The dual role of complement in cancer and its implication in anti-tumor therapy
Introduction
The contribution of inflammation in carcinogenesis has been observed over a century ago with opposing findings as to whether its outcome counters or promotes neoplasia (1,2). Presently, it is believed that acute inflammation participates in mechanisms of immune surveillance directly targeting tumor cells, at the early stages of tumor development or by containing metastatic cells. Chronic inflammation though, has been implicated in both the development and progression of the disease (1,3), where long-term exposure of healthy tissues to pro-inflammatory molecules can induce DNA damage, while inflammatory mediators can maintain and facilitate further evolvement of neoplastic cells (1-3). Complement is a fundamental component of innate immunity, which has been known for many years to be involved in the recognition and assistance in the elimination of invading pathogens (4). However, our current view of complement’s role extends beyond the simple targeting of intruders, since the powerful inflammatory molecules it contains contribute both to acute and chronic inflammation, orchestrating thus immunological and inflammatory processes (5). Moreover, complement participates in largely diverse processes, such as clearance of immune complexes, angiogenesis, mobilization of hematopoietic stem-progenitor cells and tissue regeneration (5-7). A disruption of complement homeostasis can lead to ‘self-attack’ and consequently members of the complement cascade have been implicated in immune-related pathogenesis, including neurodegenerative, ischemic (8) and age-related diseases (9,10). Interestingly, complement is also considered a functional bridge between innate and adaptive immune responses, allowing an integrated host defense to pathogenic challenges (11). These multifunctional properties of the complement system implicate it in opposing roles in cancer (12). First, as a key player in tumor immunoediting, it helps target cancer cells and orchestrates an immune response against the progress of the disease. However, it can also be a part of the long lasting inflammatory status that can lead to malignant transformation and tumor development (13,14).
This review will discuss the role of inflammation in cancer development and progression and will address the involvement of complement in the shaping of the tumor microenvironment. Finally, we will focus on issues confronting the manipulation of complement activation in anti-tumor therapies.
Cancer and inflammation
The role of inflammation in targeting non-self cells is well known, however, most cancer types are highly non-immunogenic thus escaping immune surveillance. Moreover, it has been postulated for many years that chronic inflammation is associated with tumor formation, progression and transformation (15). On the other hand, for several types of tumors, there is no direct link between chronic inflammation and tumor progression, while in some cases, mild chronic inflammation can also lead to reduced tumor risk (asthma, eczema) (16). Of note, the presence of inflammatory cells in certain tumors has been associated with both favorable (17,18) and unfavorable clinical outcome (19-21). This paradox makes apparent the fact that the inflammatory response may differ substantially in different types of tissues. The growth of malignant tumors was often been defined as an autonomous process, but soaring evidence indicates that its dynamics depend greatly on the interaction between malignant cells and the host-derived tumor microenvironment (22). Although cancer cells exhibit a distinct molecular pathway activation signature that promotes their survival, proliferation and transformation (intrinsic pathway), the role of the inflammatory microenvironment (extrinsic pathway) plays an important role in either maintaining or impeding the progress of the disease. The elucidation of the interplay between these two pathways and its modulation is becoming the holly grail for modern anti-tumor approaches.
Hanahan and Weinberg (23) have re-visited their current view regarding the hallmarks of cancer that characterize the biological processes involved in the progress of the disease. The extended list of hallmarks includes (I) sustaining of proliferative signaling; (II) evading growth suppressors; (III) resisting cell death; (IV) enabling replicative immortality; (V) the induction of angiogenesis; (VI) activating invasion and metastasis; (VII) reprogramming of energy metabolism; (VIII) evading immune destruction. In all these steps the way tumor cells interact, depends on their inflammatory microenvironment (23). The role of the tumor microenvironment is thus critical for the progress of the disease, and complement, as an essential part of the inflammatory response, is emerging as an orchestrator of the modulation of immune cells.
One of the multiple roles of the members of the complement cascade is to attract and control the activation of cells involved in both the innate and adaptive immunity. The anaphylatoxins C3a and C5a are powerful chemoattractants that guide neutrophils, monocytes and macrophages and other immune cells towards the sites of inflammation (5). For many years complement was thought to be involved in the targeting of tumor cells, since deposition of complement components has been shown in tissues of various cancer origins (24-26). Current studies are now challenging this dogma, since complement components have been implicated in many of the hallmarks of cancer but with opposing effects (12).
