Hydrogen sulfide alleviates ischemia induced liver injury by repressing the SPHK1/S1P pathway
Highlight box
Key findings
• Hydrogen sulfide (H2S) alleviates hepatic ischemia/reperfusion injury by inhibition of endoplasmic reticulum stress.
What is known and what is new?
• H2S mediates the SPHK1/S1P pathway and hepatic cell viability by reducing the expression of CHOP under hypoxic conditions;
• H2S alleviates hepatic injury and inflammatory response in the I/R induced liver injury model.
What is the implication, and what should change now?
• H2S may be a promising therapeutic strategy for I/R induced liver injury.
Introduction
Ischemia/reperfusion (I/R) injury is a common complication following liver transplant, and can lead to acute and chronic organ rejection (1). Therefore, alleviating I/R induced liver injury during clinical operations will improve the success rate of surgery and support the effective treatment of liver diseases. However, the mechanisms by which I/R injury occurs are complex and remain to be fully elucidated. Furthermore, currently, there is a paucity of effective drugs for the treatment of I/R induced liver damage (2).
The endoplasmic reticulum (ER) is a primary cellular organelle involved in protein synthesis that is highly sensitive to stress. The responses during I/R, such as hypoxia, disrupt homeostasis of the ER, which leads to the accumulation of dysfunctional proteins, and subsequently, causes ER stress. To recover ER homeostasis, the unfolded protein response (UPR), the accumulation of misfolded proteins in the ER triggers the induction of chaperones that attempt to refold the misfolded proteins (3), is stimulated by ER stress. Although the UPR can prevent cells from excessive injury, continuous ER stress can induce severe inflammation and cell apoptosis. Thus, hepatic ER stress involves various pathology, in particular, hepatic I/R (4). And in sepsis induced ER stress mediators of inflammation and cell death, Yousof et al. findings further demonstrate an essential role for ER stress-induced CHOP in the pathogenesis of septic injury in animal models (5). The transcription factor CCAAT-enhancer-binding protein homologous protein (CHOP) plays a crucial role in the regulation of ER stress and can induce cell apoptosis. For example, hypoxia increases the expression of CHOP, thereby increasing ER stress, followed by apoptosis (6).
Sphingosine-1-phosphate (S1P) is a bio-active lipid that has been implicated in physiological and pathological processes, such as angiogenesis, cell migration, immune cell trafficking, and inflammation. S1P is catalyzed by sphingosine kinase (SPHK) and has been shown to play a key role in chronic liver injury, suggesting that SPHK1/S1P signaling may be a promising therapeutic target in liver diseases (7). Qi et al. has found that SPHK1/S1P signaling can inhibit the expression of CHOP. Overexpression of IRE1α significantly attenuated the protective effect of SPHK1 on palmitate-induced CHOP expression, poly-(ADP-ribose) polymerase (PARP) cleavage, and cell death (8). However, the relationship between hydrogen sulfide (H2S) and SPHK1/S1P signaling during liver injury remains to be fully elucidated.
Up until recently, H2S was considered a toxic agent. It is now regarded as an important signaling molecule which participates various essential physiological and pathological processes. For example, in the central nervous system, administration of H2S ameliorates ischemic injuries (9). In the cardiovascular system, H2S can relax the mammalian mesenteric artery and the thoracic aorta, suggesting that H2S possesses plays an effective role in the regulation of contractility and blood pressure (10). In the respiratory system, the therapeutic relationship between H2S and pulmonary diseases such as hypertension has gained increasing attention (11). However, remains unclear whether H2S can exert a protective effect against acute liver injury induced by I/R. This current study documented the benefits of H2S in the treatment of liver injury and identified a crosstalk between H2S and hepatic SPHK1/S1P signaling via ER stress in I/R induced liver injury. We present the following article in accordance with the ARRIVE reporting checklist (available at https://atm.amegroups.com/article/view/10.21037/atm-22-6460/rc).
