lncRNA GAS5 suppression of the malignant phenotype of ovarian cancer via the miR-23a-WT1 axis
Highlight box
Key findings
• Long noncoding RNA (lncRNA) growth arrest-specific 5 (GAS5) is a tumor-suppressor gene targeting the miR-23a-WT1 axis and hence impedes the malignant behavior of ovarian cancer.
What is known and what is new?
• GAS5 is a member lncRNA that regulates cell viability and has been shown to decrease colorectal and breast cancer carcinogenesis.
• The goal of this study was to identify the essential functions of GAS5 in regulating ovarian cancer advancement and the relevant underlying mechanism.
What is the implication, and what should change now?
• The GAS5/miR-23a/WT1 cascade was found to participate in the progression of ovarian cancer. Generally, lncRNA GAS5 is a tumor-suppressor gene targeting the miR-23a–WT1 axis and hence impedes the malignant behavior of ovarian cancer.
Introduction
Ovarian cancer (OC) refers to a malignant development originating in the ovaries. OC is often believed to be the most prevalent carcinoma among women, with a high fatality rate (1,2). In 2020, a total of 21,750 new OC cases were recorded in the United States. Each year, approximately 13,940 deaths are reported (1). Over the last few decades, cancer research has made significant advancements in the field of cancer diagnosis which has improved early cancer detection and cancer therapy, benefitting patients with better long-term prognosis and increasing the 5-year rate survival of those under treatment up to approximately 90% (3). However, clinical outcomes in advanced-stage OC cases are still poor, with a 5-year rate of survival of about 30% (4). Although some progress has been made, treatment strategies are still limited, and the prognosis of OC is very poor (5). Given this, a thorough investigation of OC’s underlying mechanism at the molecular level is critical.
Long noncoding RNAs (lncRNAs) have recently garnered considerable attention (6,7). lncRNAs are defined as those RNA transcripts with over 200 nucleotides. Moreover, open reading frames are absent in then RNAs, which lack a protein-coding capacity (8). lncRNAs have recently been discovered to be critically involved in the pathophysiological regulation of cancer cell behavior (9). lncRNAs perform a major role in the development and progression of several types of carcinoma by controlling a variety of cellular pathways (both directly and indirectly) (10,11). Several lncRNAs, such as lncRNA-hsa, have been regarded as potential targets for treating OC (12). Different lncRNAs have different mechanisms depending on which cellular pathway they are involved in. lncRNA-hsa promotes growth and metastasis by increasing the amount of secreted protein acidic and rich in cysteine (SPARC)/osteonectin, CWCV and Kazal-like domains proteoglycan (SPOCK2) (13), while lncRNA CLDN14 antisense RNA 1 (PTAR) promotes epithelial-mesenchymal transition (EMT), a hallmark of tumor malignancy that has been linked to increased invasion and metastasis in severe OC. In the case of OC, lncRNA PTAR interacts with the microRNA (miRNA) miR-101-3p competitively for the regulation of ZEB1 expression (14). Also, as a tumor suppressor gene, LINC00641 can inhibit the proliferation and invasion of OC cells by targeting miR-320a (15). Given the above evidence regarding lncRNAs’ role in cancer treatment, they may serve as candidate therapeutic targets against OC and other types of carcinoma.
miRNA is a type of endogenous small noncoding RNA (sncRNA) comprising 18 25 nucleotides that regulates physiological functions including cellular growth, proliferation, and apoptosis (16,17). Several reports have revealed the significant involvement of miRNAs in various malignant processes of OC oncogenesis (18,19). In human malignancies, cross-modulation between miRNAs and lncRNAs has recently been found in multiple studies. In their role as competing endogenous RNAs (ceRNAs), lncRNAs can influence the expressions of messenger RNA (mRNA) by competitively interacting with shared miRNAs (20). From this perspective, the extensive evaluation of miRNAs and lncRNAs could significantly improve the diagnostic and therapeutic approaches in OC.
According to a recent research, the lncRNA growth arrest-specific 5 (GAS5) can enhance apoptosis in triple-negative breast cancer (TNBC) through inhibiting the signaling of miR-378a-5p/SUFU (21). It was also found that elevated expression of lncRNA GAS5 can suppress the metastasis and growth of colorectal cancer cells (22). FTO alpha-ketoglutarate dependent dioxygenase (FTO) promoted EMT process and inflammation response through reducing the m6A modification of lncRNA GAS5 (23). Also, GAS5 functions as a suppressor in glioma stem-like cells (GSCs) by targeting the miR-23a/E-cadherin axis (24). Furthermore, GAS5 was regulated by P-STAT3 and affected the sensitivity of cervical cancer (CC) to cisplatin-based chemotherapy through the miR-21/programmed cell death 4 (PDCD4) axis (25). Its role in OC, on the other hand, is uncertain. In view of this fact, this study was conducted to evaluate its role in OC progression. We found that lncRNA GAS5 binds miRNA-23a to promote the expression of WT1, thus suppressing the progression of OC. The purpose of this study was to analyze a novel candidate target for the therapy of OC. We present the following article in accordance with MDAR reporting checklist (available at https://atm.amegroups.com/article/view/10.21037/atm-22-6394/rc).
