Changes of blood gas analysis in moderate-to-severe acute respiratory distress syndrome patients during long-term prone position ventilation: a retrospective cohort study
Highlight box
Key findings
• Prone position ventilation (PPV) improves the patients’ oxygenation, however, the associated incidence of hyperoxia exceeds 33%. PPV may alleviate the acidosis associated with permissive hypercapnia in acute respiratory distress syndrome (ARDS) patients treated with lung protective ventilation strategy (LPVS).
What is known and what is new?
• PPV is widely used to improve the oxygenation and prognosis of patients with moderate-to-severe ARDS.
• We report that the incidence of hyperoxia after PPV is as high as 33%, which deserves attention. With increasing elastic resistance in the chest and abdomen in the prone position, the acidosis associated with permissive hypercapnia do not aggravate.
What is the implication, and what should change now?
• On the premise of ensuring tissue oxygenation, it is necessary to down-regulate FiO2 more sharply in accordance with patients’ conditions after PPV. Prolonged PPV can improve oxygenation in moderate-to-severe ARDS patients without exacerbating permissive hypercapnia caused by LPVS.
Introduction
Prone position ventilation (PPV), which has been shown to be able to improve the prognosis of acute respiratory distress syndrome (ARDS) patients (1), has good social and economic benefits (2). The ARDS prone position network (APRONET) cross-sectional survey conducted in 2017 showed that the utilization rate of PPV in ARDS patients had already reached 32.9% (3). PPV helps to increase the arterial partial pressure of oxygen (PaO2) in most ARDS patients (by up to 70%) (4-6). Furthermore, oxygenation tends to be improved within the first hour in most cases (7). Even for pulmonary ARDS, the improvement in oxygenation reaches a steady state in about 3 hours. Lee et al.’s research findings (8) suggest that oxygenation was improved by over 53% in patients during the first application of PPV, and that oxygenation would continue to be improved for several hours after the implementation of supine position ventilation (SPV). When PPV was repeated, oxygenation would be further improved. Regarding the concept of lung protective ventilation (LPV), the PROSEVA study (1) suggests conducting PPV when PaO2/fraction of inspired oxygen (FiO2) ratio (PFR) is lower than 150 mmHg. For patients with a good response, a concern is whether the incidence of hyperoxia may increase, making it worthwhile to retrospectively analyze the rule of improvement in oxygenation during long-term PPV. The prone position is an unconventional body position. With increasing elastic resistance in the chest and abdomen in the prone position, whether it is necessary to urgently adjust the tidal volume and respiratory rate or not is unclear. Owing to the impact of hypercapnia on all visceral organs, PPV may match ventilation/blood perfusion and improve the right heart function later than the improvement of oxygenation—thus, the effect of early PPV on carbon dioxide partial pressure (PaCO2) is poorly understood. Given low tidal volume LPVS, it is worthwhile to evaluate the effect of prolonged PPV on permissive hypercapnia (9,10); at present, there is no study on the dynamic changes of hyperoxia and permissive hypercapnia during prolonged PPV. In this study, all blood gas indexes were collected before and after each application of PPV in patients to investigate their dynamic change rule in order to offer better guidance on clinical adjustment. We present the following article in accordance with the STROBE reporting checklist (available at https://atm.amegroups.com/article/view/10.21037/atm-22-5907/rc).