Methods
Experimental animals
A total of 40 adult Wistar male rats (5–6 weeks), weighing 150–200 g, were purchased from the Laboratory Animal Center of Southern Medical University and randomly divided into 5 groups (n=8). All the animals were housed at a contain temperature of 24–28 ℃ and fed with standard chow with ad libitum water. All animal experiments were approved by the Institutional Animal Ethics Committee at the People’s Hospital of Huadu District, in compliance with institutional guidelines for the care and use of animals. A protocol was prepared before the study without registration.
Construction of the I/R induced liver injury model in rats
The I/R induced liver injury was performed in rats as follows. Briefly, 40 mg/kg pentobarbital sodium was used to anesthetize the rats by intraperitoneal (i.p.) injection and laparotomy was subsequently performed. During induction of liver ischemia, a vascular clamp was used to block the portal vein and the bile duct. This was followed by liver reperfusion at 30 minutes after ischemia. Sham rats underwent the same operation without the conduction of I/R. To examine the therapeutic effects of H2S on liver injury, sodium hydrosulfide (NaHS; 14 mmol/kg) (12) or PR-619 (an ER stress agonist, MCE, HY-13814), a broad-spectrum deubiquitinating enzyme (DUB) inhibitor (5 mmol/kg), was diluted in 200 µL sterile phosphate-buffered saline (PBS) and administered to the I/R induced rats via i.p. injection. The control group mice were given with the same volume PBS by i.p. injection.
Hematoxylin and eosin (H&E) staining
Rat liver were fixed with 4% formaldehyde solution overnight and embedded in paraffin. Serial liver sections (5-µm thick) were stained with H&E to assess the degree of liver injury. The Nikon Eclipse TS100 microscope was used to obtain the images.
Immunohistochemical staining
The liver tissues were prepared as serial sections as described above and treated with citric acid antigen repair buffer (pH =6.0). The liver sections were the incubated with anti-CHOP antibodies (Affinity, AF5280), anti-SPHK1 antibodies (Affinity, AF6005), and anti-S1P antibodies (Affinity, DF4159) overnight at 4 ℃. After rewarming for 1 hour at 37 ℃, the tissue sections were incubated with the secondary antibodies conjugated with horseradish peroxidase (HRP; ab150083, 1:1,000; Abcam, USA) at 37 ℃ for 1 hour. The Nikon Eclipse TS100 microscope was used to obtain images.
Cell culture and hypoxia treatment
The rat hepatic cell line BRL-3A was cultured in Dulbecco’s Modified Eagle Medium (DMEM; GIBCO, 12430047), supplemented with 10% fetal bovine serum (FBS) and 1% antibiotics (GIBCO, 15640055). For normoxic conditions, cells were cultured in a 5% CO2 incubator, while cells cultured under hypoxia were grown in a hypoxic chamber containing 1% O2, 5% CO2, and 94% N2 at 37 ℃. To examine the therapeutic effect of H2S on hepatocytes injury, hypoxic cells were treated with NaHS (30 µmol) and the control group was treated with the same volume of PBS.
Cell viability assay
The MTT assay (ab211091; Abcam) was used to assess cell viability. The 1×104 cells were seeded in 96 well plates and cultured under different conditions. After 72 hours, 50 µL of MTT reagent (1 mg/mL) was added to each well and incubated for 1 hour. The density was then quantified with a multimode reader (SYNERGR LX; BioTEK, USA).
Confocal microscopy
For immunofluorescence analysis, BRL-3A cells were fixed in 4% paraformaldehyde and permeabilized with 0.1% Triton X-100 in PBS. After blocking with 5% bovine serum albumin (BSA) solution, the BRL-3A cells were incubated with anti-CHOP antibodies, anti-SPHK1 antibodies, and anti-S1P antibodies overnight at 4 ℃. The cells were then washed and stained with fluorescence-conjugated secondary antibodies [fluorescein isothiocyanate (FITC), Invitrogen] and Hoechst dye (62249, Invitrogen).