Methods
Cell lines and their culture system
The Chinese Academy of Sciences (CAS) (Beijing, China) provided the OC cell line SK-OV-3. These cells were grown in RPMI-1640 (Gibco, Thermo Fisher Scientific, Waltham, MA, USA) enriched with 10% fetal bovine serum (FBS; BioSharp, Hefei, China) and 1% antibiotic solution [penicillin/streptomycin (P/S), BioSharp]. This culture mixture was then placed in an incubator which was maintained with a 5% continuous CO2 supply at 37 ℃. The medium was changed every 1 to 2 days. Additionally, cells were passaged until a confluence of approximately 80% was achieved using trypsin containing 0.05% sodium dodecyl-sulfate (SDS) in a 1:3 ratio.
Cell transfection
Short hairpin RNAs (shRNAs) against lncRNA GAS5 and scrambled control were acquired from GenePharma (Shanghai, China). miR-23a inhibitors, mimics, and negative controls were acquired from RiboBio (Guangzhou, China). Cell transfection was conducted via a designed vector, lncRNA GAS5 shRNA (sh-lncRNA GAS). Lipofectamine 2000 reagent (Invitrogen, Thermo Fisher Scientific) was acquired for the development of a miR-23a mimic, inhibitor, and control (sh-scramble). After 8 h of the experiment, we replaced the medium with a fresh one. Cells that had been transfected for 24 h were harvested to be used in subsequent experiments.
Tissue collection
Human OC tissue samples (n=60) and surrounding noncancerous tissue samples were obtained from those patients who underwent radical resections at The Sixth Affiliated Hospital of Sun Yat-sen University (Guangzhou, China) but had no radiotherapy or chemotherapy. The specimens were preserved in liquid nitrogen prior to their being used. The study was conducted in accordance with the Declaration of Helsinki (as revised in 2013). All patients who participated in this study signed an informed consent form. This study was approved by the Ethics Committee of The Sixth Affiliated Hospital of Sun Yat-sen University (approval No. 2019ZSLYEC-040).
Quantitative real-time polymerase chain reaction (qRT-PCR)
TRI Reagent (Invitrogen) was used to obtain the total RNA content of the cultured cells and was followed by Nanodrop (Implen GmbH, Munich, Germany)-based quantitative examination of the total RNA. Through use of the Prime Script RT reagent kit, 1 mg of RNA was used to construct complement DNA (cDNA) according to the suggested procedure of the manufacturer (Takara Bio, Kusatsu, Japan). The TaqMan MicroRNA assessment kit (Applied Biosystems, Thermo Fisher Scientific, USA) and the SYBR-Green-PCR kit (Takara Bio) were employed to analyze the qRT-PCR products. The 2-ΔΔCt formula was employed to evaluate the relative expressions following normalization to U6. Each experiment was run 3 times.
Cell Counting Kit-8 (CCK-8) assay
In each well of the 96-well plate, the cells were seeded at a density of 2×104/mL. At specific time points, a CCK-8 assay (Dojindo, Kumamoto, Japan) solution (10 µL) was added to each culture mixture. Following 2 h of incubation, a microplate reader was used to evaluate the absorbance of plates at 450 nm, which was followed by plotting the obtained absorbance values as a cell viability curve.
Flow cytometry
In the above-described cell transfection assay, the transfected cells that had been grown in the 96-well plate for counting purposes were then removed from the plate using trypsin reagent in the absence of ethylenediaminetetraacetic acid. The trypsinized cells were then washed with a cooled phosphate-buffered saline (PBS) solution, centrifuged, and then removed from the PBS solution. Then, an annexin V-fluorescein isothiocyanate (FITC) apoptosis discovery kit (BioLegend, Inc., San Diego, CA, USA) was employed for detecting the apoptotic process in cells. Resuscitation of cells was then carried out in 100 µL of buffer (1× binding buffer). At room temperature, resuspended cells were exposed to 5 µL of propidium iodide and annexin V-FITC in the absence of light for 15 minutes. Finally, for the analysis of the double-stained cells, the FACSCalibur Flow Cytometry instrument (BD Biosciences, Franklin Lakes, NJ, USA) was used. CellQuest v. 2 2.9 (BD Biosciences) was used for evaluating the proportion of apoptotic cells.
RNA immunoprecipitation (RIP)
The Magna RIP RNA-Binding Protein Immunoprecipitation Kit (MilliporeSigma, Burlington, MA, USA) was used for RIP assessment. The assay was performed strictly according to the kit instructions. To ensure that the observed signal originated from the precisely bound RNA, total RNA and the appropriate species of immunoglobin G (IgG; n=3) were employed as controls. In the anti-AGO2 RIP experiment, miRNA mimics were aberrantly transfected (using 2 µg/mL of anti-AGO2 antibody) into SK-OV-3 cells, which was followed by RIP cleavage 48 h after transfection.
