Metabotropic glutamate receptor-8 relieves neonatal maternal separation-induced visceral hypersensitivity in rats by regulating expression of TNF-α
Highlight box
Key findings
• MGluR8 might be a potential target for the treatment of visceral hypersensitivity (VH).
What is known and what is new?
• (S)-3,4-DCPG ameliorated colonic distension (CRD)-induced VH while MSOP partially aggravated that.
• MGluR8 relieved neonatal maternal separation (NMS)-induced VH in rats by regulating TNF-α.
What is the implication, and what should change now?
• MGluR8 might be a therapeutic target for the amelioration of VH.
Introduction
As one of the most common types of gastrointestinal diseases, irritable bowel syndrome (IBS) is characterized by persistent or intermittent abdominal pain and discomfort as well as changes of bowl movements (1). In patients with IBS, lower volumes of rectal distention can cause pain and the activation of mast cells in colon mucosa (2). It was reported that the activated mast cells, which are closed to submucosal nerve fibers, have correlation with the frequency and severity of abdominal pain in IBS patients (3). Additionally, the dysfunction of gut-brain axis in IBS alters the reflective and perceptual nervous system reactions (4). The pathogenesis of IBS can be heterogenous and many factors such as visceral hypersensitivity (VH) and chronic inflammation can contribute to the disease development (5). Currently, VH is regarded as an essential feature and keystone of IBS, which is affected by various social and mental stress factors and also reflects the brain-gut axis regulation abnormality of intestinal nociceptive processing (6). Patients with VH are more sensitive to luminal stimuli and therefore have enhanced perception of visceral discomfort (7,8). To date, many targets in the gut-brain pathways and regional neuroimmune pathways have been identified for the treatment of VH such as histamine-1 receptors, tachykinin ligands, and opioid receptors (9). A case of previous study demonstrated that neonatal maternal separation (NMS) could exaggerate neurochemical responses and visceral hyperalgesia to colonic distension (10). Additionally, NMS could contribute to the imbalance of neuroinflammation (11). As a well-established early-life stress model, NMS has been widely used in rats to establish animal models to recapitulate human VH (6,12).
As an important excitatory neurotransmitter in the central nervous system (CNS), L-Glutamate can mediate the formation of visceral hypersensitivity after inflammation through activating the ionotropic glutamate receptors (iGluRs) (13). The metabotropic glutamate receptors (mGluRs) can be further characterized into 3 subtypes: group I (mGlu1and 5), group II (mGlu2 and 3), and group III (mGlu4, 6–8) (13). Among these 3 subgroups, relatively little was known about group III receptors until a case reported in a recent study showed that this type of receptors might induce antidepressant-like effects in rodents (14). (S)-3,4-dicarboxyphenylglycine (DCPG) is a selective orthosteric agonist for mGluR8 with a >100-fold sensitivity compared to other group III mGluRs (15). Currently, no specific antagonist for mGluR8 has been identified and (RS)-α-methylserine-O-phosphate (MSOP), a pan group III mGluR antagonist, has often been used in experiments to observe the effect of mGluR8 antagonization (16). It has been well known that glutamate plays an important role in nociceptive processing. Ionotropic and metabotropic glutamate receptors are expressed in different regions of organs that are involved in pain sensation and transmission. It was reported that mGlu receptors can be activated in the process of pain sensitization (17). Evidence has indicated that group III mGluRs have systemic antihyperalgesic effects (18). Previous research showed that both systemic and intra-PAG DCPG treatments were antinociceptive in inflammatory and neuropathic pain mouse models (15). Our previous experiments revealed that activating mGluR7 ameliorated VH in NMS rats (6). Enteric nervous system could regulate the secretion and absorption of the intestine (19). In addition, evidence indicates that the mGluR8 protein is expressed in the enteric nervous system of many species such as rat, guinea pig, and human (20). Interestingly, it was evidenced that mGluR8 could relieve pain (21,22). However, few studies have investigated the role of mGluR8 on VH in MHS rats.
Accumulating evidence indicates that intestinal inflammation has a close association with the pathogenesis of VH (23). Even though robust inflammation may be absent in patients with post-infectious IBS, the number of epithelial T lymphocytes and mast cells is often increased in their gastrointestinal (GI) tract (24), indicating a persistent inflammatory status which may contribute to the pathogenesis of colonic hypersensitivity. Many cytokines such as interleukin-1β (IL-1β), tumor necrosis factor-α (TNF-α), and interleukin-10 (IL-10) have been known to be associated with the development of IBS (6) and have been well studied in IBS animal models along with signal pathways such as pregnane X receptor (PXR)/nuclear factor kappaB (NF-κB), toll-like receptor 4 (TLR4) (25-27).
To better understand the role of mGluR8 in VH, we constructed an NMS model and explored the therapeutic role of this receptor and possible molecular mechanisms. We present the following article in accordance with the ARRIVE reporting checklist (available at https://atm.amegroups.com/article/view/10.21037/atm-22-6452/rc).