Western blot analyses
Western blot analyses were performed to examine the protein expression in BRL-3A cells or liver tissues. Total protein from BRL-3A cells or liver tissues were extracted using a lysis buffer (P0013B, Beyotime). The protein concentrations were determined using the BCA Protein Assay Kit (P0010, Beyotime). A total of 40 µg proteins per sample was separated by 10% sodium dodecyl-sulfate polyacrylamide gel electrophoresis (SDS-PAGE) and transferred to polyvinylidene fluoride (PVDF) membranes (ISEQ00010, Millipore). The membranes were incubated in 5% non-fat milk solved in Tris-buffered saline Tween (TBST) for 1 hour at room temperature, followed by incubation with anti-CHOP antibodies (Affinity, AF5280), anti-SPHK1 antibodies (Affinity, AF6005), anti-S1P antibodies (Affinity, DF4159), and anti-glyceraldehyde 3-phosphate dehydrogenase (GAPDH) antibodies (ab9485; Abcam) overnight at 4 ℃. Membranes were then incubated with diluted HRP conjugated secondary antibody (1:5,000, ab6721; Abcam) for 1 hour at 37 ℃. An enhanced chemiluminescence (ECL) enhanced chemiluminescence substrate was used to visualize the protein bands using an odyssey system (Li-COR, USA). Signal intensity was quantified using ImageJ software (National Institutes of Health) with background subtraction.
Enzyme-linked immunosorbent assay (ELISA)
The levels of interleukin (IL)-6 (E-EL-R0015c; Elabscience, China), IL-1β (E-EL-R0012c; Elabscience), and IL-18 (E-EL-R0567c; Elabscience) in the serum were determined using ELISA kits and the 96-well plates placed into a multimode reader (SYNERGR LX; BioTEK).
Statistical analysis
The quantitative data are presented as mean ± standard error of the mean (SEM). All statistical analyses were performed using GraphPad Prism (v.8.0c). All data were analyzed using unpaired Student’s t-tests (for comparison of two experimental conditions) or ANOVA (for comparison of three or more experimental conditions) by using GraphPad Prism.
Results
H2S decreases ER stress and hepatic cell viability in hypoxic condition
To measure the effect of NaHS on the viability of hepatic cells during the hypoxic stress, the rat liver cell line BRL-3A was incubated with or without NaHS and subsequently cultured in hypoxic conditions for 72 hours. The viability of BRL-3A cells was measured using the MTT assay. Cells placed under hypoxic conditions for 48 hours showed decreased cell viability compared to control cells. However, NaHS treatment rescued the viability of BRL-3A cells under hypoxic conditions. Cells were treated with NaHS under hypoxic conditions for 72 hours showed significantly greater improvement in cell viability compared to 48 hours (Figure 1A). Hoechst staining showed that the apoptosis of BRL-3A cells was notable in hypoxic conditions, while Hoechst positively stained cells were rarely observed in the control group. Of note, NaHS preconditioning significantly attenuated the cell apoptosis under hypoxia, as shown by the fewer Hoechst positively stained cells (Figure 1B).
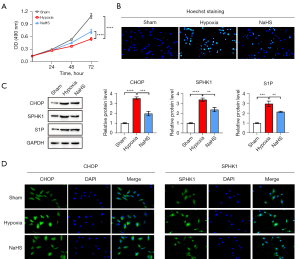
ER stress has been demonstrated to play a key role in liver injury. Thus, the expression of CHOP in the liver was investigated to confirm the correlation between NaHS and ER stress during I/R induced liver injury. The Western blot results showed that the expression of CHOP was significantly elevated during the I/R process. Furthermore, the protein expression of SPHK1 and S1P, which are both associated with hepatic cell apoptosis and liver injury, was also upregulated in the I/R group. Interestingly, all these effects were markedly diminished by NaHS treatment (Figure 1C). These results were further confirmed by immunofluorescence assays, showing that the fluorescence intensity of CHOP was increased under hypoxia and reduced by NaHS treatment. Similarly, accumulation of both SPHK-1 and CHOP reached a peak after hypoxia exposure and this was effectively inhibited by NaHS treatment (Figure 1D, Figure S1A).