The dual-luciferase reporter (DLR) assay
Bioinformatics methods were employed for the evaluation of probable miR-23a interactive sites on lncRNA GAS5 and the 3’-untranslated region (3'-UTR) of WT1. For the construction of a reporter vector, a sequence containing an estimated wild-type (WT) or mutant (MUT) miR-23a interacting site was cloned into the Pirlo reporter vectors (Promega, Madison, WI, USA). For the DLR assay, the cotransfection of SK-OV-3 cells was carried out with miR-23a mimics and reporter vector or negative control, and 48 h later, the luciferase activity was assessed with the DLR system (Promega).
Western blotting (WB)
Cell lysis was performed by using RIPA buffer with a protease inhibitor cocktail (1%). SDS-PAGE (10%) was employed to separate 40 g of protein. The proteins were then moved onto a polyvinylidene difluoride (PVDF) membrane (MilliporeSigma), which was subsequently obstructed for 2 h with skimmed milk (5%). The membrane incubation with primary antibodies was carried out for 24 h (at 4 ℃), which was followed by a 2-h incubation with the secondary antibodies. Electrochemiluminescence (ECL) was used to observe the bands, which were subsequently quantified using Image Software (National Institutes of Health, Bethesda, MD, USA). Immunoblotting was performed with the following antibodies: WT1 (1:1,000; MA5-32215; Thermo Fisher Scientific, USA), GAPDH (1:1,000; ab9485; Abcam, Cambridge, UK), and Goat Anti-Rabbit IgG H & L [horseradish peroxidase (HRP); 1:2,000; ab6721; Abcam]. Anti-mouse or anti-rabbit IgG (conjugated with HRP) was used as the secondary antibody.
Statistical analysis
All experiments were performed 3 times. The statistical evaluations were executed via SPSS v. 18.0 (IBM Corp., Armonk, NY, USA). Student t-test and 1-way analysis of variance (ANOVA) were implemented for examining variations between 2 groups and more than 2 groups accordingly. Following ANOVA, Dunnett post hoc test was performed. The link between the expression levels of lncRNA GAS5 and miR-23a was checked through using Pearson correlation coefficient. Values are expressed as mean ± standard deviation (SD). P values (2-tailed) <0.05, <0.01, <0.001, and <0.0001 (represented as * P, ** P, *** P, and **** P, respectively) were defined as statistically significant values.
Results
Decreased expression of lncRNA GAS5 predicted a favorable prognosis in OC
qRT-PCR was employed for the evaluation of lncRNA GAS5 expression in OC and match normal tissues. As seen in Figure 1A, lncRNA GAS5 expression in the tissues of OC was considerably decreased compared with neighboring normal tissues. Based on lncRNAGAS5 expression, the 60 OC tissues were classified into 2 subgroups according to a median value cutoff. A Kaplan-Meier (K-M) plot was employed to assess the survival of patients with OC. Patients with OC who had a low expression of the lncRNAGAS5 had a poorer survival rate (Figure 1B). Furthermore, we examined the link between GAS5 expression and clinicopathological characteristics in 60 patients with ovarian adenocarcinoma (OA). As illustrated in Table 1, the decreased expression of GAS5 was considerably linked with the International Federation of Gynecology and Obstetrics (FIGO) stage (P=0.0092), degree of tumor differentiation (P=0.0045), and lymph node metastasis (P=0.0201). Moreover, a considerable correlation was not detected between GAS5 expression and tumor size, age, or grade of malignancy. Taken together, we concluded that GAS5 is downregulated in OC, suggesting lncRNAGAS5 might be a promising prognostic biomarker.
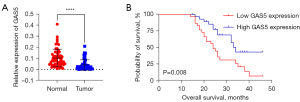
Table 1
Factors | N | GAS5 expression (n=60) | P value | |
---|---|---|---|---|
Low expression (n=30) | High expression (n=30) | |||
Age (year) | 0.4383 | |||
<50 | 29 | 16 | 13 | |
≥50 | 31 | 14 | 17 | |
Tumor size (cm) | 0.7952 | |||
≤4 | 33 | 16 | 17 | |
>4 | 27 | 14 | 13 | |
FIGO stage | 0.0092 | |||
I–II | 26 | 8 | 18 | |
III–IV | 34 | 22 | 12 | |
Differentiation | 0.0045 | |||
Poor | 31 | 21 | 10 | |
Well | 29 | 9 | 20 | |
Lymph node metastasis | 0.0201 | |||
Yes | 29 | 19 | 10 | |
No | 31 | 11 | 20 | |
Tumor grade | 0.6023 | |||
Low | 26 | 12 | 14 | |
High | 34 | 18 | 16 |
FIGO, International Federation of Gynecology and Obstetrics.