Methods
This was a retrospective observational cohort study of adult patients (≥18 years) with ARDS. The inclusion criteria were as follows: (I) adult patients (age ≥18 years) who accepted treatment in the intensive care unit (ICU) of the First Affiliated Hospital of Guangzhou Medical University from November 2015 to May 2021; (II) patients who were diagnosed with ARDS according to the Berlin definition; (III) during hospitalization, underwent PPV at least once. The exclusion criteria were as follows: (I) inability to tolerate the PPV treatment and the duration of PPV was less than 4 hours; (II) patients did not undergo blood analysis during PPV or the blood gas analysis results could not be traced. During the study period, 10,126 patients were admitted to our ICU ward, 620 patients with moderate to severe ARDS were diagnosed, and 103 of these patients received PPV. A total of 6 patients who received PPV for less than 4 hours or whose blood gas analysis results were unable to be obtained were excluded from the study. A total of 97 patients receiving PPV were included in this study (Figure 1). The study was conducted in accordance with the Declaration of Helsinki (as revised in 2013). This study was approved by the Medical Ethics Committee of the First Affiliated Hospital of Guangzhou Medical University (Document No. 34 [2017]). Individual consent for this retrospective analysis was waived. The chief clinician decided whether to continue or stop PPV. PPV was terminated immediately if any patient experienced unstable hemodynamics, arrhythmia, tracheal displacement, or other types of life-threatening deterioration during the treatment. In our study, most of patients involved received PPV for 12–16 hours in total each day, and PPV was performed at an interval of 8 hours.
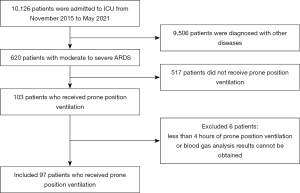
A total of 885 blood gas analyses were collected from the 97 patients included, which were grouped into SPV, PPV early stage (within 4 hours), and PPV middle and late stage (4–16 hours) according to patients’ body positions and duration of PPV when blood gas analysis was conducted (Figure S1).
Data collection
The following general information of patients was collected: gender, age, date of admission, date of transfer to ICU, acute physiological and chronic health evaluation II (APACHE II), sequential organ failure assessment (SOFA), Richmond Agitation-Sedation Scale (RASS), whether neuromuscular blockade was applied or not, principal diagnosis, outcome, and so on. The results of blood gas analysis including PH, PaCO2, PaO2, FiO2, and PFR were collected before (SPV) and after (PPV) each body position change, including PPV early stage and PPV middle and late stage.
Statistical methods
Linear mixed-effects models, taken from LmerTest (v. 3.1-3) and lme4 (v. 1.1.27-1) in R language (v. 4.1.2; Te R Foundation of Statistical Computing, Vienna, Austria), were used to test the effect of body position changes on the blood gas indexes. Then, Satterthwaite’s method was used to generate P values for the mixed models. This method can be used to explore multiple factors and covariates simultaneously with the variance among cases taken into consideration. The models were initially fitted with a random intercept and random slope taken for every case. Model comparisons were made using the maximum likelihood (ML) method to compare the models under different stochastic effects, which were finally trimmed into those containing significantly improved model fitting performance only. After random-effects models were determined, the same method was applied to the selection of fixed effects. The function was used to identify or rule out a singular fit. We finally selected “body position changes, times of PPV, gender, age, baseline SOFA, and baseline APACHE II” as fixed effects for optimal mixed-effects models. There was a random intercept and random slope for individuals when they were affected by the number of prone positions. Blood gas analysis indicators such as “PH, PaCO2, PaO2, FiO2, PFR” are dependent variables. The enumeration data were checked using the chi-squared test. Further, unless otherwise stated, a 2-tailed P value of <0.05 was considered statistically significant.
Results
Baseline data
Among the 97 patients, 68 were male while 29 were female; the average age was (56.52±14.13); 11 cases were diagnosed with septic shock, 26 were diagnosed with connective tissue disease (CTD), 33 were diagnosed with pulmonary infection, 12 were postoperative patients, 9 were diagnosed with the hematologic disease, 4 with chronic obstructed pulmonary disease (COPD), and 2 with barotrauma. The overall survival rate of patients was 53% (Table 1).
