The mitosis-related gene OIP5 is a potential biomarker in pan-cancer
Highlight box
Key findings
• OIP5 plays a role in promoting cancer in many types of tumors.
What is known and what is new?
• OIP5 is a key molecule in mitosis and is increased in testis and different cancers.
• OIP5 may promote tumor progression by affecting the tumor immune microenvironment and genomic stability.
What is the implication, and what should change now?
• OIP5 can serve as a potential candidate factor to predict prognosis and guide the use of therapeutics in cancer.
Introduction
Malignant tumors are major causes of mortality globally, and research to uncover the related molecular mechanisms has been conducted worldwide (1). Cancers have a genetic component with a common complex molecular process; therefore, exploration of the homogeneity of a particular gene across various tumors is crucial to discovering new clinical therapeutic targets and prognostic evaluation signs (2). Recently, immune checkpoint blockade therapies have been developed, and through the use of public databases such as The Cancer Genome Atlas (TCGA), scientists have continued to improve and search for new immunotherapeutic targets by analyzing pan-oncogene levels and assessing their relationship with prognostic outcomes and related signaling pathways (3,4). Therefore, it is necessary to analyze pan-cancer gene expression levels, evaluate their relationship with prognostic outcomes, and understand their molecular mechanism to identify novel therapeutics.
OIP5 is located on human chromosome 15 and encodes a 25-kDa protein with one coiled-coil domain. It was identified through a yeast two-hybrid test and was found to interact with Opa (Neisseria gonorrhoeae opacity-associated) proteins (5). OIP5 is found at the centromere and plays an important role in recruiting centromere protein-A (CENP-A) through interacting with the Holliday junction recognition protein (6). CENP-A, which contributes to chromosome fragility in human cancer, is overexpressed and localized abnormally in many kinds of cancers (7). Increased expression of OIP5 also has been reported in testis as well as a diverse range of cancers, such as glioblastoma, bladder cancer (BLCA), oral cancer, and lung cancer, and it has been associated with different tumor biological events (8-11). As a result, OIP5 is considered to be a cancer-testis specific gene, but its function in tumor development remains unclear (12). Therefore, a pan-cancer analysis is required to investigate its potential function as a novel target for tumor treatment.
In this study, we analyzed OIP5 expression levels within a diverse range of cancers and their association with prognosis using data from the Genotype-Tissue Expression (GTEx) project, TCGA, and the Cancer Cell Line Encyclopedia (CCLE). Further, to understand the impact of OIP5 on the immune microenvironment and malignant progression of tumors, we examined the relationships of OIP5 expression with immunomodulation, tumor-infiltrating immune cells (TIICs), microsatellite instability (MSI), tumor mutational burden (TMB), mRNA expression-based stemness score (RNAss), and DNA methylation-based stemness score (DNAss) in various cancers. To investigate the role of OIP5 in cancer in more detail, we also carried out gene set enrichment analysis (GSEA). We present the following article in accordance with the REMARK reporting checklist (available at https://atm.amegroups.com/article/view/10.21037/atm-22-6640/rc).
Methods
Data acquisition
Data on OIP5 expression levels in diverse cancers and matched healthy samples were acquired from the GTEx (https://commonfund.nih.gov/GTEx/), CCLE (https://portals.broadinstitute.org), and TCGA (http://cancergenome.nih.gov/) databases (13). The Tumor IMmune Estimation Resource dataset (TIMER, http://timer.cistrome.org/) was used for comprehensive assessment of the abundance of TIICs (14). Gene somatic mutation data were also downloaded for analysis. The study was conducted in accordance with the Declaration of Helsinki (as revised in 2013).
Gene expression levels
The expression levels of OIP5 in 31 healthy samples and 21 diverse tumor samples were compared using the Kruskal-Wallis test. After that, using TCGA data, OIP5 expression in tumor tissues was compared with that in corresponding healthy tissues. Due to the insufficient number of healthy noncancerous samples available from the TCGA database, healthy control sample data were also collected from the GTEx database.