Methods
Animals and neonatal maternal separation
A total of 14 15-day pregnant Sprague-Dawley rats were acquired from a qualified vendor (Nanjing, China). All rats were kept in verified specific room at 25 °C with a 12-h day/night cycle and were given free access to water and food. Animal experiments were performed in the Hangzhou Hibio Technology Co., Ltd under a project license (No. HB2007020) granted by the Ethics Board of Hangzhou Hibio Technology Co., Ltd. (Hangzhou, China), in compliance with Hangzhou Hibio Technology Co., Ltd guidelines for the care and use of animals. A protocol was prepared before the study without registration.
Neonatal rats were grouped by 6 per dam on postnatal day 2 (P2) and were randomly assigned to 6 groups: control (NC) group, (S)-3,4-DCPG group (3 mg/kg), (S)-3,4-DCPG group (10 mg/kg), MSOP group (3 mg/kg), MSOP group (10 mg/kg) (n=6/group), and NMS sham treatment group. To develop the NMS model, neonatal rats were separated from their cage and kept in fresh new cages in an adjacent room for 3 hours before being returned to their original cages afterwards on postnatal days (P)2–14. Neonatal rats in the NC group stayed in their original cages until weaned. The sex of the rats was identified on P22 and only data of male rates were collected for further analysis. Eventually, a total of 36 male rats weighting 250–300 g were included in this study.
Abdominal withdrawal reflex
At week 8, colorectal distension (CRD)-associated VH was determined by the abdominal withdrawal reflex (AWR), which measures involuntary visceromotor reflexes. The experiment was carried out following a conventional protocol. Briefly, all rats were starved for 12 hours before receiving mild anesthetization with 2% isoflurane (in oxygen, 0.5 L/min) in a sealed cage. Next, a 6-cm-long flexible latex balloon was inserted in their distal colon and maintained for 15 minutes. Then, the CRD was introduced by fast inflating of the balloon with air to predefined pressures of 20, 40, 60, or 80 mmHg, respectively, and maintained for a 20 second period. Each measurement was repeated thrice at an interval of 5 minutes. The AWR results were defined as follows: 0 refers to no response at all pressure; 1 refers to gentle movement of head but showing no contraction of abdominal muscles; 2 refers to obvious abdominal muscle contraction; 3 refers to abdominal wall elevation; 4 refers to body arching and pelvic elevation (28).
Electromyography
CRD-induced VH was also measured by electromyography (EMG) at week 8. Briefly, animals were anesthetized with 30–40 mg/kg 3% sodium pentobarbital through intraperitoneal (i.p.) injection. Next, EMG electrodes were placed at rats’ lower left abdominal and were connected subcutaneously and secured at the back of their neck (29). After 5 days, rats were starved for 12 hours and anesthetized with isoflurane before inserting the deflated latex balloon as mentioned previously. The EMG was measured via the implanted electrodes continuously using a BL-420F system (Chengdu-Techman Software, Chengdu, China). Different pressures including 20, 40, 60, and 80 mmHg were applied to the balloon inflation to record the EMG signal. All experiments were repeated 3 times. Next, the area under the curve (AUC) of each EMG data was plotted and the results were calculated following the formula ΔAUC (AUC during CRD- baseline AUC) to determine the strength of visceromotor reflex (6).
Administration of mGluR8 agonists and antagonists
The selective mGluR8 agonist (S)-3,4-DCPG (cat. no. 1302; Tocris Bioscience, Bristol, UK) and antagonists MSOP (cat. no. 0803; Tocris Bioscience) were dispersed in a suspension of 0.5% methylcellulose (Sigma-Aldrich, Stenheim, Germany). The NMS animal models were randomly assigned to 5 different groups, with 4 groups being dosed intraperitoneally with (S)-3,4-DCPG (3 mg/kg), MSOP (3 mg/kg), (S)-3,4-DCPG (10 mg/kg), or MSOP (10 mg/kg) 1 hour before AWR and EMG experiments. Vehicle control of phosphate-buffered saline (PBS) with the same volume was given to rats in the NMS sham experiment group (8,30).
Tissue preparation
At the end of the experiment, all animals were sacrificed after receiving anaesthetization with 3% pentobarbital sodium (30 mg/kg i.p.) and their distal colons were collected and snap-frozen in liquid nitrogen before storing at −70 °C. Next, the colon samples were taken to Jinhua Central Hospital for further analysis.
Real-time quantitative polymerase chain reaction
The total RNA was extracted using the TRIzol® reagent (Invitrogen, Carlsbad, CA, USA) following product instruction and the PrimeScript™ RT reagent kit (Takara Bio, Shiga, Japan) was used to reverse transcribe the RNA into complementary DNA (cDNA). The quantitative real-time polymerase chain reaction (qRT-PCR) was conducted with cDNA using an ABI 7500 Sequence with SYBR Green (Takara Bio, Inc.). The sequences of the primers (TSINGKE Biological Technology, Beijing, China) were as follows: mGluR8: 5'-ACCAAACATCAACCGCACA-3' (F), 5'- CTAAGTTCCCGCCCAGAAG -3' (R); TNF-α: 5'-GTCGTAGCAAACCACCAAGTG-3' (F), 5'-CTCCCTCCTGGCTGGTCCCTTGGTG-3' (R); IL-1β: 5'-GACTTCACCATGGAACCCGT-3' (F); 5'-CAGGGAGGGAAACACACGTT-3' (R); IL-6:5'-CTGGTCTTCTGGAGTTCCGTT-3' (F); 5'-GCATTGGAAGTTGGGGTAGGA-3' (R); GAPDH: 5'-AGATCCACAACGGATACATT-3' (F), 5'- TCCCTCAAGATTGTCAGCAA -3' (R). Glyceraldehyde 3-phosphate dehydrogenase (GAPDH) was used as the house keeping gene and the data were calculated using the 2−△△CT method. The expression levels of target genes were normalized to GAPDH.
