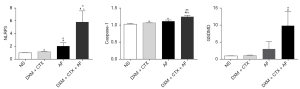
Western blotting
From the results, the expressions of NLRP3, caspase-1 and GSDMD in the AF and DXM + CTX + AF groups were significantly higher than in the NS and DXM + CTX groups. A non-significant increase was noted in the DXM + CTX group. Expressions of NLRP3 and caspase-1 in the DXM + CTX + AF group were significantly higher than in the AF group. The results are shown in Figure 4.
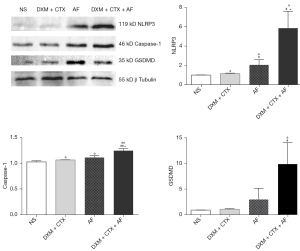
CD3+, CD4+, and CD8+ content
There were four notable results. (I) CD3+ content in DXM + CTX + AF group decreased significantly when compared with the NS group; similarly the content in the DXM + CTX + AF group decreased significantly when compared with the AF group. (II) CD4+ content in the DXM + CTX + AF increased significantly when compared with the NS group. (III) CD8+ content in the DXM + CTX + AF was markedly lower than that of the NS and DXM + CTX groups. (IV) CD4+/CD8+ ratio in the DXM + CTX + AF group was significantly higher than in the NS and DXM + CTX groups. The results are shown in Figure 5.
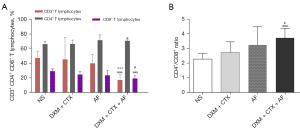
Discussion
Aspergillosis has emerged as one of the most common infectious causes of death in severely immunocompromised patients (26-29). Our study demonstrated that DXM + CTX + AF treatment increased the expression of proinflammatory cytokines and chemokines such as IL-1β, IL-8 and TNF-α, and these results were strongly associated with lung injury score results, and neutrophil infiltration, indicating that DXM + CTX + AF treatment can increase lung infection injury by inducing the expression of proinflammatory mediators.
Characteristic pathological changes in leukocytes, TNF-α, and inflammatory factors such as IL-8 are the most common response to diseases. In our experimental results, the pathological changes in the lung tissue samples from the DXM + CTX + AF rats were more severe than in the AF or DXM + CTX groups, highlighting the need for prevention of IPA in patients on immunosuppressive regimens.
The NLRP3 inflammasome is a widely studied macromolecular multiprotein complex mainly composed of NLRP3, and the apoptosis-related dot-like protein, caspase-1 (30). NLRP3 can mediate the host’s immune response to microbial infection and potentially reduce cell damage. When pathogen- or cell damage-related molecules bind to the corresponding cell receptors, nuclear factor kappa B (NF-κB) or reactive oxygen species are activated, which in turn activate NLRP3 (31). Activation of NLRP3 activates caspase-1, followed by IL-1β and IL-8 activation, resulting in inflammation. Caspase is important in the regulation of the classical pathway of cellular pyrogens (32). The process of cell scorch death or pyroptosis, performed by the GSDMD protein (33,34), is a type of inflammatory programmed cell death. Pyroptosis includes activation of the classical and nonclassical pathways of caspase-dependent cell death (4,5,11). NLRP3 is activated by a myriad of molecules, bacteria and viruses during the classical activation pathway of pyroptosis (35). Recruitment of activated caspase precursors into active caspase cleaves the GSDMD protein and forms pore domains at the exposed ends (23,24). Peptides containing the amino terminal active domain of GSDMD induce cell rupture, lead to cell scorch death and release of IL-1β, IL-18 and other material that induce an inflammatory reaction. Conversely, activated caspase-1 has no effect on precursor IL-1β; IL-18 is excised to form active IL-1β and IL-18; after being released, the extracellular factors lead to inflammatory cell damage. In the present experiment, after rats were infected with Aspergillus fumigatus, the NLRP3/caspase-1/GSDMD pathway was activated, and inflammatory protein expression and immunosuppression increased significantly.
In conclusion, infection with Aspergillus fumigatus increased the pathological score of lung tissue damage, significantly changed routine blood indices, and also significantly progressed the deterioration in the health of immunosuppressed rats. This mechanism has a role in the upregulation of the focal cell death pathway of NLRP3/caspase-1/GSDMD expression. Further studies are necessary to elucidate the correlation between Aspergillus fumigatus and DXM + CTX therapy in future therapeutic recommendations.
Conclusions
In immunosuppressed rats, Aspergillus fumigatus induced inflammatory reaction. The most severe pathological changes were related to the enhanced expression of NLRP3/caspase-1/GSDMD pathway.
Acknowledgments
Funding: This research was supported by the Guangxi Zhuang Autonomous Region Nanning Science Research and technology development programme (No. 20183039-5) and the Nanning Talent Guangxi Zhuang Autonomous Region Programme – LRB (No. 2018014).