Inflammatory cells and cancer
T cells
CD8+ T cells are a major population of the anti-tumor immune response. These cytotoxic effector T cells (CTL) can recognize MHC class I restricted antigens on cancer cells and initiate cytolytic killing. In most cases, the higher the number of CTLs present at the tumor site, the better the prognosis for a patient is (27). However, tumors are able to escape immune clearance and several reasons for that have been proposed (28). The effects of CTLs are mediated by T-helper (Th) cells; these are CD4+ effector cells that normally lack any direct cytotoxic activity, yet they play a central orchestrating role in adaptive immunity. Th1 response has effective anti-tumor properties by promoting antigen presentation to CTL, but activated Th cells can also mediate tumor clearance independent of antigen presenting cells (APCs). Th cells are quite plastic and can undergo differentiation to regulatory T cells (Treg) that exert immunosuppressive properties, thus hindering effective tumor clearance (29) (Figure 1), or to Th17 cells, whose role in tumors remains highly debated (30,31). Apart from Th-derived Tregs, another population that is CD8+ can also have an immunosuppressive effect in tumors (32,33). Although the mechanism of Treg function is complicated, their depletion can cause decreased tumor burden.
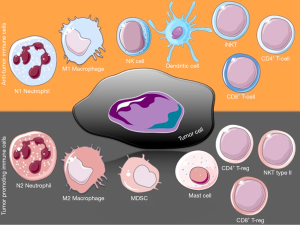
The complement system has been shown to control CD4+ T-cell activation and differentiation (34). C3 mediates Th1/Th17 polarization in human T-cell activation and skin graft versus host disease patients, while blocking of C3 activation with compstatin significantly inhibits Th1/Th17 polarization in activated human CD4+ T cells (35).
Invariant natural killer T cells (iNKT), or type I NKT cells, express a canonical TCR-Vα-chain that recognizes glycolipid antigens presented by the monomorphic CD1d molecule (36). iNKTs are believed to recognize endogenous ligands presented by CD1d on tumor cells, damaged epithelial cells or APCs and result in increased antitumor responses through IFN-γ production (37). Type II NKT cells have diverse repertoires of TCRs and suppress the antitumor response through several mechanisms, including TGF-β production (37). Moreover, they have opposing and counter-acting effects on iNKTs (38), while themselves are being controlled by tumor-controlling iNKTs (39).
Interestingly, engagement of the membrane complement regulatory protein (mCRP) CD46 differentially affects CD8+ T cell cytotoxicity, CD4+ T cell proliferation and IL-2 and IL-10 production (40-42). In addition, complement promotes Th17 differentiation with the participation of TLRs through C5aR signaling (43).
Macrophages
Tumor-associated macrophages (TAMs) comprise one of the largest immune populations in the tumor (44). Their role in tumor immunity can be complicated since they constitute a heterogeneous population that can be either classically activated M1 polarized, or alternatively M2 activated (44). This variation leads to opposing functions, with M1 polarized TAMs having anti-tumor pro-inflammatory properties and M2 TAMs having immune regulatory properties that promote tumor progression. TAMs participate in all stages of cancer progression, from contribution to genetic alterations and instability, regulation of senescence, interaction with and remodeling of the extracellular matrix, to promotion of invasion and metastasis (45). However, the tumor phenotype of TAMs is not always clear, because these cells can share both M1 and M2 characteristics. This variation in the macrophage repertoire and function is tumor type and stage specific and is extensively reviewed by Biswas and Mantovani (46). Activated macrophages produce C3 and participate in complement-initiated phagocytosis of intruding entities, while they are also involved in the clearance of apoptotic and necrotic cells (47). Moreover, C5aR signaling in TLR-activated macrophages selectively inhibits the transcription of genes that encode the IL-12 cytokine family, that in turn drive the polarization and recruitment of Th1 cells (48).
Neutrophils
Polymorphonuclear neutrophils (PMNs) are professional phagocytes with potent anti-microbial and anti-inflammatory capacities. Their role in tumor progression can be opposing, since they can be either pro- or anti-tumorigenic (49). Tumor associated neutrophils (TANs) are believed to employ their dual role according to their polarization. The shift towards N1 polarization promotes tumor cell death, activates CTLs and inhibits tumor growth (50). On the other hand, N2 PMNs are thought to promote tumor angiogenesis and metastasis (51). Their role in tumor promotion is highlighted by Pekrek et al., where depletion of PMNs in an in vivo model resulted in tumor growth inhibition (52).