Western blotting (WB)
The WB was conducted following a conventional protocol. Briefly, the total protein was separated by 10% sodium dodecyl sulfate polyacrylamide gel electrophoresis (SDS-PAGE) gel before transferring onto nitrocellulose membrane (Whatman, Kent, UK). Next, the membrane was blocked with 5% non-fat milk at room temperature for 1 hour and rinsed before incubating with primary antibodies overnight at cold room (rabbit anti-mGluR8 1:500, cat. no. A06589; Boster Biological Technology, Wuhan, China; rabbit anti-NF-kB P65 1:1,000, cat. no. 8242; Cell Signaling Technology (CST), Danvers, MA, USA; rabbit anti-TLR4 1:500, cat. no. 19811-1-AP; Proteintech Group, Rosemont, IL, USA). Next, the membrane was rinsed with tris-buffered saline (TBS) for three times and stained with a second antibody which was conjugated to horseradish peroxidase (HRP; cat. no. KGAA35; Jiangsu KeyGEN BioTECH, Jiangsu, China) for 2 hours at room temperature. After rinsing, the images were acquired using a G: BOX chemiXR5 system (Syngene, Bangalore, India) and measured based on their grey value that normalized to GAPDH.
Immunohistochemistry
Slides were deparaffinized in xylene and rehydrated with PBS containing 1.5% H2O2 for 30 minutes. The slides were then stained with primary antibodies (rabbit anti-mGluR8 1:50, cat. no. A06589; Boster Biological Technology, China; rabbit anti-myeloperoxidase (MPO) 1:1,000, cat. no. ab208670; Abcam plc, UK; rabbit anti-CD3 1:100, cat. no. ab5690; Abcam plc, UK; rabbit anti-CD68 1:200, cat. no. ab125212; Abcam plc, UK). After thorough rinsing, the secondary antibody (goat anti-rabbit) was applied to the slide and incubated for 1 hour at room temperature. After staining with the HRP-labelled rabbit IgG fraction and 3,3-diaminobenzidine (DAB), the slide was counterstained with hematoxylin and eosin (H&E) and visualized with a microscope (Olympus, Tokyo, Japan). The FluoView software (FV1000, Olympus Corp.) was then used to obtain the image. To determine the expression level of target protein, 10 fields across each section were randomly chosen and examined at 400× magnification. The average optical density (AOD) of positive cells was measured by ImageJ (National Institutes of Health, Bethesda, MD, USA).
Statistical analysis
All statistical analyses were conducted using SPSS 19.0 software (IBM Corp., Armonk, NY, USA). All results were shown as mean ± standard deviation (SD) and compared by student’s t-test or a one-way analysis of variance (ANOVA) as appropriate. Statistical significance was considered when the P value was less than 0.05.
Results
Establishment of VH rat model
The level of VH was measured by the AWR score and EMG response. The data showed that the rat model was successfully established and presented with increased VH compared to NC rats, both in AWR score (Figure 1A, P<0.05 in 40 mmHg, P<0.01 in 60 mmHg and in 80 mmHg) and EMG response (Figure 1B, P<0.001 in 20 mmHg, 60 mmHg, and 80 mmHg, P<0.05 in 40 mmHg). The VH rat model was successfully induced and then used to detect the effect of mGluR8 on VH.
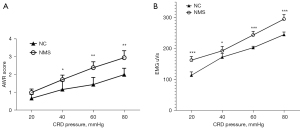
The expression of mGluR8 was enhanced in colons of NMS rats
We discovered that most mGluR8 expression was in the lamina propria of the mucosa layer (Figure 2A). To determine whether NMS was related to mGluR8, we examined the expression of mGluR8 in colon tissues. Our data showed that compared to the controls, the expression of mGluR8 was elevated in NMS rats (Figure 2B, P<0.05). The mRNA and protein expression of mGluR8 was also elevated in the NMS rats compared to the controls (Figure 2C, P<0.05 and Figure 2D,2E, P<0.01). These data indicated that the VH in NMS rats might be related with mGluR8 overexpression.