Survival prognosis analysis
Clinical phenotypic and survival information of TCGA cases was extracted. Univariate regression was used to assess the relationships of OIP5 with the following four prognostic indicators in different tumors: overall survival (OS), disease-specific survival (DSS), disease-free survival (DFS), and progression-free survival (PFS). Cases were classified into the high- or low-expression group based on the median OIP5 level. The Kaplan-Meier method and log-rank test were used for the survival analysis of each cancer.
Analysis of the effect of OIP5 on TIICs and the tumor microenvironment
The effect of OIP5 on TIIC infiltration levels in diverse cancers was assessed using Spearman’s rank correlation coefficient. The TIICs of focus mainly included CD4+ T cells, CD8+ T cells, B cells, dendritic cells, macrophages, and neutrophils. The Estimation of STromal and Immune cells in MAlignant Tumors using Expression data (ESTIMATE) algorithm was used to determine the immune, stromal, and ESTIMATE scores of different samples, and the relationships between OIP5 expression levels with these scores were also assessed (15).
Analysis of the correlations of OIP5 expression with immunomodulation, immune neoantigens, and gene mutations
The correlations of OIP5 expression level with 150 common immune-related molecules, including the major histocompatibility complex, chemokines, chemokine receptors, immune stimulator proteins, and immune inhibitors, were analyzed using Spearman's rank correlation coefficient. Similarly, the relationship between OIP5 expression level and the number of neoantigens was evaluated for each tumor type. The gene mutation information of 514 cases with lung adenocarcinoma (LUAD) was retrieved from the TCGA database; the samples were classified and compared based on OIP5 expression, and the role of OIP5 in cell genome stability was evaluated.
Analysis of the correlations of OIP5 expression with DNAss, RNAss, TMB, and MSI
The correlations of OIP5 expression level with the DNAss, RNAss, TMB, and MSI were examined in different tumors by calculating the Pearson’s correlation coefficient.
GSEA
To identify significantly different pathways in the high and low OIP5 expression groups, GSEA was performed. The Kyoto Encyclopedia of Genes and Genomes (https://www.kegg.jp.) database was used for analysis. The criteria for significance were set at normalized enrichment score (NES) >1.5, false discovery rate (FDR) <0.25, and P value <0.05.
Statistical analysis
The R software (version 4.0.2; https://www.R-project.org) was used for data analysis, and plots were obtained using various R packages, including limma (16), clusterprofiler (17), survival (18), and ggplot2 (19). P value <0.05 was considered to be statistically significant.
Results
OIP5 showed up-regulation in various malignancies
Levels of OIP5 expression were analyzed in cancer and healthy samples from the GTEx, TCGA, and CCLE databases. In the GTEx dataset analysis, OIP5 expression was found in 31 healthy samples, with high expression in the testis and bone marrow and low expression in the heart and pancreas (Figure 1A). Based on CCLE data, OIP5 expression was highest in hematopoietic and lymphoid tissue and lowest in the kidney (Figure 1B). OIP5 expression levels were analyzed in samples and matched non-carcinoma samples derived from the TCGA (Figure 1C) in combination with normal tissue data from the GTEx project (Figure 1D). Compared to normal tissues, all solid tumors showed increased expression of OIP5 except for pheochromocytoma and paraganglioma and kidney chromophobe (KICH).
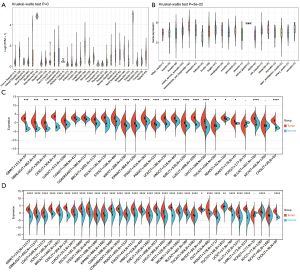
Prognostic significance of OIP5 in pan-cancer
To evaluate the prognostic role of OIP5 in different cancers, we next conducted univariate Cox regression. Results showed that OIP5 expression predicted poor OS in adrenocortical carcinoma (ACC), LUAD, the pan-kidney cohort [KICH, kidney renal clear cell carcinoma (KIRC), and kidney renal papillary cell carcinoma (KIRP)], glioblastoma multiforme low-grade glioma (LGG), and many other cancers (Figure 2A). Similar results were observed for DSS (Figure 2B), DFS (Figure 2C), and PFS (Figure 2D).