Dendritic cells (DCs)
DCs are APCs found in tumors and present tumor-associated antigens (TAA) to T cells and NK cells. Large clinical studies have shown that the density of DCs correlates to the number of effector T cells in the tumor and both were associated with improved cell survival (53). However, the local tumor microenvironment was shown to have an effect on the maturation of DCs, thus impeding their anti-tumor activity by affecting CTL response (54). Chronic inflammation can suppress the immunogenicity of DCs and induce a tolerogenic phenotype. In spleen-derived DCs (sDCs), C5aR activation plays an important role for naive CD4+ Th cells to differentiate into either Th1 or Th17 effector cells, while blockade of the receptor in sDCs results in the expansion of Treg, as shown in murine models (55). Additionally, C1q has been to shown to regulate the development of DCs from monocytes while affecting T cell stimulation (56).
Natural killer (NK) cells
NK cells are cytotoxic lymphocytes that recognize MHC-I molecules on target cells and can act directly without the need for prior sensitization (57). Apart from direct killing of cancer cells (58), NK cells produce IFNγ, which is important for Th cell activation that leads to tumor clearance. In a murine melanoma model, C3-/- mice had smaller tumors than wild-type animals, while this effect was abolished after NK depletion in the knockout animals, suggesting increased NK activity in the absence of C3.
Myeloid-derived suppressor cells (MDSCs)
MDSCs are found in the tumors of most cancer patients and experimental animal models. They can be categorized as monocytic and granulocytic MDSCs (59). MDSCs accumulate in response to pro-inflammatory mediators and suppress the activation of CD4+ and CD8+ T cells (60), as well as M1 macrophages and NK cells, thus blocking both innate and adaptive antitumor immunity. Moreover, they facilitate the activation and the anti-inflammatory action of Treg. Of interest, Markiewski et al. have shown the involvement of complement in MDSCs regulation in a murine cancer model (more on section “Modulation of infiltration and activation of immune cells by complement”).
Mast cells
Mast cells are APCs that can promote migration, and maturation of DCs, as well as lymphocyte recruitment (61). Their sentinel presence in epithelial tissues makes them one of the first immune cell populations to come in contact with neoplastic cells. They orchestrate inflammatory reactions and angiogenesis that shape the tumor microenvironment and promote tumor cell proliferation and invasion. Mast cells can affect Treg long-term repercussions (62). However, their presence in tumors has been correlated with both favorable and poor prognosis (61). They express C5aR and C5a has been shown to activate them and to induce degranulation (63), while both C5a and C3a induce chemotaxis (64).
It is becoming apparent that the participation of each immune cell type can have opposing results on tumor pathophysiology. The interplay between these populations depends on the type and stage of tumor. Complement is a known orchestrator of immune responses and is responsible for modulating the functions of most immune cells.
Role of complement in cancer
Modulation of infiltration and activation of immune cells by complement
Despite the multifactorial role of complement in several disease models, little is known regarding its direct implication in the regulation at the tumor-specific setting.
The role of complement in orchestrating the inflammatory state in cancer
Markiewski et al. have shown that complement cascade can regulate inflammatory cells to suppress the immune response and promote tumor growth (14). More specifically, using a murine model of cervical cancer and mice deficient in various complement components (C3, C4, factor B and C5aR) the authors showed that C5a presence in the tumor microenvironment regulates the accumulation and migration of MDSCs, which express receptors for C5a, and boosts the effectiveness of these cells by increasing their content of reactive oxygen and nitrogen species, as well as arginase, all of which contribute to MDSC-mediated immunosuppression. Moreover, this was taken a step further, since the blockade of C5a with either treatment with a peptide antagonist of the C5a receptor, or using C5aR knockout animals, resulted in an increased number of CD8+ CTL in the tumor site. Finally, the importance of C5a involvement in this model was further highlighted when treatment with an established chemotherapeutic agent, paclitaxel (Taxol), showed similar results regarding the retardation of tumor growth to those caused by the pharmaceutical blockade of C5aR (14). The role of C5aR on MDSC modulation was also confirmed in the model Lewis lung carcinoma (3LL) (65). In addition, in a mouse model of breast cancer, C5aR was shown to promote metastasis by suppressing CD8+ T cell function and by enhancing Treg generation (66). In the same model, alveolar macrophages were shown to contribute to the metastatic potential in a C5aR dependent manner (67).