Table 1
Baseline data | Value |
---|---|
Age (years), mean ± SD | 56.52±14.13 |
Male, n (%) | 68 (70.10) |
Neuromuscular blockade, n (%) | 48 (49.48) |
APACHE II, mean ± SD | 20.14±6.88 |
RASS, median (1st–3rd quartile) | −4 (−4, −4) |
Pre-prone blood gases, median (1st–3rd quartile) | |
PH | 7.37 (7.30, 7.41) |
PaCO2 (mmHg) | 50.10 (43.85, 60) |
PaO2 (mmHg) | 81.90 (68.55, 103.50) |
FiO2 | 0.80 (0.63, 1.00) |
PaO2/FiO2 (mmHg) | 102.35 (81.05, 146.25) |
Principal diagnosis, n [%] | |
Septic shock | 11 [11] |
CTD | 26 [27] |
Pulmonary infection | 33 [34] |
Postoperative patient | 12 [12] |
Blood disease | 9 [9] |
COPD | 4 [4] |
Barotrauma | 2 [2] |
ICU outcome, survival | 51 [53] |
SD, standard deviation; APACHE, acute physiological and chronic health evaluation; RASS, Richmond Agitation-Sedation Scale; CTD, connective tissue disease; ICU, intensive care unit.
Incidence rate of hyperoxia
Compared with SPV, PPV early stage and PPV middle and late stage saw higher incidence of hyperoxia (P<0.05) (Table 2, Figure 2).
Table 2
Stage | |||
---|---|---|---|
SPV [1] | PPV early stage [2] | PPV middle and late stage [3] | |
Hyperoxia | 39 (12.42%) | 93 (33.33%) | 98 (33.56%) |
Non-hyperoxia | 275 (87.58%) | 186 (66.67%) | 194 (66.44%) |
Total | 314 | 279 | 292 |
P value: 1 vs. 2, P<0.001; 1 vs. 3, P<0.001; 2 vs. 3, P=0.954. PPV, prone position ventilation; SPV, supine position ventilation.
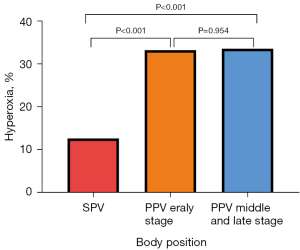
Blood gas analysis changes before and after PPV
PH was significantly higher at PPV middle and late stage than in SPV (P<0.05); there was no significant difference in PaCO2 among SPV, PPV early stage, and PPV middle and late stage (P>0.05); compared with SPV, PaO2 was significantly higher at PPV early stage and PPV middle and late stage, and the difference was statistically significant (P<0.05); compared SPV, FiO2 was significantly lower at PPV early stage and PPV middle and late stage, and it further decreased at PPV middle and late stage compared to PPV early stage (P<0.05); PaO2/FiO2 was significantly higher at PPV early stage and PPV middle and late stage than SPV, and the difference was statistically significant (P<0.05) (Table 3, Figure 3).
Table 3
Blood gases | Stage | P value | ||
---|---|---|---|---|
SPV | PPV early stage | PPV middle and late stage | ||
PH | 7.38 [7.32, 7.41] | 7.37 [7.31, 7.41] | 7.39 [7.34, 7.42]b | <0.05 |
PaCO2 | 51.00 [45.35, 59.13] | 51.50 [45.30, 61.00] | 50.20 [44.30, 58.80] | >0.05 |
PaO2 | 84.65 [70.85, 104.85] | 103.00 [86.10, 128.00]a | 108.10 [88.53, 128.00]a | <0.05 |
FiO2 | 0.70 [0.60, 0.85] | 0.70 [0.57, 0.80]a | 0.66 [0.60, 0.80]a,b | <0.05 |
PFR | 123.00 [91.75, 163.00] | 158.00 [118.00, 203.00]a | 161.00 [129.00, 202.75]a | <0.05 |
Data are expressed as median [interquartile range]. a, P<0.05 vs. SPV; b, P<0.05 vs. early PPV. PPV, prone position ventilation; SPV, supine position ventilation; PaCO2, carbon dioxide partial pressure; PaO2, partial pressure of oxygen; FiO2, fraction of inspired oxygen; PFR, PaO2/FiO2.
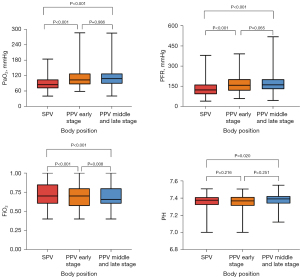
Multivariate analysis and blood gas indexes
PH and PaO2/FiO2 were positively correlated with times of PPV and negatively correlated with FiO2, namely, with the passage of time, PH and PaO2/FiO2 showed an increasing trend whereas FiO2 showed a decreasing trend (P<0.05); FiO2 was positively correlated with the baseline SOFA score and negatively correlated with PH, namely, the higher the baseline SOFA score, the higher FiO2, and the lower PH (P<0.05); blood gas indexes were not significantly correlated with gender, age, and baseline APACHE II (Table 4).