lncRNAGAS5 functioned as a sponge for miR-23a in SK-OV-3 cells
According to the obtained results, miR-23a expression was considerably elevated in OC tissue, indicating poorer survival in patients with OC, as indicated in Figure 2A,2B. We further determined the link between miR-23a expression and clinicopathological properties in 60 patients with OA. Increased miR-23a expression was considerably linked with FIGO stage (P=0.0018), degree of tumor differentiation (P=0.0201), and lymph node metastasis (P=0.0045), as indicated in Table 2. However, a statistically considerable link was not observed between the expression of miR-23a and age, tumor size, or grade. Moreover, in OC tissues, an inverse expression association was found between lncRNAGAS5 and miR-23a (Figure 2C). Using the online prediction software StarBase (https://starbase.sysu.edu.cn/), it was predicted that lncRNAGAS5 could be a target for miR-23a-5p. A possible miR-23a binding site in the 3'UTR of lncRNAGAS5 was located, as depicted in Figure 2D. Elevated expression of miR-23a decreased the activity of the WT-GAS5 reporter, according to the data obtained from the DLR assay. (Figure 2E). Moreover, RIP experiments revealed that miR-23a coprecipitated with GAS5, confirming the potent physical interactions between GAS5 and miR-23a (Figure 2F). These results show that lncRNAGAS5 has a direct relationship with miR-23a and acts as a molecular sponge for miR-23a in OC cells.
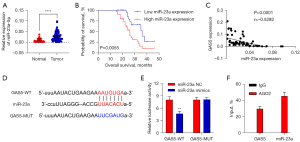
Table 2
Factors | N | miR-23a expression (n=60) | P value | |
---|---|---|---|---|
Low expression (n=30) | High expression (n=30) | |||
Age (year) | 0.7961 | |||
<50 | 29 | 14 | 15 | |
≥50 | 31 | 16 | 15 | |
Tumor size (cm) | 0.4363 | |||
≤4 | 33 | 15 | 18 | |
>4 | 27 | 15 | 12 | |
FIGO stage | 0.0018 | |||
I–II | 26 | 19 | 7 | |
III–IV | 34 | 11 | 23 | |
Differentiation | 0.0201 | |||
Poor | 31 | 11 | 20 | |
Well | 29 | 19 | 10 | |
Lymph node metastasis | 0.0045 | |||
Yes | 29 | 9 | 20 | |
No | 31 | 21 | 10 | |
Tumor grade | 0.6023 | |||
Low | 26 | 14 | 12 | |
High | 34 | 16 | 18 |
FIGO, International Federation of Gynecology and Obstetrics.
lncRNAGAS5 overexpression suppressed OC cell proliferation by interacting with miR-23a
SK-OV-3 cells were used in functional assays to determine the function of lncRNAGAS5. First, SK-OV-3 cells were transfected with pcDNA4.0 vector, pcDNA4.0-GAS5 vector, pcDNA4.0-GAS5 vector with miR-23a mimics, shCTRL, and shGAS5 or shGAS5 vector with miR-23a inhibitor, respectively. To identify the variations in the expressions of GAS5 and miR-23a, we performed qRT-PCR after transfection. We observed that a decrease in GAS5 mRNA expression (Figure 3A) caused by shGAS5 could be effectively reversed by cotransfection with miR-23a inhibitor. Following this, a CCK8 assessment was implemented to appraise the influence of lncRNAGAS5 on the proliferative potential of the cell. According to the obtained data, cellular proliferation was considerably attenuated in the GAS5 overexpression group relative to the negative control group (i.e., OE-NC in SK-OV-3 cells; P<0.001). Consequently, GAS5-knockdown cells grew faster relative to the shCTRL group (P<0.001). Moreover, these processes were significantly reversed through cotransfection of shlncRNAGAS5 along with either miR-23a inhibitor or pcDNA4.0-GAS5 vector with miR-23a mimics, as shown in Figure 3B. According to the EdU assay, elevated lncRNAGAS5 expression inhibited cell growth, while lncRNAGAS5 knockdown reversed these effects (Figure 3C). Similarly, inhibition of miR-23a could restore the proliferation capacity in lncRNAGAS5-depleted SK-OV-3 cells (Figure 3C), suggesting lncRNAGAS5 restrains cell proliferation through attenuating miR-23a-5p in OC. As a result, the lncRNAGAS5 increased the apoptotic potential of SK-OV-3 cells; however, suppression of miR-23a reversed these processes, as shown in Figure 3D. Wester blotting also revealed that upregulation of lncRNAGAS5 enhanced WT1 expression, but lncRNAGAS5 knockdown reversed these effects (Figure 3E). Moreover, attenuation of miR-23a could reverse the level of WT1 in lncRNA GAS-depleted SK-OV-3 cells (Figure 3E). These findings imply that lncRNAGAS5 functions as a cancer-suppressor lncRNA by interacting with miR-23a and preventing the development of OC.