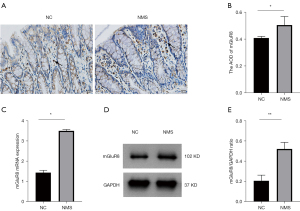
(S)-3,4-DCPG ameliorated whereas MSOP partially aggravated CRD-induced VH
To determine the role of mGluR8 on VH, different doses of (S)-3,4-DCPG or MSOP were injected to NMS rats intraperitoneally. First, WB was used to confirm the specificity of these 2 molecules to mGluR8. The protein expression of mGluR8 in (S)-3,4-DCPG groups was higher than in NMS rats, significantly in the dose of 10 mg/kg (Figure 3A,3B, P<0.01). Furthermore, we found that the protein expression of mGluR8 in MSOP groups were lower than that in NMS rats, significantly in the dose of 10 mg/kg (Figure 3A,3B, P<0.05). The agonism of (S)-3,4-DCPG and the antagonism of MSOP were significant. Then, the effect of mGluR8 was tested by AWR score and EMG response to CRD pressure. The experimental design of using (S)-3,4-DCPG and typical change of EMG responses to CRD is shown in Figure 3C. A dose of 10 mg/kg of (S)-3,4-DCPG remarkably decreased the AWR score compared to that of NMS rats (Figure 3D), significantly in the dose of 3 mg/kg at 40 and 60 mmHg, and at the dose of 10 mg/kg at 40, 60, and 80 mmHg. Similarly (S)-3,4-DCPG attenuated the EMG response compared to NMS rats (Figure 3E), significantly in the dose of 3 mg/kg at 20 and 60 mmHg and at the dose of 10 mg/kg with all pressures.
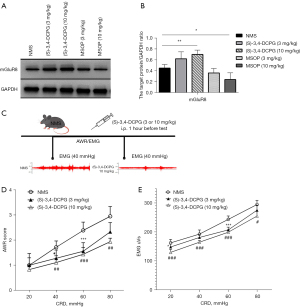
The experimental design of using MSOP and typical change of EMG responses to CRD is shown in Figure 4A. MSOP at the doses of 3 or 10 mg/kg showed no significant effect measured by the AWR score (Figure 4B). In contrast, MSOP aggravated the EMG response compared with NMS rats (Figure 4C), significantly in the dose of 10 mg/kg at 40 and 60 mmHg (P<0.01).
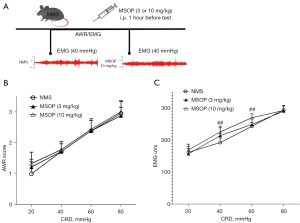
Therefore, inhibition or activation of mGluR8 expression regulated VH in NMS rats following a dose-dependent manner.
(S)-3,4-DCPG (10 mg/kg) reduced MPO expression in intestinal mucosa of NMS rats
Altering the intestinal microbiota can trigger the intestinal mucosal immune system and lead to persistent mild local inflammation which eventually results in IBS (31). Furthermore, the number of mucosal immune cells is often increased in IBS (32). As a surrogate parameter, colonic MPO is often evaluated to determine the severity of local inflammation (33). We found that the MPO expression in NMS rats was increased compared to that of NC rats and most expressions were identified in the laminae propria mucosae (Figure 5A). The structure of the mucosa was basically normal, which was consistent with the IBS model of subclinical or nonspecific low-grade inflammation in the intestinal mucosa. The (S)-3,4-DCPG (10 mg/kg) group exhibited markedly decreased MPO level compared to that of the NMS group (Figure 5A,5B, P<0.05). Meanwhile 3 or 10 mg/kg MSOP treatment had no significant change on the rat’s colon. The CD3 expression in the (S)-3,4-DCPG and the MSOP groups did not differ from that in the NMS group (Figure 5A,5B). Additionally, CD68 expression in the intestinal mucosa was rare in all groups, and statistical analysis was difficult. Following the results of (S)-3,4-DCPG (10 mg/kg) alleviating the low-grade inflammation, we next explored the related inflammatory signaling pathway.
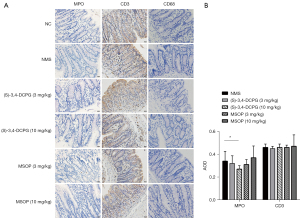
(S)-3,4-DCPG decreased TNF-α expression in NMS rats, MSOP increased the expression of TNF-α in NMS rats
We found that (S)-3,4-DCPG (10 mg/kg) treatment reduced the expression of MPO in intestinal mucosa of NMS rats. We further measured the levels of some IBS-associated inflammatory cytokines such as TNF-α, IL-1β, and IL-6 by RT-qPCR and discovered that the (S)-3,4-DCPG (3 mg/kg) treatment decreased TNF-α expression compared to controls (Figure 6A, P<0.05) whereas the MSOP (3 mg/kg) treatment increased TNF-α expression (Figure 6A, P<0.05). The IL-1β and IL-6 levels did not significantly change upon treatments (Figure 6A). The TLR4 /NF-κB signaling pathway has been testified to be a critical regulator in inflammatory responses in many diseases (34,35). Therefore, we examined the activation of the TLR4/NF-κB signaling pathway in the present study by WB. We did not observe statistically significant changes of TLR4 expression (Figure 6B,6C) and the expression of NF-κB seemed increased in the (S)-3,4-DCPG group and decreased in the MSOP group compared to the NMS group. However, both the results were not statistically significant.