NaHS mediates SPHK1/S1P and hepatic cell viability by reducing the expression of CHOP in hypoxic conditions
To confirm the involvement of ER stress and the SPHK-1/SIP axis in the NaHS-mediated prevention of BRL-3A cell injury during hypoxia, CHOP was overexpressed to increase the ER stress in BRL-3A cells. The MTT assay revealed that the viability of BRL-3A cells was increased by NaHS treatment. However, CHOP overexpression visibly reduced the prevention of BRL-3A cell growth by NaHS under hypoxic conditions (Figure 2A). The effects of CHOP on cellular viability of BRL-3A cells exposed to hypoxia were further examined by Hoechst staining analysis. The results showed significant apoptosis under hypoxic conditions, while the number of positively stained cells was decreased by NaHS treatment. Of note, increased CHOP expression significantly aggravated the cell apoptosis, as evidenced by enhanced Hoechst positive staining cells (Figure 2B, Figure S1B).
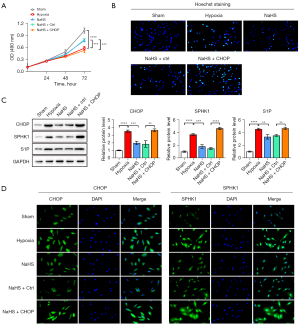
As shown in Figure 2C, CHOP overexpression was successfully achieved by CHOP plasmid transfection. The expression of SPHK1 and S1P were significantly upregulated by hypoxia, and effectively inhibited by NaHS treatment (Figure 2C). However, the inhibitory effect of NaHS on the expression of the above genes during hypoxia was reversed by CHOP overexpression (Figure 2C). Immunofluorescence assay revealed that CHOP was markedly upregulated. Both SPHK1 and S1P fluorescence intensity were notably upregulated after exposure to hypoxia, but was obviously attenuated by NaHS treatment. However, the decrease in SPHK1 and S1P fluorescence intensity was restored by CHOP overexpression (Figure 2D, Figure S1C).
NaHS alleviates hepatic injury and the inflammatory response in the rat I/R liver injury model
The secretion of various cytokines leading to severe inflammatory responses and tissues injury is a predominate event during I/R induced liver injury. To investigate the role of ER stress in the therapeutic effect of H2S during I/R induced liver injury, PR-619, an ER stress agonist. The serum levels of IL-6, IL-1β, and IL-18 were examined by ELISA. The results showed that I/R treated rats had significantly elevated levels of serum IL-6, IL-1β, and IL-18 compared to sham operated rats. NaHS treatment dramatically reversed the I/R induced increase of these inflammatory cytokines. However, PR-619 treatment severely aggravated the increased levels of inflammatory cytokines (Figure 3A-3C).
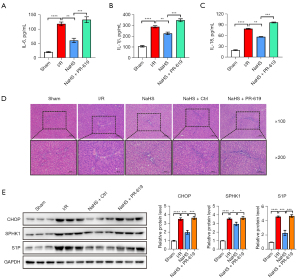
H&E staining was performed to evaluate the therapeutic effect of H2S in the I/R induced rat model. Liver sections from the sham group showed normal hepatocytes arrangement (Figure 3B). In contrast, in the I/R group, inflammatory infiltration and disorganized hepatic cells were observed. The histological hepatic tissue damage induced by I/R treatment was significantly improved after NaHS intervention, as shown by the more normal morphology of liver tissues and the alleviation of hepatic inflammation. PR-619 treatment caused more severe damaged and pathological changes in hepatic morphology, which was reflected as severely disorganized tissue and inflammatory infiltration (Figure 3D). These results suggested that treatment with NaHS ameliorated the I/R induced hepatic injury.