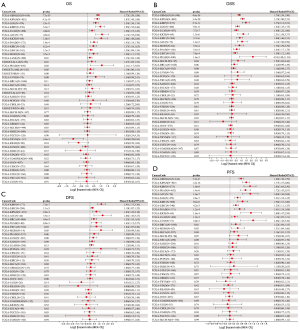
Further, Kaplan-Meier curves were plotted to analyze OS, DSS, DFS, and PFS in patients with cancer based on OIP5 expression. Increased expression of OIP5 was found to predict dismal OS in ACC, BLCA, KICH, KIRC, KIRP, LGG, liver hepatocellular carcinoma (LIHC), LUAD, mesothelioma (MESO), pancreatic adenocarcinoma (PAAD), prostate adenocarcinoma (PRAD), thymoma (THYM), and uveal melanoma (UVM). Interestingly, in contrast, OIP5 expression was associated with longer OS in THYM (Figure 3). To eliminate the effect of death from non-cancer causes on the statistical results, DSS was used as the end event. The results confirmed that increased OIP5 expression had similar prognostic significance for DSS as it did for OS in all tumors, except THYM (Figure 4). Increased expression of OIP5 predicted dismal prognostic outcomes in ACC, breast cancer (BRCA), KIRP, LIHC, PAAD, and PRAD, as revealed by DFS analysis (Figure 5). Results also showed that OIP5 expression was similarly correlated with PFS (Figure 6). See Table S1 for a full list of abbreviations used.
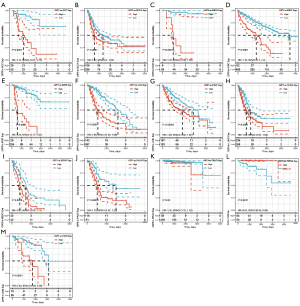
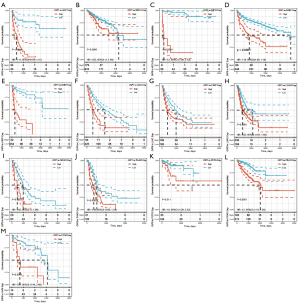
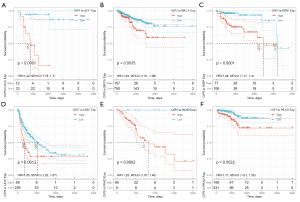
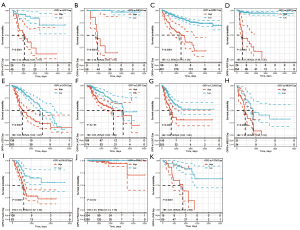
Effects of OIP5 on tumor immune infiltration and the tumor immune microenvironment
The effect of OIP5 expression on TIIC recruitment was investigated through analysis of the relationship of OIP5 gene expression levels with the numbers of CD4+ T cells, CD8+ T cells, B cells, dendritic cells, neutrophils, and macrophages in different tumors. OIP5 expression was negatively related to TIIC levels in LUAD, lung squamous cell carcinoma (LUSC), esophageal carcinoma, and stomach and esophageal carcinoma. However, a positive relationship was found between TIIC levels and increased expression of OIP5 in THYM, thyroid carcinoma, KIRC, and LGG (Figure 7A).
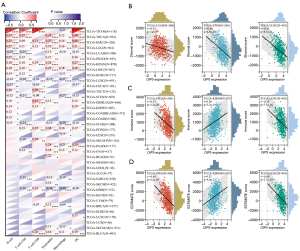
We also analyzed the effect of OIP5 on the tumor microenvironment by assessing the relationships of OIP5 gene expression level with the stromal score, immune score, and ESTIMATE score. OIP5 expression showed negative relationships with stromal score (Figure 7B), immune score (Figure 7C), and ESTIMATE score (Figure 7D) in LUAD, stomach and esophageal carcinoma, stomach adenocarcinoma (STAD), and LUSC. These results suggested that OIP5 might inhibit TIIC infiltration in the tumor microenvironment.
Correlation analysis of OIP5 expression levels with immune regulation, neoantigens, and gene mutation
Based on the effect of OIP5 expression on the immune microenvironment, we further examined the association between OIP5 expression and 150 common immunomodulatory molecules in 40 different tumors. Surprisingly, OIP5 showed a negative correlation with the levels of most immunomodulators in LUAD, LUSC, and STAD but showed a positive correlation with immunomodulator levels in thyroid carcinoma, the pan-kidney cohort, breast cancer, ovarian cancer, and other tumors (Figure 8A). This result suggested that OIP5 possibly affects TIIC levels by regulating the specific immune response.