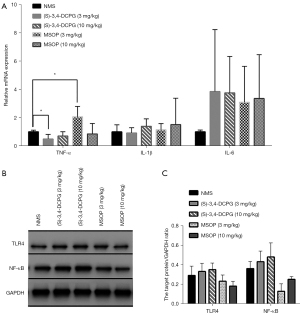
Discussion
In this study, we established a VH rat model by NMS and discovered that the expression level of mGluR8 was increased in the colons. Activation of mGluR8 could ameliorate CRD-induced VH and in contrast, inhibition of mGluR8 could partially aggravate VH (only in EMG response). Additionally, activation of mGluR8 reduced MPO expression in the colons and attenuated VH by inhibiting the secretion of TNF-α; in contrast, antagonizing mGluR8 partially aggravated VH through the promotion of TNF-α.
As a group of critical modulators for excitatory functions, metabotropic glutamate receptors are commonly distributed through the CNS. Metabotropic glutamates (mGlus) regulate glutamatergic and GABAergic neurotransmission through G protein coupling to the second messenger pathway (36). Outside of the CNS, recent study showed that group III mGlu receptors are also located in human stomach and colon which provide a novel therapeutic target for many diseases such as gastrointestinal dysfunction (37). Tong and Kirchgessner reported that mGlu7/mGlu8 expression was strong in rat gastrointestinal (GI) tract and mGlu8 receptor agonist could trigger longitudinal muscle contraction in the colon of guinea pigs (20). However, the role of mGluR8 in VH in the colon has not been studied.
Our study showed that the expression of mGluR8 was increased in NMS rats in both RNA and protein levels. NMS simulated early IBS, and the VH model was created. Therefore, mGluR8 may participate in the VH of IBS.
A case of previous study has shown that mGluR8 knockout mice often present with anxiogenic-like phenotype or without presentation of anxiety-associated behaviors (15). These conflicting results may be because the ages of animals used in these studies were different. Other studies have demonstrated that MGluR8 knockout mice show increased anxiety until age of 6 months (38,39). In addition, MGluR8 stimulation resulted in ameliorated anxiety in wild-type mice (40) and a further study showed that mGluR8 activation can not only decrease anxiety, but also reduce voluntary ethanol intake as well as associated acquisition of conditioned place preference (CPP) (41). In addition, previous studies have revealed that mGluR8 has anti-hyperalgesic effects mainly in CNS regions, such as the amygdala, periaqueductal gray (PAG), dorsal striatum, and nucleus tractus solitarius (NTS) (16,17); however, it also affects cutaneous nociceptors and the spine (10,13,33). MGluR8 has been known to be able to regulate TRPA1 activity on cutaneous nociceptors. Interestingly, DCPG was shown to relieve mechanical allodynia as well as thermal hyperalgesia 3 days after the induction of neuropathic pain but became ineffective 7 days later (16,20). We infer that mGluR8, which has anti-hyperalgesic and anti-anxiety effects, may also reduce VH in subjects with anxiety-related IBS. Our data showed that mGluR8 activation relieved CRD-induced VH, and antagonizing mGluR8 had the opposite effect. The effect of MSOP on visceral hypersensitivity is not so obvious as that of (s)-3,4-DCPG, because MSOP is not a selective antagonist of mGluR8. Anti-VH of mGluR8 is similar to that of mGluR7 (6). This is also consistent with the above study that agonists of mGluR8 have anti-hyperalgesic effects (16,20,42). To the best of our knowledge, this was the first study to identify and explore the anti-hyperalgesia effect of mGluR8 in the intestine with VH models.
A recent study suggests that persistent mild inflammation can result in VH under the circumstances of IBS. Compared to healthy individuals, IBS patients have increased risk for mucosal hyperplasia and often present with inflammatory cell infiltration and aggregation, indicating the occurrence persistent mild inflammation (43). In our study, we found that NMS rats were associated with increased level of MPO which was decreased after receiving (S)-3,4-DCPG. Furthermore, it has been well studied that imbalance of Th1/Th2 is characterized in IBS patients with diarrhea (D-IBS). Th1 cells mediate the expressions of cytokines such as IL-1β and TNF-α, which all promote the inflammatory response, whereas Th2 mediated the expressions of anti-inflammatory cytokines such as IL-10 (44). It has been shown that Th1 cells can activate immune cells and promote the release of pro-inflammatory cytokines which affects intestinal neurons and smooth muscle cells, thereby increasing the sensitivity of chemoreceptors and mechanoreceptors of the intestinal mucosa (45). Accumulating evidence suggests that TNF-α and IL-6 play important roles in IBS (46-49).
Elevated secretion of pro-inflammatory cytokines has been known to participate in the pathogenesis of VH in neonatal colonic irritation model (50). Another study showed that Bifco can elevate the level of short-chain fatty acids (SCFAs) and decrease secretion of IL-6 and TNF-α (26). In the present study, decreased TNF-α mRNA expression and MPO activity were found in the intestinal mucosa of rats treated with (S)-3,4-DCPG. This study showed that activating mGluR8 decreased the TNF-α expression in the colon and ameliorated inflammation, and that the effect of mGluR8 inhibition is opposite, supporting the conclusion from the opposite side. These results further confirm the findings of the previous studies.