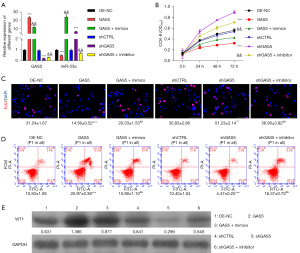
WT1 acted as a target gene of miR-23a
As described above, lncRNAs can competitively bind to miRNA to relieve the inhibition of miRNA on mRNA. According to this hypothesis, miRNAs are negatively correlated with lncRNAs or mRNAs; however, a positive correlation has been observed between mRNAs and lncRNAs. We assumed that lncRNAGAS5 may contribute to the development of OC through the targeted inhibition of WT1 expression by sponge adsorption of miR-23a. Consequently, qRT-PCR and Western blotting were performed to identify the WT1 expression. According to the obtained data, WT1 expression was considerably decreased in the tissues of OC relative to normal control tissues (Figure 4A,4B). An inverse expression correlation was detected between WT1 and miR-23a in OC tissues, as indicated in Figure 4C. By using the online platform TargetScan (http://www.targetscan.org/), we discovered that WT1 could be the miR-23a target gene. In the 3'UTR of WT1, we found a putative site of binding for miR-23a, as indicated in Figure 4D. Thus, miR-23a could suppress the luciferase activities of WT1 3'-UTR-WT plasmid in SK-OV-3 cells via transfection (Figure 4E). Moreover, RIP experiments showed that miR-23a coprecipitated with WT1, confirming that miR-23a binds with the 3'UTR of WT1 (Figure 4F). Hence, it was predicted that WT1 is a target gene of miR-23a.
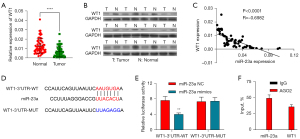
miR-23a inhibited proliferation and promoted WT1 expression through the miR-23a/WT1 pathway in SK-OV-3 cells
Functional and corresponding rescue assessments were conducted to identify the link between miR-23a and WT1. First, SK-OV-3 cells were transfected with miR-23a mimic NC, miR-23a mimics, pcDNA4.0-WT1 vector along with miR-23a mimics, miR-23a inhibitor NC, miR-23a inhibitor, or shWT1 vector along with miR-23a inhibitor, respectively. Posttransfection, Western blotting was performed to examine the variations in the expressions of WT1 and miR-23a. We observed that the cotransfection of WT1 overexpression vector with miR-23a mimics effectively reversed a decrease in WT1 protein expression levels (Figure 5A). The impact of miR-23a on cell proliferation was then determined via CCK8 assay. Cell proliferation was considerably elevated in the miR-23a overexpression group compared to the negative control group (mimics NC) in SK-OV-3 cells (P<0.001). miR-23a inhibited cells proliferated slower than the NC group (P<0.001) (Figure 5B). Similarly, the EdU assay indicated that miR-23a overexpression boosted cell growth while its inhibition lowered cell growth (Figure 5C). However, these processes were significantly reversed through cotransfection of WT1 overexpression vector along with miR-23a mimics or shWT1 vector along with miR-23a inhibitor, as demonstrated in Figure 5B,5C, suggesting that miR-23a promotes cell proliferation in OC through inhibiting WT1; in the same vein, the expression of miR-23a suppresses the apoptosis capability of SK-OV-3 cells (Figure 5D). However, the process was reversed by the cotransfection of the WT1 overexpression vector in SK-OV-3 cells (Figure 5D). These results suggest that miR-23a reduces proliferation and enhances WT1 expression in SK-OV-3 cells via the miR-23a/WT1 pathway.
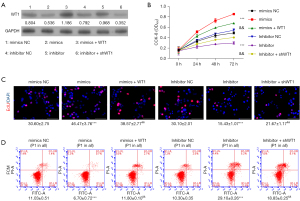
Discussion
Over recent decades, research into lncRNAs has yielded pivotal discoveries concerning the development and origin of a variety of human malignancies in (26,27). Some dysregulated lncRNAs, for example, are involved in multiple malignant pathways in OC (13,14,28). Hence, lncRNAs could be a productive target for OC diagnosis, treatment, and prognosis. Although the role of several types of lncRNAs have been clarified, further advanced exploration is necessary to elucidate the function of lncRNAs in OC. We therefore conducted this study to determine the expression and roles of lncRNAGAS5 in OC. We further aimed to clarify how lncRNAGAS5 controls OC cell oncogenicity in vitro.
Although the most studied and, apparently, the most widespread mechanism of action of lncRNAs in OC according to the competing endogenous RNAs (ceRNAs) model is interaction with mRNA of protein genes mediated by various miRNAs, in some other cases, their effect is associated with direct interaction with proteins or mRNA. Some lncRNAs, such as GAS5, has been shown to have different mechanisms of action (29). GAS5 is a lncRNA molecule that regulates the apoptotic, proliferative, invasive, and metastatic potential of cells across various human malignancies (30). The expression of lncRNA GAS5, a tumor-suppressor gene, is reduced in several malignancies, including breast (31), prostate (32), gastric (33), and lung cancer (34). lncRNA GAS5 was found to enhance the apoptotic process in TNBC through targeting miR-378a-5p/SUFU signaling (21). Furthermore, lncRNAGAS5 has been recognized as a critical regulator in the progression of lung cancer, and decreased expression of GAS5 has been found to considerably accelerate non-small cell lung cancer (NSCLC) growth, migration, and invasion (34). These studies demonstrate that lncRNAGAS5 figures prominently in various malignancies and is highly linked to the survival rate of patients with cancer.