The expression of CHOP, SPHK1, and S1P in the liver were assessed (Figure 3E). The expression of CHOP was conspicuously increased in liver tissues from the I/R treated rats compared with the sham operated rats, and preconditioning with NaHS markedly reduced the expression of CHOP compared with the I/R group. Of note, the expression of CHOP was decreased in the NaHS + control group compared to the IR group, suggesting that NaHS is associated with the ER stress. Overexpression of SPHK1 and S1P was observed in liver tissues from I/R induced rats, which was reduced after treatment with NaHS. However, this was markedly increased with NaHS + PR-619 group (Figure 3E). Subsequently, immunohistochemical analysis of CHOP, SPHK1, and S1P was performed in liver sections. Compared with the sham group, CHOP positive hepatocytes were visible as brown granules, predominantly expressed in the cytoplasm of the hepatocytes, with some expression in the nucleus. The number of these positive staining hepatocytes was significantly decreased by NaHS treatment and restored to a relative higher abundance after PR-619 treatment. Similarly, SPHK1 and S1P were significantly upregulated in the liver of I/R induced rats, and markedly decreased upon treatment with NaHS. However, PR-619 treatment significantly reversed the NaHS mediated inhibition of these proteins (Figure 4). These results confirmed that the hepatic I/R injury rat model was established successfully, and treatment with H2S ameliorated the I/R induced hepatic injury by repressing ER stress.
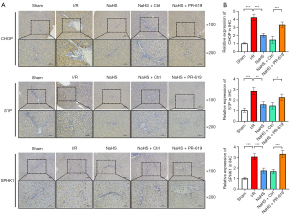
Discussion
I/R induced liver injury is an unavoidable clinical complication of liver surgery due to the recovery of blood perfusion after prolonged ischemic injury (1). This current study demonstrated that H2S treatment efficaciously improved I/R induced liver injury by alleviating inflammation and decreasing hepatic apoptosis. Mechanistically, H2S inhibited the SPHK1/S1P pathway by decreasing ER stress, and this may represent a potential therapeutic measure for preventing I/R induced liver injury during clinical hepatic surgery.
While H2S has been regarded as a toxic agent in the past, recent research have suggested that H2S may in fact participate in many physiological processes, including angiogenesis, neuronal activity, vascular relaxation, and glucose homeostasis. For example, endogenous H2S functions as a neural modulator in the brain (13). Furthermore, H2S has been reported to regulate cardiovascular homeostasis, which is dependent on activation of AKT (14). In addition, the preventive function of H2S on I/R injury in organs and tissues has been demonstrated by a number of studies. For example, H2S has been shown to protect neurons from oxidative stress by scavenging mitochondrial reactive oxygen species (ROS) and facilitating glutathione production (2,15). H2S also protects photoreceptor cells from light-induced deterioration via inhibition of the elevated Ca2+ concentrations in photoreceptor cells (16).
ER stress is an important driver of hepatocyte damage and is thus related to many hepatic diseases. The current study revealed that H2S inhibited ER stress, thereby reducing I/R induced liver injury. Other studies have also shown that H2S can inactivate ER stress, which accelerates Nrf2 translocation into the nucleus, and subsequently increases the expression of anti-apoptotic and antioxidant gene (17-19). In eukaryotic cells, CHOP is closely related to ER stress, and permits the transduction of downstream processes involved in apoptosis (20). As an important downstream regulator of ER stress, CHOP prevents perturbations in the AKT/FOXO3a dependent pathway to restrain ER stress (21). Similarly, ROS in neuronal cells activate the CRP78/CHOP axis and induce mitochondrial dysfunction via the JNK-ERK pathway. CHOP deficiency prevents this ROS-induced neuronal cell death (22). Hypoxia and HIF-1α can trigger ER stress and CHOP-mediated apoptosis in alveolar epithelial cells (23). Similarly, our data indicated that CHOP expression was markedly increased in both hypoxic hepatic cells and I/R induced liver injury in rats, and this was attenuated by H2S treatment. This suggested that CHOP may be a therapeutic target and H2S may be an effective treatment for I/R liver injury. Further experiments were conducted to increased ER stress by CHOP overexpression in vitro and administration of an ER stress agonist in vivo. The results verified that decreased CHOP expression and ER stress are associated with the protective actions of H2S against I/R induced liver injury.