Footnote
Reporting Checklist: The authors have completed the ARRIVE reporting checklist. Available at https://atm.amegroups.com/article/view/10.21037/atm-22-6600/rc
Data Sharing Statement: Available at https://atm.amegroups.com/article/view/10.21037/atm-22-6600/dss
Conflicts of Interest: All authors have completed the ICMJE uniform disclosure form (available at https://atm.amegroups.com/article/view/10.21037/atm-22-6600/coif). YY is an employee of Guangxi Zhuoqiang Technology Co., Ltd. The other authors have no conflicts of interest to declare.
Ethical Statement: The authors are accountable for all aspects of the work in ensuring that questions related to the accuracy or integrity of any part of the work are appropriately investigated and resolved. Animal experiments procedures were approved by the Animal Research Ethics Committee of Guangxi Zhuoqiang Technology Co., Ltd. (No. ZQIA-2022-017), in compliance with Animal Research Ethics Committee of Guangxi Zhuoqiang Technology Co., Ltd. guidelines for the care and use of animals.
Open Access Statement: This is an Open Access article distributed in accordance with the Creative Commons Attribution-NonCommercial-NoDerivs 4.0 International License (CC BY-NC-ND 4.0), which permits the non-commercial replication and distribution of the article with the strict proviso that no changes or edits are made and the original work is properly cited (including links to both the formal publication through the relevant DOI and the license). See: https://creativecommons.org/licenses/by-nc-nd/4.0/.
References
- Ledoux MP, Guffroy B, Nivoix Y, et al. Invasive Pulmonary Aspergillosis. Semin Respir Crit Care Med 2020;41:80-98. [Crossref] [PubMed]
- Russo A, Tiseo G, Falcone M, et al. Pulmonary Aspergillosis: An Evolving Challenge for Diagnosis and Treatment. Infect Dis Ther 2020;9:511-24. [Crossref] [PubMed]
- Gersuk GM, Underhill DM, Zhu L, et al. Dectin-1 and TLRs permit macrophages to distinguish between different Aspergillus fumigatus cellular states. J Immunol 2006;176:3717-24. [Crossref] [PubMed]
- Monforte V, Ussetti P, López R, et al. Nebulized liposomal amphotericin B prophylaxis for Aspergillus infection in lung transplantation: pharmacokinetics and safety. J Heart Lung Transplant 2009;28:170-5. [Crossref] [PubMed]
- Monforte V, Ussetti P, Gavaldà J, et al. Feasibility, tolerability, and outcomes of nebulized liposomal amphotericin B for Aspergillus infection prevention in lung transplantation. J Heart Lung Transplant 2010;29:523-30. [Crossref] [PubMed]
- Balloy V, Chignard M. The innate immune response to Aspergillus fumigatus. Microbes Infect 2009;11:919-27. [Crossref] [PubMed]
- Denning DW, Anderson MJ, Turner G, et al. Sequencing the Aspergillus fumigatus genome. Lancet Infect Dis 2002;2:251-3. [Crossref] [PubMed]
- van de Veerdonk FL, Gresnigt MS, Romani L, et al. Aspergillus fumigatus morphology and dynamic host interactions. Nat Rev Microbiol 2017;15:661-74. [Crossref] [PubMed]
- Posch W, Blatzer M, Wilflingseder D, et al. Aspergillus terreus: Novel lessons learned on amphotericin B resistance. Med Mycol 2018;56:73-82. [Crossref] [PubMed]
- Chandorkar P, Posch W, Zaderer V, et al. Fast-track development of an in vitro 3D lung/immune cell model to study Aspergillus infections. Sci Rep 2017;7:11644. [Crossref] [PubMed]
- Fraczek MG, Chishimba L, Niven RM, et al. Corticosteroid treatment is associated with increased filamentous fungal burden in allergic fungal disease. J Allergy Clin Immunol 2018;142:407-14. [Crossref] [PubMed]
- Patel DC, Bhandari P, Epstein DJ, et al. Surgical resection for patients with pulmonary aspergillosis in the national inpatient sample. J Thorac Dis 2021;13:4977-87. [Crossref] [PubMed]
- Trevisini S, Trevisan G, Zalaudek I, et al. Adamantiades-Behçet's disease therapy: current treatment options and recommendations with regard to the COVID-19 pandemic. Dermatol Ther 2022;35:e15286. [PubMed]