However, few studies to date have examined the function and expression of lncRNAGAS5 in OC. Recently, Long and colleagues demonstrated that lncRNA GAS5 is substantially downregulated in OC tissues and that this is associated with poor prognosis, whereas lncRNA GAS5 suppresses cis-platinum (DDP) resistance and tumor growth in epithelial OC through the GAS5-E2F4-PARP1-MAPK axis (35). GAS5 suppressive effects on cell proliferation, viability, as well as migration, invasion, and EMT were established by functional studies in vitro using OC-derived A2780 cells and in vivo with animal models. Direct interactions in both axes were validated using expression-modulating studies and the DLR gene assay as well (29). The related data showed consistency with our results, as we found decreased expression of lncRNAGAS5 in OC. Furthermore, the expression of lncRNAGAS5 was relatively lower in SK-OV-3 cell lines compared to controls. Overexpression of the lncRNA GAS5 gene facilitated the apoptotic process while decreasing the proliferative potential of the OC cells. Thus, lncRNA GAS5 could be used as a potential biomarker for patient risk stratification and local regional metastasis in OC.
According to several recent studies, lncRNAs are composed of miRNA responsive elements (36,37) that act as a molecular sponge for miRNAs, protecting mRNAs from degradation in several malignancies (38). As a result of this interaction between lncRNA and miRNA, pathogenic processes are triggered during cancer progression (39,40). In our study, miR-23a was found to be the miRNA target for lncRNAGAS5. This finding was further validated by a DLR assay, which confirmed the binding between lncRNAGAS5 and miR-23a in OC. Several investigations have revealed an elevated expression of miR-23a in several carcinomas, including colorectal cancer (41). Reduced expression of miR-23 was found to increase the apoptotic capacity of OC stem cells while decreasing their migration capacity (42). WT1 is a tumor-suppressor gene that has been found in several malignancies, including breast, hepatocellular, and OC (43,44). Our findings indicate that WT1 inhibits the progression of OC. We demonstrated that the lncRNAGAS5-miR-23a axis is able to regulate WT1 expression in OC, which adds to our understanding of the regulatory mechanism of WT1.
Our study indicated that the lncRNAGAS5-miR-23a axis contributes to the regulation of WT1 expression in OC cells. We further demonstrated that through enhancing the outputs of the miR-23a-WT1 axis, the effects on OC cell proliferation and apoptosis caused by lncRNAGAS5 silencing can be reversed, meaning that thwe miR-23a/WT1 cascade is critical for OC progression facilitated by lncRNAGAS5. Generally, lncRNA GAS5 is a tumor-suppressor gene targeting the miR-23a-WT1 axis and hence impedes the malignant behavior of OC.
Conclusions
Our study shows that lncRNAGAS5 inhibits OC progression by upregulating WT1 and attenuating miR-23a, implying that lncRNAGAS5 can be considered a new therapeutic target in the treatment of OC.
Acknowledgments
Funding: This study was supported by the Natural Science Foundation of Guangdong Province (No. 2018A030313594).
Footnote
Reporting Checklist: The authors have completed the MDAR reporting checklist. Available at https://atm.amegroups.com/article/view/10.21037/atm-22-6394/rc
Data Sharing Statement: Available at https://atm.amegroups.com/article/view/10.21037/atm-22-6394/dss
Conflicts of Interest: All authors have completed the ICMJE uniform disclosure form (available at https://atm.amegroups.com/article/view/10.21037/atm-22-6394/coif). The authors have no conflicts of interest to declare.
Ethical Statement: The authors are accountable for all aspects of the work, including ensuring that any questions related to the accuracy or integrity of any part of the work have been appropriately investigated and resolved. The study was conducted in accordance with the Declaration of Helsinki (as revised in 2013). All patients who participated in this study signed an informed consent form, and this study was approved by the Ethics Committee of The Sixth Affiliated Hospital of Sun Yat-sen University (No. 2019ZSLYEC-040).
Open Access Statement: This is an Open Access article distributed in accordance with the Creative Commons Attribution-NonCommercial-NoDerivs 4.0 International License (CC BY-NC-ND 4.0), which permits the non-commercial replication and distribution of the article with the strict proviso that no changes or edits are made and the original work is properly cited (including links to both the formal publication through the relevant DOI and the license). See: https://creativecommons.org/licenses/by-nc-nd/4.0/.