Table 4
Blood gases | Times | Baseline SOFA | Sex, male | Age | Baseline APACHE II |
---|---|---|---|---|---|
PH | 0.011c | 0.003d | >0.05 | >0.05 | >0.05 |
PaCO2 | >0.05 | >0.05 | >0.05 | >0.05 | >0.05 |
PaO2 | >0.05 | >0.05 | >0.05 | >0.05 | >0.05 |
FiO2 | <0.001d | 0.003c | >0.05 | >0.05 | >0.05 |
PFR | 0.007c | >0.05 | >0.05 | >0.05 | >0.05 |
c, estimate >0; d, estimate <0. Time represents the frequency of PPV. SOFA, sequential organ failure assessment; APACHE, acute physiological and chronic health evaluation; PaCO2, carbon dioxide partial pressure; PaO2, partial pressure of oxygen; FiO2, fraction of inspired oxygen; PFR, PaO2/FiO2; PPV, prone position ventilation.
Discussion
Compared with SPV, there was an average rise of 35 and 38 mmHg in patients’ PFR at PPV early and middle-and-late stage, suggesting the continuous improvement of oxygenation in long-term PPV, which was consistent with the results of a previous study (1). The incidence of hyperoxia in the ICU could reach 50% according to previous studies, which was closely related to the length of stay and mortality rate (11,12), but clinicians pay far less attention to hyperoxia than to hypoxia, especially when FiO2 has dropped below 60% (13). In this study, PaO2 rose after the patients underwent PPV, with the median PaO2 fluctuating from 84.65 to 108.10 mmHg. Some 33.3% of the patients were diagnosed with hyperoxia (PaO2 ≥120 mmHg) (14) at the PPV early stage, whereas 33.6% were diagnosed with hyperoxia at PPV middle and late stage, both significantly higher than those at SPV; hyperoxia makes it possible to generate reactive oxygen species (ROS) and free radicals, which have pro-inflammatory and cytotoxic effects, causing oxidative stress and neuron injuries, which may aggravate the damage to multiple organs including the heart, lung, kidney, and brain (15-17). A recent observational study indicated a U-shaped relationship between PaO2 and mortality in ARDS patients, with a minimum mortality risk when PaO2 was at 93.8–105 mmHg (18); moreover, at PPV early stage and PPV middle and late stage in this study, the chief physician took the initiative to down-regulate FiO2 with the improvement of oxygenation, but the median remained above 0.7. Hyperoxia can directly damage bronchial alveolar epithelium, aggravating mechanical ventilation-related lung injuries (19,20), increasing the risk of oxygen poisoning and ventilator-associated pneumonia (VAP) (21). Especially at PPV middle and late stage, median FiO2 further decreased to 0.66, but there was still a slow increase in the proportion of patients with hyperoxia.
There is a long controversy over the research on liberal oxygen therapy and conservative oxygen therapy. ARDS patients mostly experience hypoxemia-related complications at the early stage. Although a clear recommendation on PaO2 from ARDSnet (22), clinicians, who are still concerned about histanoxia, risk taking hyperoxia as a means of raising oxygen reserves (23), but actually, there are many factors affecting oxygen delivery. Despite the lack of evidence for a significant dose-effect relationship between PaO2 and mortality, the number of days with PaO2 >120 mmHg is an independent risk factor for VAP (17). The HYPER2S randomized controlled trial (RCT) suggests that patients with hyperoxia experience more severe ICU-acquired weakness, and the mortality increases on day 28 after complication with lactic acid elevation (24,25). Most of the cases included in this study were pulmonary ARDS patients with septic shock. The data suggested that FiO2 was positively correlated with the baseline SOFA score and negatively correlated with PH. In other words, the higher the baseline SOFA score, the higher FiO2 and the lower PH. This might be related to the difference in SpO2 and SaO2 in response to peripheral tissue perfusion during septic shock (26). Clinicians often use higher FiO2 to avoid histanoxia (13), whereas the target of oxygen therapy is relatively free. After primary diseases gradually came under control, the proportion of hyperoxia caused by PPV reached 33.6%.