It has been known that NF-κB-dependent inflammatory mediators such as IL-6 play important roles in the establishment of a NMS model of IBS (51). In addition, elevated secretion of cytokines such as IL-1β and TNF-α could result in VH through activating the TLR4/MD88/NF-κB signaling pathway in the spinal cord (50). However, in our study, the TLR4 and NF-κB protein expression did not statistically change and it is worthwhile to try other inflammatory signaling pathways such as ERK, JAK-STAT. These findings indicate that activating mGluR8 may inhibit the expression of TNF-α to relieve inflammation which result in further alleviation of VH.
MGluR8 is an important excitatory modulator related to several pathological diseases of the nervous system, and subjects with IBS exhibit abnormalities of the brain-gut axis. However, we did not further explore mGluR8 expression in CNS and conduction system (spinal cord) in this VH rat model and did not investigate the role and mechanism of glutamate transporters that participate in glutamate excitation transmission in the central and peripheral nervous systems. These are some limitations of this study.
Conclusions
In summary, we demonstrated that mGluR8 may participate in the pathogenesis of VH in IBS through inhibiting the production of TNF-α.
Acknowledgments
Funding: This work was funded by Science and Technology Hall of Zhejiang Province, China (No. LGD19H030002).
Footnote
Reporting Checklist: The authors have completed the ARRIVE reporting checklist. Available at https://atm.amegroups.com/article/view/10.21037/atm-22-6452/rc
Data Sharing Statement: Available at https://atm.amegroups.com/article/view/10.21037/atm-22-6452/dss
Conflicts of Interest: All authors have completed the ICMJE uniform disclosure form (available at https://atm.amegroups.com/article/view/10.21037/atm-22-6452/coif). The authors have no conflicts of interest to declare.
Ethical Statement: The authors are accountable for all aspects of the work in ensuring that questions related to the accuracy or integrity of any part of the work are appropriately investigated and resolved. Animal experiments were performed in the Hangzhou Hibio Technology Co., Ltd. under a project license (No. HB2007020) granted by the Hangzhou Hibio Technology Co., Ltd. in compliance with Hangzhou Hibio Technology Co., Ltd. guidelines for the care and use of animals.
Open Access Statement: This is an Open Access article distributed in accordance with the Creative Commons Attribution-NonCommercial-NoDerivs 4.0 International License (CC BY-NC-ND 4.0), which permits the non-commercial replication and distribution of the article with the strict proviso that no changes or edits are made and the original work is properly cited (including links to both the formal publication through the relevant DOI and the license). See: https://creativecommons.org/licenses/by-nc-nd/4.0/.
References
- Zhao K, Yu L, Wang X, et al. Clostridium butyricum regulates visceral hypersensitivity of irritable bowel syndrome by inhibiting colonic mucous low grade inflammation through its action on NLRP6. Acta Biochim Biophys Sin (Shanghai) 2018;50:216-23. [Crossref] [PubMed]
- Barbara G, Stanghellini V, De Giorgio R, et al. Activated mast cells in proximity to colonic nerves correlate with abdominal pain in irritable bowel syndrome. Gastroenterology 2004;126:693-702. [Crossref] [PubMed]
- Park JH, Rhee PL, Kim HS, et al. Mucosal mast cell counts correlate with visceral hypersensitivity in patients with diarrhea predominant irritable bowel syndrome. J Gastroenterol Hepatol 2006;21:71-8. [Crossref] [PubMed]
- Tang HY, Jiang AJ, Wang XY, et al. Uncovering the pathophysiology of irritable bowel syndrome by exploring the gut-brain axis: a narrative review. Ann Transl Med 2021;9:1187. [Crossref] [PubMed]
- Shukla R, Ghoshal U, Ranjan P, et al. Expression of Toll-like Receptors, Pro-, and Anti-inflammatory Cytokines in Relation to Gut Microbiota in Irritable Bowel Syndrome: The Evidence for Its Micro-organic Basis. J Neurogastroenterol Motil 2018;24:628-42. [Crossref] [PubMed]
- Shao L, Liu Y, Xiao J, et al. Activating metabotropic glutamate receptor-7 attenuates visceral hypersensitivity in neonatal maternally separated rats. Int J Mol Med 2019;43:761-70. [Crossref] [PubMed]
- Eijkelkamp N, Heijnen CJ, Carbajal AG, et al. G protein-coupled receptor kinase 6 acts as a critical regulator of cytokine-induced hyperalgesia by promoting phosphatidylinositol 3-kinase and inhibiting p38 signaling. Mol Med 2012;18:556-64. [Crossref] [PubMed]