S1P is involved in many physiological or pathological processes, and has been shown to play a critical role in regulating the accumulation of fibrosis in different tissues (7). SPHK1 is a primary catalyzing enzyme of S1P and SPHK1/S1P signaling has been shown to be an important pathway of hepatic fibrosis diseases in different animal models (24-27). Our results in the I/R treated rat model of liver injury suggested that inhibition of the SPHK1/S1P pathway by H2S may be involved in the anti-fibrosis effect in the liver (28,29). The research found deletion or inhibition of SPHK1 mitigates fulminant hepatic failure by suppressing TNFα-dependent inflammation and apoptosis. Thus, a potent inhibitor of SPHK1 could potentially be a therapeutic agent for fulminant hepatitis (30). Thus, we hypothesize that inhibition of SPHK1 activity and the subsequent decreased levels of S1P may be involved in attenuating liver injury by improving liver fibrosis.
There is abundant literature demonstrating that inflammation caused by I/R injury aggravates liver damage. Our results demonstrated that H2S not only reduced the apoptosis caused by ER stress, but also decreased the levels of inflammatory factors, and this may play a crucial role in the protective effect of H2S in I/R induced liver injury. Ischemia causes an initial injury to the liver, and this is followed by more extensive damage during the period of re-perfusion, which is driven by abundant inflammatory factors, including IL-6, IL-1β, and IL-18 (31-33). In our study, H2S was found to efficiently repress the inflammatory levels in the serum of rats following I/R injury, suggesting that H2S may exert an anti-inflammation role in I/R induced liver injury.
There were some limitations to this study. There are three stages of I/R induced liver injury, namely, ischemic preconditioning, conditioning, and postconditioning. Thus, it is necessary to confirm the appropriate time and optimal duration of treatment according to the property of H2S releasing compounds. Only a constant dose of H2S was used in all of the experiments. Since different doses of H2S may exert different therapeutic effects, different doses of H2S should be introduced to further explore the therapeutic effect on the improvement of I/R induced liver injury. In addition, the current research was mainly carried out on an I/R induced injury animal model and further research is warranted to verify the therapeutical effects of H2S in clinical practice.
Conclusions
In summary, this study demonstrated that H2S can improve I/R induce hepatic inflammation and apoptosis, possibly via inhibiting ER stress. Indeed, H2S may be a promising therapeutic strategy for I/R induced liver injury.
Acknowledgments
Funding: This research was supported by the Guangzhou Science and Technology Program Guangzhou Science and Technology Planning Project (No. 202102080659), the People’s Hospital of Huadu District Project (2022-2025, Guangzhou Huadu District People’s Hospital Gastroenterology), and the Guangzhou Huadu District Science and Technology Bureau Project (No. 21-HDWS-001).
Footnote
Reporting Checklist: The authors have completed the ARRIVE reporting checklist. Available at https://atm.amegroups.com/article/view/10.21037/atm-22-6460/rc
Data Sharing Statement: Available at https://atm.amegroups.com/article/view/10.21037/atm-22-6460/dss
Conflicts of Interest: All authors have completed the ICMJE uniform disclosure form (available at https://atm.amegroups.com/article/view/10.21037/atm-22-6460/coif). The authors have no conflicts of interest to declare.
Ethical Statement: The authors are accountable for all aspects of the work, including ensuring that any questions related to the accuracy or integrity of any part of the work have been appropriately investigated and resolved. All animal experiments were approved by the Institutional Animal Ethics Committee at the People’s Hospital of Huadu District, in compliance with institutional guidelines for the care and use of animals.
Open Access Statement: This is an Open Access article distributed in accordance with the Creative Commons Attribution-NonCommercial-NoDerivs 4.0 International License (CC BY-NC-ND 4.0), which permits the non-commercial replication and distribution of the article with the strict proviso that no changes or edits are made and the original work is properly cited (including links to both the formal publication through the relevant DOI and the license). See: https://creativecommons.org/licenses/by-nc-nd/4.0/.