Low tidal volume LPVS is normally adopted for moderate-to-severe ARDS patients, with a sedative and analgesic or even neuromuscular blocking agents used in combination to reduce abnormal central respiratory drive (27). The results of this study suggest that the median PaCO2 in the patients was 50.1 mmHg before PPV, reaching the standard of permissive hypercapnia. During PPV, the anterior chest wall and abdomen were restricted, with elastic resistance increasing and minute volume decreasing. PaCO2 inevitably rose further when lung homogeneity had not yet been optimally achieved after a change in the body position (9). Severe hypercapnia or a rapid rise in PaCO2 could cause myocardial depression, leading to an increase in pulmonary vascular resistance (PVR), aggravating right heart insufficiency, thereby increasing the risk of inpatient death in patients with cerebral injury (28), and exacerbating concomitant symptoms such as renal injury, pulmonary injury, and so on (29,30). Is there a need to adjust the tidal volume and respiratory rate as early as possible? This study indicated that PaCO2 rose slightly at PPV early stage, but there was no significant difference from SPV, and PaCO2 even decreased gradually at PPV middle and late stage; at the same time, PH showed a downward trend at PPV early stage compared with SPV, but there was no significant difference. PH tended to rise significantly at the middle-and-late stage compared with PPV early stage. A previous study revealed that the PaCO2 responders had a better prognosis after PPV (31). For patients with pulmonary ARDS included in this study, a long-term prone position helped to promote systemic venous return and reduce PVR while improving oxygenation and enhancing pulmonary reexpansion, leading to an increase in cardiac output (32), thereby improving ventilation/blood flow ratio, and finally relieving acidosis.
Conclusions
This study holds that on the premise of ensuring tissue oxygenation, it is necessary to down-regulate FiO2 more sharply in accordance with patients’ conditions after PPV, and select a recommended target of oxygen therapy (33) to decrease FiO2 to or below 60%, so as to reduce the incidence of hyperoxia and its impact on the lung and other organs for better efficacy of PPV; prolonged PPV can improve oxygenation in moderate-to-severe ARDS patients without exacerbating permissive hypercapnia caused by LPVS.
Limitations
There are still some shortcomings in this study, as follows: In the single-center retrospective study, blood gas indexes were primarily analyzed without any reference to oxygen toxicity-related biological indicators and cytokines; efficacy comparisons were not performed for patients with ARDS caused by different etiological factors; the outcome of patients was not taken into account, and so on.
Acknowledgments
Funding: This study was supported by the Guangzhou Science and Technology Planning Project (No. 202102010353), Zhongnanshan Medical Foundation of Guangdong Province (No. ZNSA-2020003), Guangzhou Institute of Respiratory Health/2019 Clinical Independent Exploration Project of National Clinical Research Center of the First Hospital of Guangzhou Medical University (No. 2019GIRHZ10), and the Project of Cultivating High-level Clinical Research launched by the Guangzhou Medical University.
Footnote
Reporting Checklist: The authors have completed the STROBE reporting checklist. Available at https://atm.amegroups.com/article/view/10.21037/atm-22-5907/rc
Data Sharing Statement: Available at https://atm.amegroups.com/article/view/10.21037/atm-22-5907/dss
Conflicts of Interest: All authors have completed the ICMJE uniform disclosure form (available at https://atm.amegroups.com/article/view/10.21037/atm-22-5907/coif). The authors have no conflicts of interest to declare.
Ethical Statement: The authors are accountable for all aspects of the work in ensuring that questions related to the accuracy or integrity of any part of the work are appropriately investigated and resolved. The study was conducted in accordance with the Declaration of Helsinki (as revised in 2013). This study was approved by the Medical Ethics Committee of the First Affiliated Hospital of Guangzhou Medical University (Document No. 34 [2017]). Individual consent for this retrospective analysis was waived.