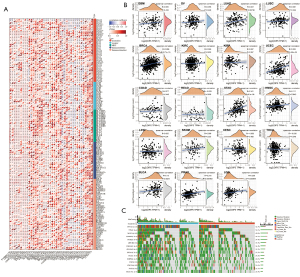
Additionally, we also analyzed the association between the number of neoantigens and OIP5 expression in each cancer. OIP5 expression showed a positive correlation with neoantigen number in LUAD, uterine corpus endometrial carcinoma, breast cancer, STAD, low-grade glioma, and prostate adenocarcinoma (Figure 8B). These findings suggested that OIP5 possibly enhances neoantigen generation in cancer cells, which was further confirmed by gene mutation analysis. In LUAD, more gene mutations were observed in the OIP5 high-expression group than in the low-expression group. In particular, TP53, which is a well-known tumor suppressor gene, had a remarkably increased mutation rate (Figure 8C).
Association of OIP5 expression level with DNAss, RNAss, TMB, and MSI
The DNAss and RNAss of tumor cells have been reported to be tightly associated with tumor progression, metastasis, and drug resistance (20). MSI and TMB are indicators of tumor cell genomic stability (21).
In this study, OIP5 expression showed a positive relationship with both the DNAss (Figure 9A) and RNAss (Figure 9B) in many tumors, including LUAD, LUSC, STAD, and testicular germ cell tumors. The OIP5 expression level also showed a positive correlation with TMB in many tumors, such as LUAD, STAD, and KICH, but a negative correlation with THYM, ovarian cancer, and thyroid carcinoma (Figure 9C). Moreover, the expression of OIP5 was positively correlated with MSI in STAD, cholangiocarcinoma, and stomach and esophageal carcinoma but was negatively correlated with MSI in glioblastoma multiforme low-grade glioma and testicular germ cell tumors (Figure 9D).
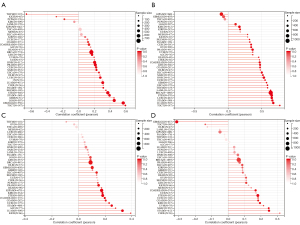
GSEA
The protein-protein interaction network of OIP5 was generated to understand the function and potential mechanism of OIP5 in cells. Surprisingly, the results showed that OIP5 was linked to CENP-A, CENP-M, CENPN, CENP-U, MIS18A, MIS18BP1, HJURP, NCAPG, NUSAP1, and CDCA8, which are all mitosis-related proteins (Figure 10A). Furthermore, to evaluate the possible biological involvement of OIP5 using GSEA analysis, data in The Kyoto Encyclopedia of Genes and Genomes were classified into high or low OIP5 expression groups based on the median OIP5 expression. Surprisingly, OIP5 showed enrichment in the cell cycle, base excision repair, homologous recombination, DNA replication, the p53 signaling pathway, and mismatch repair pathways (Figure 10B).
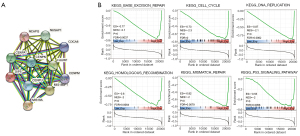
Discussion
In recent years, targeted therapy has brought patients with cancer hope for a cure; however, the lack of effective targets has hindered the optimization of this anticancer therapy. Increasing evidence of the crucial effects of cancer-testis specific genes on tumor development has provided some new potential targets for cancer therapy (12,22). OIP5, a cancer-testis specific gene, plays an important role in centromere protein A (CENPA) recruitment to the centromere during mitosis (23,24). It also affects chromatin organization and controls the cell cycle. The mitotic rate of a cancer cell represents a vital manifestation of its proliferation ability, proliferation ability often predicts dismal prognostic outcomes. LncRNA OIP5-AS1 (Opa-InteractionProtein5 antisense RNA1) can bind to and negatively regulate the activity of multiple cellular RNAs and microRNAs, including cyclin G associated kinase and ELAV like RNA binding protein 1. Overexpression of OIP5-AS1 can inhibit the survival, colony formation, invasion and migration of multiple myeloma cells in vitro, induce cell cycle arrest in G1 phase, induce apoptosis, and inhibit tumor formation in vivo (25). Therefore, in this study, a pan-cancer analysis of OIP5 expression was performed to evaluate its role in prognosis, immunity, and metabolism, as well as its therapeutic potential.