- Corso A, Arcaini L, Caberlon S, et al. A combination of dexamethasone, cyclophosphamide, etoposide, and cisplatin is less toxic and more effective than high-dose cyclophosphamide for peripheral stem cell mobilization in multiple myeloma. Haematologica 2002;87:1041-5. [PubMed]
- Pu Q, Gan C, Li R, et al. Atg7 Deficiency Intensifies Inflammasome Activation and Pyroptosis in Pseudomonas Sepsis. J Immunol 2017;198:3205-13. [Crossref] [PubMed]
- Aglietti RA, Dueber EC. Recent Insights into the Molecular Mechanisms Underlying Pyroptosis and Gasdermin Family Functions. Trends Immunol 2017;38:261-71. [Crossref] [PubMed]
- Fisher CJ Jr, Slotman GJ, Opal SM, et al. Initial evaluation of human recombinant interleukin-1 receptor antagonist in the treatment of sepsis syndrome: a randomized, open-label, placebo-controlled multicenter trial. Crit Care Med 1994;22:12-21. [Crossref] [PubMed]
- Boost KA, Hoegl S, Hofstetter C, et al. Targeting caspase-1 by inhalation-therapy: effects of Ac-YVAD-CHO on IL-1 beta, IL-18 and downstream proinflammatory parameters as detected in rat endotoxaemia. Intensive Care Med 2007;33:863-71. [Crossref] [PubMed]
- Li P, Allen H, Banerjee S, et al. Mice deficient in IL-1 beta-converting enzyme are defective in production of mature IL-1 beta and resistant to endotoxic shock. Cell 1995;80:401-11. [Crossref] [PubMed]
- Liu A, Wang Q, Yang X, et al. Cord blood CD4(+)/CD25(+) T cells down-regulates NKG2D on cytokine-induced killer cells. Front Biosci (Landmark Ed) 2019;24:1259-70. [Crossref] [PubMed]
- Xu Y, Wang Q, Li D, et al. Protective effect of lithium chloride against hypoglycemia-induced apoptosis in neuronal PC12 cell. Neuroscience 2016;330:100-8. [Crossref] [PubMed]
- Wang Q, Yang W, Zhang J, et al. TREM2 Overexpression Attenuates Cognitive Deficits in Experimental Models of Vascular Dementia. Neural Plast 2020;2020:8834275. [Crossref] [PubMed]
- Xu Y, Wang Q, Wu Z, et al. The effect of lithium chloride on the attenuation of cognitive impairment in experimental hypoglycemic rats. Brain Res Bull 2019;149:168-74. [Crossref] [PubMed]
- Du X, Xu Y, Chen S, et al. Inhibited CSF1R Alleviates Ischemia Injury via Inhibition of Microglia M1 Polarization and NLRP3 Pathway. Neural Plast 2020;2020:8825954. [Crossref] [PubMed]
- Pirat A, Zeyneloglu P, Aldemir D, et al. Pretreatment with simvastatin reduces lung injury related to intestinal ischemia-reperfusion in rats. Anesth Analg 2006;102:225-32. [Crossref] [PubMed]
- Latgé JP, Chamilos G. Aspergillus fumigatus and Aspergillosis in 2019. Clin Microbiol Rev 2019;33:e00140-18. [Crossref] [PubMed]
- Brown GD, Denning DW, Gow NA, et al. Hidden killers: human fungal infections. Sci Transl Med 2012;4:165rv13. [Crossref] [PubMed]
- Lortholary O, Gangneux JP, Sitbon K, et al. Epidemiological trends in invasive aspergillosis in France: the SAIF network (2005-2007). Clin Microbiol Infect 2011;17:1882-9. [Crossref] [PubMed]
- Azie N, Neofytos D, Pfaller M, et al. The PATH (Prospective Antifungal Therapy) Alliance® registry and invasive fungal infections: update 2012. Diagn Microbiol Infect Dis 2012;73:293-300. [Crossref] [PubMed]
- Bae JY, Lee SW, Shin YH, et al. P2X7 receptor and NLRP3 inflammasome activation in head and neck cancer. Oncotarget 2017;8:48972-82. [Crossref] [PubMed]
- Shirasuna K, Karasawa T, Takahashi M. Role of the NLRP3 Inflammasome in Preeclampsia. Front Endocrinol (Lausanne) 2020;11:80. [Crossref] [PubMed]
- Shi J, Zhao Y, Wang Y, et al. Inflammatory caspases are innate immune receptors for intracellular LPS. Nature 2014;514:187-92. [Crossref] [PubMed]
- Liu X, Zhang Z, Ruan J, et al. Inflammasome-activated gasdermin D causes pyroptosis by forming membrane pores. Nature 2016;535:153-8. [Crossref] [PubMed]
- Wu DM, He M, Zhao YY, et al. Increased susceptibility of irradiated mice to Aspergillus fumigatus infection via NLRP3/GSDMD pathway in pulmonary bronchial epithelia. Cell Commun Signal 2022;20:98. [Crossref] [PubMed]
- Haneklaus M, O'Neill LA, Coll RC. Modulatory mechanisms controlling the NLRP3 inflammasome in inflammation: recent developments. Curr Opin Immunol 2013;25:40-5. [Crossref] [PubMed]
(English Language Editor: K. Brown)