- Nozu T, Miyagishi S, Ishioh M, et al. Phlorizin attenuates visceral hypersensitivity and colonic hyperpermeability in a rat model of irritable bowel syndrome. Biomed Pharmacother 2021;139:111649. [Crossref] [PubMed]
- Farzaei MH, Bahramsoltani R, Abdollahi M, et al. The Role of Visceral Hypersensitivity in Irritable Bowel Syndrome: Pharmacological Targets and Novel Treatments. J Neurogastroenterol Motil 2016;22:558-74. [Crossref] [PubMed]
- Ren TH, Wu J, Yew D, et al. Effects of neonatal maternal separation on neurochemical and sensory response to colonic distension in a rat model of irritable bowel syndrome. Am J Physiol Gastrointest Liver Physiol 2007;292:G849-56. [Crossref] [PubMed]
- Ji NN, Xia M. Enriched environment alleviates adolescent visceral pain, anxiety- and depression-like behaviors induced by neonatal maternal separation. Transl Pediatr 2022;11:1398-1407. [Crossref] [PubMed]
- Tang Y, Chen L, Liu B, et al. Spinal P2X4 Receptors Involved in Visceral Hypersensitivity of Neonatal Maternal Separation Rats. Purinergic Signal 2022; Epub ahead of print. [Crossref]
- Lee KS, Park EH, Cho HY, et al. Peripheral group II and III metabotropic glutamate receptors in the knee joint attenuate carrageenan-induced nociceptive behavior in rats. Neurosci Lett 2013;542:21-5. [Crossref] [PubMed]
- Dogra S, Conn PJ. Targeting metabotropic glutamate receptors for the treatment of depression and other stress-related disorders. Neuropharmacology 2021;196:108687. [Crossref] [PubMed]
- Boccella S, Marabese I, Guida F, et al. The Modulation of Pain by Metabotropic Glutamate Receptors 7 and 8 in the Dorsal Striatum. Curr Neuropharmacol 2020;18:34-50. [Crossref] [PubMed]
- Palazzo E, Marabese I, Luongo L, et al. Nociception modulation by supraspinal group III metabotropic glutamate receptors. J Neurochem 2017;141:507-19. [Crossref] [PubMed]
- Khan A, Khan S, Kim YS. Insight into Pain Modulation: Nociceptors Sensitization and Therapeutic Targets. Curr Drug Targets 2019;20:775-88. [Crossref] [PubMed]
- Govea RM, Zhou S, Carlton SM. Group III mGluR8 negatively modulates TRPA1. Neuroscience 2016;334:134-47. [Crossref] [PubMed]
- Sharkey KA, Mawe GM. The enteric nervous system. Physiol Rev 2022; [Crossref]
- Tong Q, Kirchgessner AL. Localization and function of metabotropic glutamate receptor 8 in the enteric nervous system. Am J Physiol Gastrointest Liver Physiol 2003;285:G992-G1003. [Crossref] [PubMed]
- Palazzo E, Fu Y, Ji G, et al. Group III mGluR7 and mGluR8 in the amygdala differentially modulate nocifensive and affective pain behaviors. Neuropharmacology 2008;55:537-45. [Crossref] [PubMed]
- Marabese I, de Novellis V, Palazzo E, et al. Effects of (S)-3,4-DCPG, an mGlu8 receptor agonist, on inflammatory and neuropathic pain in mice. Neuropharmacology. 2007;52:253-62. [Crossref] [PubMed]
- Lucarini E, Seguella L, Vincenzi M, et al. Role of Enteric Glia as Bridging Element between Gut Inflammation and Visceral Pain Consolidation during Acute Colitis in Rats. Biomedicines 2021;9:1671. [Crossref] [PubMed]
- Ohman L, Simrén M. Pathogenesis of IBS: role of inflammation, immunity and neuroimmune interactions. Nat Rev Gastroenterol Hepatol 2010;7:163-73. [Crossref] [PubMed]
- Shao YY, Guo Y, Feng XJ, et al. Oridonin Attenuates TNBS-induced Post-inflammatory Irritable Bowel Syndrome via PXR/NF-κB Signaling. Inflammation 2021;44:645-58. [Crossref] [PubMed]
- Jalanka J, Lam C, Bennett A, et al. Colonic Gene Expression and Fecal Microbiota in Diarrhea-predominant Irritable Bowel Syndrome: Increased Toll-like Receptor 4 but Minimal Inflammation and no Response to Mesalazine. J Neurogastroenterol Motil 2021;27:279-91. [Crossref] [PubMed]
- Zhou Y, Zhang F, Mao L, et al. Bifico relieves irritable bowel syndrome by regulating gut microbiota dysbiosis and inflammatory cytokines. Eur J Nutr 2022; Epub ahead of print. [Crossref]
- Li L, Xie R, Hu S, et al. Upregulation of cystathionine beta-synthetase expression by nuclear factor-kappa B activation contributes to visceral hypersensitivity in adult rats with neonatal maternal deprivation. Mol Pain 2012;8:89. [Crossref] [PubMed]
- Matsumoto K, Takagi K, Kato A, et al. Role of transient receptor potential melastatin 2 (TRPM2) channels in visceral nociception and hypersensitivity. Exp Neurol 2016;285:41-50. [Crossref] [PubMed]