First, we analyzed the differential OIP5 expression in tumor cells and healthy samples. In normal tissues, OIP5 was most significantly up-regulated in the bone marrow and testis, whereas it was down-regulated in the heart and pancreas. We found that the expression level of OIP5 was correlated with the proliferative activity of the tissue. This result is consistent with the reported role of OIP5 in the mitotic process and its effect on the cell cycle. Based on the analysis of the differential expression of OIP5 in tumor and paired healthy samples, OIP5 expression was found to be increased in all tumors except kidney chromophobe and pheochromocytoma and paraganglioma. These findings corroborate those of previous studies and the reported characteristics of OIP5 as a cancer-testis specific gene (8,26,27). Examination of the effect of OIP5 expression level on OS, dss, dfs, and pfs in various cancers showed that OIP5 up-regulation predicted a dismal prognostic outcome in multiple cancers. These results highlight the potential of OIP5 to become a new prognostic biomarker of pan-cancer.
Of note, in THYM, increased expression of OIP5 predicted a better prognosis and was also associated with the promotion of immune cell infiltration. The immune system plays an important role in tumor genesis and progression, and tumor occurrence is often associated with immune system dysfunction (28). OIP5 might inhibit TIIC infiltration in the tumor microenvironment. OIP5-AS1 level was highly expressed in Cancer-associated fibroblasts, which are the most important stromal cells in the tumor microenvironment, and exosomes derived from cancer-associated fibroblasts promote lung cancer progression through the OIP5-AS1/miR-142-5p/PD-L1 axis (29). Linc-OIP5 in breast cancer cells regulates angiogenesis of human umbilical vein endothelial cells through YAP1/Notch/NRP1 signal circuit in tumor microenvironment (30). Various immune cells, including dendritic cells, macrophages, neutrophils, B cells, and T cells, can infiltrate the tumor microenvironment, which affects tumor development. Our results showed an inconsistent association of OIP5 expression levels with TIIC levels in different tumors, which may be attributable to the complexity of the TIIC infiltration process. Surprisingly, TIIC levels were positively related to OIP5 expression in THYM, and prognosis showed a similar trend, whereas the opposite trend was observed in LUAD and glioblastoma. Further research on the association of OIP5 with tumor immune and stromal scores showed that OIP5 impacted immune cell infiltration and had significant effects on stromal cell infiltration, which can affect the purity and prognosis of tumors (15,31). Although the influence of OIP5 on immune cell infiltration was inconsistent in different tumors, most of the correlations were statistically significant. This result highlights that OIP5 predicted a dismal prognostic outcome, and the mechanism of it worth investigating.
Extensive research on immune checkpoints, and the advent of immune checkpoint inhibitors (ICIs), has drawn increasing interest in the roles of immune regulation and neoantigen formation in tumor genesis and progression (32). Despite the fact that few diseases can be treated with ICIs at present, the effects of OIP5 on tumor immune modulation and neoantigen development must be analyzed (33). In this study, association analysis of OIP5 expression with 150 immune regulatory molecules in different cancers revealed a similar trend to that observed for immune cell infiltration, which was negatively correlated with OIP5 expression in LUAD, LUSC, and STAD but positively correlated in thyroid carcinoma, the pan-kidney cohort, and ovarian cancer. These results show a consistent and stable effect of OIP5 on immune regulation in different tumors. Further examination of the relationships of OIP5 expression level with neoantigen formation and gene mutation frequency in tumors showed positive correlations, further proving that OIP5 could potentially serve as an antitumor therapeutic target.