References
- Peralta C, Jiménez-Castro MB, Gracia-Sancho J. Hepatic ischemia and reperfusion injury: effects on the liver sinusoidal milieu. J Hepatol 2013;59:1094-106. [Crossref] [PubMed]
- Whiteman M, Armstrong JS, Chu SH, et al. The novel neuromodulator hydrogen sulfide: an endogenous peroxynitrite 'scavenger'? J Neurochem 2004;90:765-8. [Crossref] [PubMed]
- Glimcher LH, Lee AH, Iwakoshi NN. XBP-1 and the unfolded protein response (UPR). Nat Immunol 2020;21:963-5. [Crossref] [PubMed]
- Yang Y, Wang P, Zhang C, et al. Hepatocyte-derived MANF alleviates hepatic ischaemia-reperfusion injury via regulating endoplasmic reticulum stress-induced apoptosis in mice. Liver Int 2021;41:623-39. [Crossref] [PubMed]
- Yousof T, Sharma H, Austin RC, et al. Stressing the endoplasmic reticulum response as a diagnostic tool for sepsis. Ann Transl Med 2022;10:812. [Crossref] [PubMed]
- Hu H, Tian M, Ding C, et al. The C/EBP Homologous Protein (CHOP) Transcription Factor Functions in Endoplasmic Reticulum Stress-Induced Apoptosis and Microbial Infection. Front Immunol 2018;9:3083. [Crossref] [PubMed]
- Zhao X, Yang L, Chang N, et al. Neutrophil recruitment mediated by sphingosine 1-phosphate (S1P)/S1P receptors during chronic liver injury. Cell Immunol 2021;359:104243. [Crossref] [PubMed]
- Qi Y, Wang W, Chen J, et al. Sphingosine Kinase 1 Protects Hepatocytes from Lipotoxicity via Down-regulation of IRE1α Protein Expression. J Biol Chem 2015;290:23282-90. [Crossref] [PubMed]
- Cunha TM, Dal-Secco D, Verri WA Jr, et al. Dual role of hydrogen sulfide in mechanical inflammatory hypernociception. Eur J Pharmacol 2008;590:127-35. [Crossref] [PubMed]
- Li YF, Xiao CS, Hui RT. Calcium sulfide (CaS), a donor of hydrogen sulfide (H(2)S): a new antihypertensive drug? Med Hypotheses 2009;73:445-7. [Crossref] [PubMed]
- Hongfang J. Effects of hydrogen sulfide on hypoxic pulmonary vascular structural remodeling. Life Sci 2006;78:1299-309. [Crossref] [PubMed]
- Wu DD, Wang DY, Li HM, et al. Hydrogen Sulfide as a Novel Regulatory Factor in Liver Health and Disease. Oxid Med Cell Longev 2019;2019:3831713. [Crossref] [PubMed]
- Abe K, Kimura H. The possible role of hydrogen sulfide as an endogenous neuromodulator. J Neurosci 1996;16:1066-71. [Crossref] [PubMed]
- Cai WJ, Wang MJ, Moore PK, et al. The novel proangiogenic effect of hydrogen sulfide is dependent on Akt phosphorylation. Cardiovasc Res 2007;76:29-40. [Crossref] [PubMed]
- Kimura Y, Kimura H. Hydrogen sulfide protects neurons from oxidative stress. FASEB J 2004;18:1165-7. [Crossref] [PubMed]
- Mikami Y, Shibuya N, Kimura Y, et al. Hydrogen sulfide protects the retina from light-induced degeneration by the modulation of Ca2+ influx. J Biol Chem 2011;286:39379-86. [Crossref] [PubMed]
- Calvert JW, Jha S, Gundewar S, et al. Hydrogen sulfide mediates cardioprotection through Nrf2 signaling. Circ Res 2009;105:365-74. [Crossref] [PubMed]
- Krishnan N, Fu C, Pappin DJ, et al. H2S-Induced sulfhydration of the phosphatase PTP1B and its role in the endoplasmic reticulum stress response. Sci Signal 2011;4:ra86. [Crossref] [PubMed]