- Cavas M, Scesa G, Martín-López M, et al. Selective agonism of mGlu8 receptors by (S)-3,4-dicarboxyphenylglycine does not affect sleep stages in the rat. Pharmacol Rep 2017;69:97-104. [Crossref] [PubMed]
- Joo YE. Alteration of fecal microbiota in patients with postinfectious irritable bowel syndrome. J Neurogastroenterol Motil 2015;21:135-7. [Crossref] [PubMed]
- Kim S, Keum B, Byun J, et al. Colonic Mucosal Immune Activation in Mice with Ovalbumin-Induced Allergic Airway Disease: Association between Allergic Airway Disease and Irritable Bowel Syndrome. Int J Mol Sci 2021;23:181. [Crossref] [PubMed]
- Bao CH, Wang CY, Li GN, et al. Effect of mild moxibustion on intestinal microbiota and NLRP6 inflammasome signaling in rats with post-inflammatory irritable bowel syndrome. World J Gastroenterol 2019;25:4696-714. [Crossref] [PubMed]
- Ye Y, Wang P, Zhou F. miR-489-3p inhibits TLR4/NF-κB signaling to prevent inflammation in psoriasis. Exp Ther Med 2021;22:744. [Crossref] [PubMed]
- Bao Z, Zhang S, Li X. MiR-5787 Attenuates Macrophages-Mediated Inflammation by Targeting TLR4/NF-κB in Ischemic Cerebral Infarction. Neuromolecular Med 2021;23:363-70. [Crossref] [PubMed]
- Fernández-Montoya J, Avendaño C, Negredo P. The Glutamatergic System in Primary Somatosensory Neurons and Its Involvement in Sensory Input-Dependent Plasticity. Int J Mol Sci. 2017;19:69. [Crossref] [PubMed]
- Julio-Pieper M, O’Connor RM, Dinan TG, et al. Regulation of the brain-gut axis by group III metabotropic glutamate receptors. Eur J Pharmacol 2013;698:19-30. [Crossref] [PubMed]
- Linden AM, Johnson BG, Peters SC, et al. Increased anxiety-related behavior in mice deficient for metabotropic glutamate 8 (mGlu8) receptor. Neuropharmacology 2002;43:251-9. [Crossref] [PubMed]
- Duvoisin RM, Zhang C, Pfankuch TF, et al. Increased measures of anxiety and weight gain in mice lacking the group III metabotropic glutamate receptor mGluR8. Eur J Neurosci 2005;22:425-36. [Crossref] [PubMed]
- Duvoisin RM, Pfankuch T, Wilson JM, et al. Acute pharmacological modulation of mGluR8 reduces measures of anxiety. Behav Brain Res 2010;212:168-73. [Crossref] [PubMed]
- Bahi A. Decreased anxiety, voluntary ethanol intake and ethanol-induced CPP acquisition following activation of the metabotropic glutamate receptor 8 "mGluR8". Pharmacol Biochem Behav 2017;155:32-42. [Crossref] [PubMed]
- Chae JW, Kang DH, Li Y, et al. Antinociceptive effects of nefopam modulating serotonergic, adrenergic, and glutamatergic neurotransmission in the spinal cord. Neurosci Lett 2020;731:135057. [Crossref] [PubMed]
- Oświęcimska J, Szymlak A, Roczniak W, et al. New insights into the pathogenesis and treatment of irritable bowel syndrome. Adv Med Sci 2017;62:17-30. [Crossref] [PubMed]
- Tao X, Liu H, Xia J, et al. Processed product (Pinelliae Rhizoma Praeparatum) of Pinellia ternata (Thunb.) Breit. Alleviates the allergic airway inflammation of cold phlegm via regulation of PKC/EGFR/MAPK/PI3K-AKT signaling pathway. J Ethnopharmacol 2022;295:115449. [Crossref] [PubMed]
- Collins SM, Piche T, Rampal P. The putative role of inflammation in the irritable bowel syndrome. Gut 2001;49:743-5. [Crossref] [PubMed]
- Li Y, Tian X, Li S, et al. Total polysaccharides of adlay bran (Coix lachryma-jobi L.) improve TNF-α induced epithelial barrier dysfunction in Caco-2 cells via inhibition of the inflammatory response. Food Funct 2019;10:2906-13. [Crossref] [PubMed]
- Scully P, McKernan DP, Keohane J, et al. Plasma cytokine profiles in females with irritable bowel syndrome and extra-intestinal co-morbidity. Am J Gastroenterol 2010;105:2235-43. [Crossref] [PubMed]
- O'Malley D, Dinan TG, Cryan JF. Altered expression and secretion of colonic interleukin-6 in a stress-sensitive animal model of brain-gut axis dysfunction. J Neuroimmunol 2011;235:48-55. [Crossref] [PubMed]
- O'Malley D, Dinan TG, Cryan JF. Interleukin-6 modulates colonic transepithelial ion transport in the stress-sensitive wistar kyoto rat. Front Pharmacol 2012;3:190. [Crossref] [PubMed]
- Chen ZY, Zhang XW, Yu L, et al. Spinal toll-like receptor 4-mediated signalling pathway contributes to visceral hypersensitivity induced by neonatal colonic irritation in rats. Eur J Pain 2015;19:176-86. [Crossref] [PubMed]
- O'Malley D, Liston M, Hyland NP, et al. Colonic soluble mediators from the maternal separation model of irritable bowel syndrome activate submucosal neurons via an interleukin-6-dependent mechanism. Am J Physiol Gastrointest Liver Physiol 2011;300:G241-52. [Crossref] [PubMed]
(English Language Editor: J. Jones)