The level of tumor differentiation is closely related to cancer stem cell viability, which is reflected by the RNAss and DNAss, with a higher stemness score indicating a lower degree of tumor differentiation (20). Research has proven that TMB and MSI are important indicators for the efficacy of ICI treatment in patients with cancer (34,35). In this study, we examined the relationships of OIP5 expression with these four indexes to explore how OIP5 might affect tumor differentiation and immunotherapy sensitivity. In many cancers, the OIP5 expression level was related to low differentiation and high TMB and MSI. Interestingly, OIP5 expression in THYM showed a positive correlation with tumor differentiation, which explains its positive prognostic effect. A low differentiation level of tumor cells is always associated with a poor prognosis; however, higher TMB and MSI mean that increased neoantigen production by the tumor can be easily recognized by immune cells, thus improving the therapeutic efficacy of ICIs (36,37). Previous studies have shown that the benefits of ICI therapy are significantly increased for cases with a high TMB compared to those with a low TMB (38-40). Currently, OIP5 is known to be related to poor survival in diverse cancers; however, with the increasing application of ICIs in tumor treatment, it might also become a positive indicator to predict the therapeutic effect and be related to a positive prognostic outcome. This study serves additional evidence that OIP5 could serve as a marker for cancer diagnosis and treatment.
To understand the pathophysiological mechanism underlying the role of OIP5 in pan-cancer, a GSEA was carried out to obtain the functional network and related signal pathways of OIP5. Through functional network and signal pathway analysis, OIP5 was found to be an important player in cell mitosis and to be enriched in the cell cycle, base excision repair, homologous recombination, DNA replication, the p53 signaling pathway, and mismatch repair pathways. These results suggest that OIP5, as a key CENP-A recruitment molecule, may ultimately affect tumor genesis and progression by influencing the precise distribution of DNA during the mitotic process (41,42).
Conclusions
In summary, our research demonstrates that OIP5, as a cancer-testis specific gene, exhibits high expression and predicts a dismal prognostic outcome in many common cancers. Its expression might influence patient prognosis by affecting the tumor microenvironment and genomic stability. Therefore, OIP5 has the potential to serve as a therapeutic target and prognostic evaluation index for multiple cancers. The main limitation of our study was its lack of in vivo and in vitro experiments to prove the findings of our bioinformatic analyses performed on openly accessible databases. Therefore, it is necessary to conduct follow-up validation experiments in the future.
Acknowledgments
Funding: This work was supported by the National Natural Science Foundation of China (82173252, 81871866), the Shaanxi Special Support Plan-Program for Leading Talents of Science and Technology Innovation (No. 2019 Special Support Plan), the Shaanxi Social Development Science and Technology Key Project (2022SF-145), Military High-level Scientific and Technological Innovation Youth Talent Project (No. [2022]151) and Key R & D plan of Xuzhou City [KC22259].
Footnote
Reporting Checklist: The authors have completed the REMARK reporting checklist. Available at https://atm.amegroups.com/article/view/10.21037/atm-22-6640/rc
Conflicts of Interest: All authors have completed the ICMJE uniform disclosure form (available at https://atm.amegroups.com/article/view/10.21037/atm-22-6640/coif). The authors have no conflicts of interest to declare.
Ethical Statement: The authors are accountable for all aspects of the work in ensuring that questions related to the accuracy or integrity of any part of the work are appropriately investigated and resolved. The study was conducted in accordance with the Declaration of Helsinki (as revised in 2013).
Open Access Statement: This is an Open Access article distributed in accordance with the Creative Commons Attribution-NonCommercial-NoDerivs 4.0 International License (CC BY-NC-ND 4.0), which permits the non-commercial replication and distribution of the article with the strict proviso that no changes or edits are made and the original work is properly cited (including links to both the formal publication through the relevant DOI and the license). See: https://creativecommons.org/licenses/by-nc-nd/4.0/.