Open Access Statement: This is an Open Access article distributed in accordance with the Creative Commons Attribution-NonCommercial-NoDerivs 4.0 International License (CC BY-NC-ND 4.0), which permits the non-commercial replication and distribution of the article with the strict proviso that no changes or edits are made and the original work is properly cited (including links to both the formal publication through the relevant DOI and the license). See: https://creativecommons.org/licenses/by-nc-nd/4.0/.
References
- Guérin C, Reignier J, Richard JC, et al. Prone positioning in severe acute respiratory distress syndrome. N Engl J Med 2013;368:2159-68. [Crossref] [PubMed]
- Baston CM, Coe NB, Guerin C, et al. The Cost-Effectiveness of Interventions to Increase Utilization of Prone Positioning for Severe Acute Respiratory Distress Syndrome. Crit Care Med 2019;47:e198-205. [Crossref] [PubMed]
- Guérin C, Beuret P, Constantin JM, et al. A prospective international observational prevalence study on prone positioning of ARDS patients: the APRONET (ARDS Prone Position Network) study. Intensive Care Med 2018;44:22-37. [Crossref] [PubMed]
- Fernandez R, Trenchs X, Klamburg J, et al. Prone positioning in acute respiratory distress syndrome: a multicenter randomized clinical trial. Intensive Care Med 2008;34:1487-91. [Crossref] [PubMed]
- Dupont H, Depuydt P, Abroug F. Prone position acute respiratory distress syndrome patients: less prone to ventilator associated pneumonia? Intensive Care Med 2016;42:937-9. [Crossref] [PubMed]
- Scholten EL, Beitler JR, Prisk GK, et al. Treatment of ARDS With Prone Positioning. Chest 2017;151:215-24. [Crossref] [PubMed]
- Roche-Campo F, Aguirre-Bermeo H, Mancebo J. Prone positioning in acute respiratory distress syndrome (ARDS): when and how? Presse Med 2011;40:e585-94. [Crossref] [PubMed]
- Lee HY, Cho J, Kwak N, et al. Improved Oxygenation After Prone Positioning May Be a Predictor of Survival in Patients With Acute Respiratory Distress Syndrome. Crit Care Med 2020;48:1729-36. [Crossref] [PubMed]
- Hering R, Kreyer S, Putensen C. Effects of lung protective mechanical ventilation associated with permissive respiratory acidosis on regional extra-pulmonary blood flow in experimental ARDS. BMC Anesthesiol 2017;17:149. [Crossref] [PubMed]
- Mekontso Dessap A, Gendreau S, Vieillard-Baron A. Respective roles of hypercapnia and acidosis in acute distress respiratory syndrome. Intensive Care Med 2022;48:787-8. [Crossref] [PubMed]
- Girardis M, Busani S, Damiani E, et al. Effect of Conservative vs Conventional Oxygen Therapy on Mortality Among Patients in an Intensive Care Unit: The Oxygen-ICU Randomized Clinical Trial. JAMA 2016;316:1583-9. [Crossref] [PubMed]
- Chu DK, Kim LH, Young PJ, et al. Mortality and morbidity in acutely ill adults treated with liberal versus conservative oxygen therapy (IOTA): a systematic review and meta-analysis. Lancet 2018;391:1693-705. [Crossref] [PubMed]
- de Graaff AE, Dongelmans DA, Binnekade JM, et al. Clinicians' response to hyperoxia in ventilated patients in a Dutch ICU depends on the level of FiO2. Intensive Care Med 2011;37:46-51. [Crossref] [PubMed]
- Page D, Ablordeppey E, Wessman BT, et al. Emergency department hyperoxia is associated with increased mortality in mechanically ventilated patients: a cohort study. Crit Care 2018;22:9. [Crossref] [PubMed]