- Sen N, Paul BD, Gadalla MM, et al. Hydrogen sulfide-linked sulfhydration of NF-κB mediates its antiapoptotic actions. Mol Cell 2012;45:13-24. [Crossref] [PubMed]
- Travers KJ, Patil CK, Wodicka L, et al. Functional and genomic analyses reveal an essential coordination between the unfolded protein response and ER-associated degradation. Cell 2000;101:249-58. [Crossref] [PubMed]
- Ferri KF, Kroemer G. Organelle-specific initiation of cell death pathways. Nat Cell Biol 2001;3:E255-63. [Crossref] [PubMed]
- Ghosh AP, Klocke BJ, Ballestas ME, et al. CHOP potentially co-operates with FOXO3a in neuronal cells to regulate PUMA and BIM expression in response to ER stress. PLoS One 2012;7:e39586. [Crossref] [PubMed]
- Delbrel E, Soumare A, Naguez A, et al. HIF-1α triggers ER stress and CHOP-mediated apoptosis in alveolar epithelial cells, a key event in pulmonary fibrosis. Sci Rep 2018;8:17939. [Crossref] [PubMed]
- Lan T, Li C, Yang G, et al. Sphingosine kinase 1 promotes liver fibrosis by preventing miR-19b-3p-mediated inhibition of CCR2. Hepatology 2018;68:1070-86. [Crossref] [PubMed]
- Li L, Wang H, Zhang J, et al. SPHK1 deficiency protects mice from acetaminophen-induced ER stress and mitochondrial permeability transition. Cell Death Differ 2020;27:1924-37. [Crossref] [PubMed]
- Ye Q, Zhou Y, Zhao C, et al. Salidroside Inhibits CCl(4)-Induced Liver Fibrosis in Mice by Reducing Activation and Migration of HSC Induced by Liver Sinusoidal Endothelial Cell-Derived Exosomal SphK1. Front Pharmacol 2021;12:677810. [Crossref] [PubMed]
- Hou L, Yang L, Chang N, et al. Macrophage Sphingosine 1-Phosphate Receptor 2 Blockade Attenuates Liver Inflammation and Fibrogenesis Triggered by NLRP3 Inflammasome. Front Immunol 2020;11:1149. [Crossref] [PubMed]
- Nojima H, Freeman CM, Schuster RM, et al. Hepatocyte exosomes mediate liver repair and regeneration via sphingosine-1-phosphate. J Hepatol 2016;64:60-8. [Crossref] [PubMed]
- Kawai H, Osawa Y, Matsuda M, et al. Sphingosine-1-phosphate promotes tumor development and liver fibrosis in mouse model of congestive hepatopathy. Hepatology 2022;76:112-25. [Crossref] [PubMed]
- Avni D, Harikumar KB, Sanyal AJ, et al. Deletion or inhibition of SphK1 mitigates fulminant hepatic failure by suppressing TNFα-dependent inflammation and apoptosis. FASEB J 2021;35:e21415. [Crossref] [PubMed]
- Zhang JX, Li N, Xu QY, et al. Kupffer cell depletion attenuates IL-6/STAT3 mediates hepatocyte apoptosis in immunological liver injury of trichloroethylene sensitized mice. Int Immunopharmacol 2020;88:106897. [Crossref] [PubMed]
- Shen Y, Malik SA, Amir M, et al. Decreased Hepatocyte Autophagy Leads to Synergistic IL-1β and TNF Mouse Liver Injury and Inflammation. Hepatology 2020;72:595-608. [Crossref] [PubMed]
- Akimova T, Zhang T, Christensen LM, et al. Obesity-related IL-18 Impairs T-Regulatory Cell Function and Promotes Lung Ischemia-Reperfusion Injury. Am J Respir Crit Care Med 2021;204:1060-74. [Crossref] [PubMed]