References
- Siegel RL, Miller KD, Fuchs HE, et al. Cancer statistics, 2022. CA Cancer J Clin 2022;72:7-33. [Crossref] [PubMed]
- Pan-cancer analysis of whole genomes. Nature 2020;578:82-93. [Crossref] [PubMed]
- Ribas A, Wolchok JD. Cancer immunotherapy using checkpoint blockade. Science 2018;359:1350-5. [Crossref] [PubMed]
- Blum A, Wang P, Zenklusen JC. SnapShot: TCGA-Analyzed Tumors. Cell 2018;173:530. [Crossref] [PubMed]
- Williams JM, Chen GC, Zhu L, et al. Using the yeast two-hybrid system to identify human epithelial cell proteins that bind gonococcal Opa proteins: intracellular gonococci bind pyruvate kinase via their Opa proteins and require host pyruvate for growth. Mol Microbiol 1998;27:171-86. [Crossref] [PubMed]
- Fu H, Liu N, Dong Q, et al. SENP6-mediated M18BP1 deSUMOylation regulates CENP-A centromeric localization. Cell Res 2019;29:254-7. [Crossref] [PubMed]
- Das A, Iwata-Otsubo A, Destouni A, et al. Epigenetic, genetic and maternal effects enable stable centromere inheritance. Nat Cell Biol 2022;24:748-56. [Crossref] [PubMed]
- He J, Zhao Y, Zhao E, et al. Cancer-testis specific gene OIP5: a downstream gene of E2F1 that promotes tumorigenesis and metastasis in glioblastoma by stabilizing E2F1 signaling. Neuro Oncol 2018;20:1173-84. [Crossref] [PubMed]
- He X, Hou J, Ping J, et al. Opa interacting protein 5 acts as an oncogene in bladder cancer. J Cancer Res Clin Oncol 2017;143:2221-33. [Crossref] [PubMed]
- Koinuma J, Akiyama H, Fujita M, et al. Characterization of an Opa interacting protein 5 involved in lung and esophageal carcinogenesis. Cancer Sci 2012;103:577-86. [Crossref] [PubMed]
- Zhu M, Takano A, Tsevegjav B, et al. Characterization of Opa interacting protein 5 as a new biomarker and therapeutic target for oral cancer. Int J Oncol 2022;60:27. [Crossref] [PubMed]
- Whitehurst AW. Cause and consequence of cancer/testis antigen activation in cancer. Annu Rev Pharmacol Toxicol 2014;54:251-72. [Crossref] [PubMed]
- Barretina J, Caponigro G, Stransky N, et al. The Cancer Cell Line Encyclopedia enables predictive modelling of anticancer drug sensitivity. Nature 2012;483:603-7. [Crossref] [PubMed]
- Li T, Fan J, Wang B, et al. TIMER: A Web Server for Comprehensive Analysis of Tumor-Infiltrating Immune Cells. Cancer Res 2017;77:e108-10. [Crossref] [PubMed]
- Yoshihara K, Shahmoradgoli M, Martínez E, et al. Inferring tumour purity and stromal and immune cell admixture from expression data. Nat Commun 2013;4:2612. [Crossref] [PubMed]
- Ritchie ME, Phipson B, Wu D, et al. limma powers differential expression analyses for RNA-sequencing and microarray studies. Nucleic Acids Res 2015;43:e47. [Crossref] [PubMed]
- Yu G, Wang LG, Han Y, et al. clusterProfiler: an R package for comparing biological themes among gene clusters. OMICS 2012;16:284-7. [Crossref] [PubMed]
- Rizvi AA, Karaesmen E, Morgan M, et al. gwasurvivr: an R package for genome-wide survival analysis. Bioinformatics 2019;35:1968-70. [Crossref] [PubMed]
- Ito K, Murphy D. Application of ggplot2 to Pharmacometric Graphics. CPT Pharmacometrics Syst Pharmacol 2013;2:e79. [Crossref] [PubMed]
- Malta TM, Sokolov A, Gentles AJ, et al. Machine Learning Identifies Stemness Features Associated with Oncogenic Dedifferentiation. Cell 2018;173:338-354.e15. [Crossref] [PubMed]
- Yates LR, Campbell PJ. Evolution of the cancer genome. Nat Rev Genet 2012;13:795-806. [Crossref] [PubMed]
- Wang D, Chen Z, Lin F, et al. OIP5 Promotes Growth, Metastasis and Chemoresistance to Cisplatin in Bladder Cancer Cells. J Cancer 2018;9:4684-95. [Crossref] [PubMed]