- Singer M, Young PJ, Laffey JG, et al. Dangers of hyperoxia. Crit Care 2021;25:440. [Crossref] [PubMed]
- Angus DC. Oxygen Therapy for the Critically Ill. N Engl J Med 2020;382:1054-6. [Crossref] [PubMed]
- Six S, Jaffal K, Ledoux G, et al. Hyperoxemia as a risk factor for ventilator-associated pneumonia. Crit Care 2016;20:195. [Crossref] [PubMed]
- Boyle AJ, Holmes DN, Hackett J, et al. Hyperoxaemia and hypoxaemia are associated with harm in patients with ARDS. BMC Pulm Med 2021;21:285. [Crossref] [PubMed]
- Yamada M, Kubo H, Kobayashi S, et al. Interferon-gamma: a key contributor to hyperoxia-induced lung injury in mice. Am J Physiol Lung Cell Mol Physiol 2004;287:L1042-7. [Crossref] [PubMed]
- Li LF, Liao SK, Ko YS, et al. Hyperoxia increases ventilator-induced lung injury via mitogen-activated protein kinases: a prospective, controlled animal experiment. Crit Care 2007;11:R25. [Crossref] [PubMed]
- Ohshimo S. Oxygen administration for patients with ARDS. J Intensive Care 2021;9:17. [Crossref] [PubMed]
- Acute Respiratory Distress Syndrome Network. Ventilation with lower tidal volumes as compared with traditional tidal volumes for acute lung injury and the acute respiratory distress syndrome. N Engl J Med 2000;342:1301-8. [Crossref] [PubMed]
- Itagaki T, Nakano Y, Okuda N, et al. Hyperoxemia in mechanically ventilated, critically ill subjects: incidence and related factors. Respir Care 2015;60:335-40. [Crossref] [PubMed]
- Asfar P, Schortgen F, Boisramé-Helms J, et al. Hyperoxia and hypertonic saline in patients with septic shock (HYPERS2S): a two-by-two factorial, multicentre, randomised, clinical trial. Lancet Respir Med 2017;5:180-90. [Crossref] [PubMed]
- Demiselle J, Wepler M, Hartmann C, et al. Hyperoxia toxicity in septic shock patients according to the Sepsis-3 criteria: a post hoc analysis of the HYPER2S trial. Ann Intensive Care 2018;8:90. [Crossref] [PubMed]
- Thijssen M, Janssen L, le Noble J, et al. Facing SpO(2) and SaO(2) discrepancies in ICU patients: is the perfusion index helpful? J Clin Monit Comput 2020;34:693-8. [Crossref] [PubMed]
- Jonkman AH, de Vries HJ, Heunks LMA. Physiology of the Respiratory Drive in ICU Patients: Implications for Diagnosis and Treatment. Crit Care 2020;24:104. [Crossref] [PubMed]
- Tiruvoipati R, Pilcher D, Botha J, et al. Association of Hypercapnia and Hypercapnic Acidosis With Clinical Outcomes in Mechanically Ventilated Patients With Cerebral Injury. JAMA Neurol 2018;75:818-26. [Crossref] [PubMed]
- Audard V, Homs S, Habibi A, et al. Acute kidney injury in sickle patients with painful crisis or acute chest syndrome and its relation to pulmonary hypertension. Nephrol Dial Transplant 2010;25:2524-9. [Crossref] [PubMed]
- Nin N, Muriel A, Peñuelas O, et al. Severe hypercapnia and outcome of mechanically ventilated patients with moderate or severe acute respiratory distress syndrome. Intensive Care Med 2017;43:200-8. [Crossref] [PubMed]
- Gattinoni L, Vagginelli F, Carlesso E, et al. Decrease in PaCO2 with prone position is predictive of improved outcome in acute respiratory distress syndrome. Crit Care Med 2003;31:2727-33. [Crossref] [PubMed]
- Jozwiak M, Monnet X, Teboul JL. Optimizing the circulation in the prone patient. Curr Opin Crit Care 2016;22:239-45. [Crossref] [PubMed]
- Young PJ, Hodgson CL, Rasmussen BS. Oxygen targets. Intensive Care Med 2022;48:732-5. [Crossref] [PubMed]
(English Language Editor: J. Jones)