References
- Siegel RL, Miller KD, Jemal A. Cancer statistics, 2020. CA Cancer J Clin 2020;70:7-30. [Crossref] [PubMed]
- Zhan S, Yung MMH, Siu MKY, et al. New Insights into Ferroptosis Initiating Therapies (FIT) by Targeting the Rewired Lipid Metabolism in Ovarian Cancer Peritoneal Metastases. Int J Mol Sci 2022;23:15263. [Crossref] [PubMed]
- Matulonis UA, Sood AK, Fallowfield L, et al. Ovarian cancer. Nat Rev Dis Primers 2016;2:16061. [Crossref] [PubMed]
- Zhao H, Xu Y, Shang H. Ferroptosis: A New Promising Target for Ovarian Cancer Therapy. Int J Med Sci 2022;19:1847-55. [Crossref] [PubMed]
- Liu L, Ding L, Zhang L, et al. Mechanism of periplakin on ovarian cancer cell phenotype and its influence on prognosis. Transl Cancer Res 2022;11:1372-85. [Crossref] [PubMed]
- Matsui M, Corey DR. Non-coding RNAs as drug targets. Nat Rev Drug Discov 2017;16:167-79. [Crossref] [PubMed]
- Sanchez Calle A, Kawamura Y, Yamamoto Y, et al. Emerging roles of long non-coding RNA in cancer. Cancer Sci 2018;109:2093-100. [Crossref] [PubMed]
- Ning S, Li X. Non-coding RNA Resources. Adv Exp Med Biol 2018;1094:1-7. [Crossref] [PubMed]
- Chi Y, Wang D, Wang J, et al. Long Non-Coding RNA in the Pathogenesis of Cancers. Cells 2019;8:1015. [Crossref] [PubMed]
- Sharma U, Barwal TS, Malhotra A, et al. Long non-coding RNA TINCR as potential biomarker and therapeutic target for cancer. Life Sci 2020;257:118035. [Crossref] [PubMed]
- Zhang W, Guan X, Tang J. The long non-coding RNA landscape in triple-negative breast cancer. Cell Prolif 2021;54:e12966. [Crossref] [PubMed]
- Meng C, Zhou JQ, Liao YS. Autophagy-related long non-coding RNA signature for ovarian cancer. J Int Med Res 2020;48:300060520970761. [Crossref] [PubMed]
- Lou W, Ding B, Zhong G, et al. Dysregulation of pseudogene/lncRNA-hsa-miR-363-3p-SPOCK2 pathway fuels stage progression of ovarian cancer. Aging (Albany NY) 2019;11:11416-39. [Crossref] [PubMed]
- Liang H, Yu T, Han Y, et al. LncRNA PTAR promotes EMT and invasion-metastasis in serous ovarian cancer by competitively binding miR-101-3p to regulate ZEB1 expression. Mol Cancer 2018;17:119. [Crossref] [PubMed]
- Li Y, Lv M, Wang J, et al. LINC00641 inhibits the proliferation and invasion of ovarian cancer cells by targeting miR-320a. Transl Cancer Res 2021;10:4894-904. [Crossref] [PubMed]
- Ye L, Fan T, Qin Y, et al. MicroRNA-455-3p accelerate malignant progression of tumor by targeting H2AFZ in colorectal cancer. Cell Cycle 2022; Epub ahead of print. [Crossref]
- Mazziotta C, Cervellera CF, Lanzillotti C, et al. MicroRNA dysregulations in Merkel cell carcinoma: molecular mechanisms and clinical application. J Med Virol 2023;95:e28375. [Crossref] [PubMed]
- Ismail A, Abulsoud AI, Fathi D, et al. The role of miRNAs in ovarian cancer pathogenesis and therapeutic resistance - A focus on signaling pathways interplay. Pathol Res Pract 2022;240:154222. [Crossref] [PubMed]
- Cui M, Liu Y, Cheng L, et al. Research progress on anti-ovarian cancer mechanism of miRNA regulating tumor microenvironment. Front Immunol 2022;13:1050917. [Crossref] [PubMed]
- Ye Y, Li SL, Wang SY. Construction and analysis of mRNA, miRNA, lncRNA, and TF regulatory networks reveal the key genes associated with prostate cancer. PLoS One 2018;13:e0198055. [Crossref] [PubMed]
- Zheng S, Li M, Miao K, et al. lncRNA GAS5-promoted apoptosis in triple-negative breast cancer by targeting miR-378a-5p/SUFU signaling. J Cell Biochem 2020;121:2225-35. [Crossref] [PubMed]
- Ni W, Yao S, Zhou Y, et al. Long noncoding RNA GAS5 inhibits progression of colorectal cancer by interacting with and triggering YAP phosphorylation and degradation and is negatively regulated by the m(6)A reader YTHDF3. Mol Cancer 2019;18:143. [Crossref] [PubMed]