- Fujita Y, Hayashi T, Kiyomitsu T, et al. Priming of centromere for CENP-A recruitment by human hMis18alpha, hMis18beta, and M18BP1. Dev Cell 2007;12:17-30. [Crossref] [PubMed]
- Black BE, Bassett EA. The histone variant CENP-A and centromere specification. Curr Opin Cell Biol 2008;20:91-100. [Crossref] [PubMed]
- Wang Y, Wang H, Ruan J, et al. Long non-coding RNA OIP5-AS1 suppresses multiple myeloma progression by sponging miR-27a-3p to activate TSC1 expression. Cancer Cell Int 2020;20:155. [Crossref] [PubMed]
- Nakamura Y, Tanaka F, Nagahara H, et al. Opa interacting protein 5 (OIP5) is a novel cancer-testis specific gene in gastric cancer. Ann Surg Oncol 2007;14:885-92. [Crossref] [PubMed]
- Gong M, Li Y, Song E, et al. OIP5 Is a Novel Prognostic Biomarker in Clear Cell Renal Cell Cancer Correlating With Immune Infiltrates. Front Immunol 2022;13:805552. [Crossref] [PubMed]
- Schreiber RD, Old LJ, Smyth MJ. Cancer immunoediting: integrating immunity's roles in cancer suppression and promotion. Science 2011;331:1565-70. [Crossref] [PubMed]
- Jiang Y, Wang K, Lu X, et al. Cancer-associated fibroblasts-derived exosomes promote lung cancer progression by OIP5-AS1/ miR-142-5p/ PD-L1 axis. Mol Immunol 2021;140:47-58. [Crossref] [PubMed]
- Zhu Q, Li J, Wu Q, et al. Linc-OIP5 in the breast cancer cells regulates angiogenesis of human umbilical vein endothelial cells through YAP1/Notch/NRP1 signaling circuit at a tumor microenvironment. Biol Res 2020;53:5. [Crossref] [PubMed]
- Bruni D, Angell HK, Galon J. The immune contexture and Immunoscore in cancer prognosis and therapeutic efficacy. Nat Rev Cancer 2020;20:662-80. [Crossref] [PubMed]
- Sun C, Mezzadra R, Schumacher TN. Regulation and Function of the PD-L1 Checkpoint. Immunity 2018;48:434-52. [Crossref] [PubMed]
- He X, Xu C. Immune checkpoint signaling and cancer immunotherapy. Cell Res 2020;30:660-9. [Crossref] [PubMed]
- Litchfield K, Reading JL, Puttick C, et al. Meta-analysis of tumor- and T cell-intrinsic mechanisms of sensitization to checkpoint inhibition. Cell 2021;184:596-614.e14. [Crossref] [PubMed]
- Le DT, Durham JN, Smith KN, et al. Mismatch repair deficiency predicts response of solid tumors to PD-1 blockade. Science 2017;357:409-13. [Crossref] [PubMed]
- Schumacher TN, Schreiber RD. Neoantigens in cancer immunotherapy. Science 2015;348:69-74. [Crossref] [PubMed]
- Kim JM, Chen DS. Immune escape to PD-L1/PD-1 blockade: seven steps to success (or failure). Ann Oncol 2016;27:1492-504. [Crossref] [PubMed]
- Killock D. Lung Cancer: Frontline nivolumab - CheckMate 026 ends in stalemate. Nat Rev Clin Oncol 2017;14:458-9. [Crossref] [PubMed]
- Garon EB, Hellmann MD, Rizvi NA, et al. Five-Year Overall Survival for Patients With Advanced Non‒Small-Cell Lung Cancer Treated With Pembrolizumab: Results From the Phase I KEYNOTE-001 Study. J Clin Oncol 2019;37:2518-27. [Crossref] [PubMed]
- Berger MF, Hodis E, Heffernan TP, et al. Melanoma genome sequencing reveals frequent PREX2 mutations. Nature 2012;485:502-6. [Crossref] [PubMed]
- Sharma AB, Dimitrov S, Hamiche A, et al. Centromeric and ectopic assembly of CENP-A chromatin in health and cancer: old marks and new tracks. Nucleic Acids Res 2019;47:1051-69. [Crossref] [PubMed]
- Yatskevich S, Muir KW, Bellini D, et al. Structure of the human inner kinetochore bound to a centromeric CENP-A nucleosome. Science 2022;376:844-52. [Crossref] [PubMed]
(English Language Editor: J. Reylonds)