- Li X, Li Y, Wang Y, et al. The m(6)A demethylase FTO promotes renal epithelial-mesenchymal transition by reducing the m(6)A modification of lncRNA GAS5. Cytokine 2022;159:156000. [Crossref] [PubMed]
- Wang H, Wang D, Shen Y, et al. GAS5 attenuates the malignant progression of glioma stem-like cells by promoting E-cadherin. Cancer Gene Ther 2022; Epub ahead of print. [Crossref]
- Fang X, Zhong G, Wang Y, et al. Low GAS5 expression may predict poor survival and cisplatin resistance in cervical cancer. Cell Death Dis 2020;11:531. [Crossref] [PubMed]
- Peng WX, Koirala P, Mo YY. LncRNA-mediated regulation of cell signaling in cancer. Oncogene 2017;36:5661-7. [Crossref] [PubMed]
- Bhan A, Soleimani M, Mandal SS. Long Noncoding RNA and Cancer: A New Paradigm. Cancer Res 2017;77:3965-81. [Crossref] [PubMed]
- Du W, Feng Z, Sun Q. LncRNA LINC00319 accelerates ovarian cancer progression through miR-423-5p/NACC1 pathway. Biochem Biophys Res Commun 2018;507:198-202. [Crossref] [PubMed]
- Braga EA, Fridman MV, Moscovtsev AA, et al. LncRNAs in Ovarian Cancer Progression, Metastasis, and Main Pathways: ceRNA and Alternative Mechanisms. Int J Mol Sci 2020;21:8855. [Crossref] [PubMed]
- Yang X, Xie Z, Lei X, et al. Long non-coding RNA GAS5 in human cancer. Oncol Lett 2020;20:2587-94. [Crossref] [PubMed]
- Filippova EA, Fridman MV, Burdennyy AM, et al. Long Noncoding RNA GAS5 in Breast Cancer: Epigenetic Mechanisms and Biological Functions. Int J Mol Sci 2021;22:6810. [Crossref] [PubMed]
- Lin CY, Wang SS, Yang CK, et al. Impact of GAS5 genetic polymorphism on prostate cancer susceptibility and clinicopathologic characteristics. Int J Med Sci 2019;16:1424-9. [Crossref] [PubMed]
- Liu Y, Yin L, Chen C, et al. Long non-coding RNA GAS5 inhibits migration and invasion in gastric cancer via interacting with p53 protein. Dig Liver Dis 2020;52:331-8. [Crossref] [PubMed]
- Dong L, Li G, Li Y, et al. Upregulation of Long Noncoding RNA GAS5 Inhibits Lung Cancer Cell Proliferation and Metastasis via miR-205/PTEN Axis. Med Sci Monit 2019;25:2311-9. [Crossref] [PubMed]
- Long X, Song K, Hu H, et al. Long non-coding RNA GAS5 inhibits DDP-resistance and tumor progression of epithelial ovarian cancer via GAS5-E2F4-PARP1-MAPK axis. J Exp Clin Cancer Res 2019;38:345. [Crossref] [PubMed]
- Chan JJ, Tay Y. Noncoding RNA:RNA Regulatory Networks in Cancer. Int J Mol Sci 2018;19:1310. [Crossref] [PubMed]
- Zhang Y, Xu Y, Feng L, et al. Comprehensive characterization of lncRNA-mRNA related ceRNA network across 12 major cancers. Oncotarget 2016;7:64148-67. [Crossref] [PubMed]
- Russo F, Fiscon G, Conte F, et al. Interplay Between Long Noncoding RNAs and MicroRNAs in Cancer. Methods Mol Biol 2018;1819:75-92. [Crossref] [PubMed]
- Fan CN, Ma L, Liu N. Systematic analysis of lncRNA-miRNA-mRNA competing endogenous RNA network identifies four-lncRNA signature as a prognostic biomarker for breast cancer. J Transl Med 2018;16:264. [Crossref] [PubMed]
- Wang W, Lou W, Ding B, et al. A novel mRNA-miRNA-lncRNA competing endogenous RNA triple sub-network associated with prognosis of pancreatic cancer. Aging (Albany NY) 2019;11:2610-27. [Crossref] [PubMed]
- Lee Y, Kim SJ, Choo J, et al. miR-23a-3p is a Key Regulator of IL-17C-Induced Tumor Angiogenesis in Colorectal Cancer. Cells 2020;9:1363. [Crossref] [PubMed]
- Zhuang RJ, Bai XX, Liu W. MicroRNA-23a depletion promotes apoptosis of ovarian cancer stem cell and inhibits cell migration by targeting DLG2. Cancer Biol Ther 2019;20:897-911. [Crossref] [PubMed]
- Zhang Y, Yan WT, Yang ZY, et al. The role of WT1 in breast cancer: clinical implications, biological effects and molecular mechanism. Int J Biol Sci 2020;16:1474-80. [Crossref] [PubMed]
- Chau YY, Hastie ND. The role of Wt1 in regulating mesenchyme in cancer, development, and tissue homeostasis. Trends Genet 2012;28:515-24. [Crossref] [PubMed]