The dual role of complement in angiogenesis
In order for tumors to be able to survive and progress, they require an adequate supply of oxygen and nutrients and an effective way to remove waste products. This is attained by neo-angiogenesis, through a complicated balance of pro- and anti-angiogenic molecules (68). This phenomenon has motivated several researchers to look into therapeutically inhibiting angiogenesis in an attempt to restrain tumor growth. Furthermore, the activation of both angiogenesis and immunosuppressive responses, often occur in the same cell types or are mediated by the same soluble factors (69). Several reports have examined the role of complement in neovascularization in the context of cancer and other models. Nozaki et al showed that the absence of receptors for C3a or C5a, after laser injury, is associated with decreased VEGF expression and neo-angiogenesis and that antibody-mediated neutralization of C3a or C5a or pharmacological blockade of their receptors also reduces neovascularization (70). However, Langer et al. showed, both in vitro and in a model of retinopathy of prematurity, that the deficiency in C3 or C5aR resulted in more neovascularization and angiogenesis (71). Furthermore, in a mouse model of ovarian cancer, Nunez-Cruz et al. showed that C3 and C5aR deficiency resulted in decreased vascularization and tumor growth (72). The proangiogenic effect of complement was further supported by the finding that C5a induced vessel formation in 3LL cells (65). A recent report has addressed the importance of the complement component of the classical pathway C1q in the modulation of tumor growth. More specifically, C1q was shown to be present at the vascular compartment of several types of cancer and its deficiency was associated with decreased vascularization and tumor growth in a murine model of melanoma (73).
The role of complement in cancer-mediated hypoxia
Another role of complement in tumor progression is through the modulation of hypoxia. Hypoxia is characterized by altered cellular metabolism and increased resistance to radiotherapy (74), while it is mostly noted in rapidly expanding tumors, where the oxygen consumption is higher, and in large tumors with no nearby blood vessels. Presence of hypoxia has been correlated with poor prognosis for cancer patients (75). Okroj et al. reported that hypoxia increases susceptibility of non-small cell lung cancer (NSCLC) cells to complement attack. They showed, in an in vitro model, that hypoxic cells had reduced expression of membrane-bound complement inhibitors CD46, CD55 and CD59, as well as decreased secretion of factor I and H. This was followed by increased C3b and C9 deposition on the cells. The authors concluded that hypoxia induced the activation of all three pathways of the complement cascade (76). However, other reports in HUVEC cells (human umbilical vein endothelial cells) have shown that hypoxia causes an increase in complement regulator expression, which might down-regulate complement activation especially after reoxygenation (77), thus highlighting once again the multiple mechanisms in complement’s functions.
Tumor therapy and complement
Complement dependent cytotoxicity and antibody therapy
The development of monoclonal antibody (mAb) therapy has been a hopeful anti-cancer treatment that targets directly antigens uniquely (or mostly) expressed in tumor cells, thus improving the specificity of the therapy (78). mAbs directed against TAA can initiate the natural anti-tumor responses, including complement-dependent cytotoxicity (CDC) (79). CDC is mediated by the C1 complex-initiated complement activation that results in anaphylatoxin production and deposition of C3b fragments on the target cells. C3b, together with C4b and C2a, forms the C5 convertase (C4b2a3b) that in turn cleaves C5 to C5a and C5b. C5b in turn participates in the formation of the C5b-C6-C7-C8-C9 complex that is the subunit component of the membrane attack complex (MAC). MAC formation on the target cell’s surface leads to subsequent cellular lysis (Figure 2). When cancer therapeutic Abs activate the classical complement pathway, they trigger the formation of MAC on cancer cells, leading to the killing of cancer cells through CDC (80). In antibody-dependent cellular cytotoxicity (ADCC), the Fc portion of the antibody is recognized by cells expressing the Fc-receptor (monocytes/macrophages, NK cells and granulocytes) and results in the activation of phagocytic or lytic properties of the effector cells. C3 membrane bound fragments (iC3b) can enhance ADCC by the recognition of iC3b by the complement receptor 3 (CR3) in conjunction with the antibody-Fc-receptor complex. Many clinical trials indicate that although mAb therapy causes no serious side effects, it has most often unsatisfactory results. So far, the most successful immunotherapy is the treatment of B-cell non-Hodgkin’s lymphoma with rituximab, an antibody which recognizes and binds to CD20, with several others also currently being used in the clinic after successfully passing clinical trials, while more are still being evaluated.
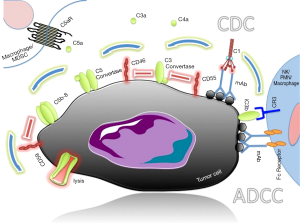
On the other hand, there are cases where CDC is an unwanted side-effect of antibody-based immunotherapies. Immune checkpoint inhibition, such as the blockade of cytotoxic T-lymphocyte antigen-4 (CTLA-4) and programmed cell death protein-1 or its ligand (PD-1/L1), is gaining momentum in several types of cancer. PD-1 is expressed by activated T cells and down-modulates T cell effector functions upon binding to its ligands, PD-L1 and PD-L2, which are present on antigen-presenting cells. In patients with cancer, the expression of PD-1 on tumor-infiltrating lymphocytes and its interaction with its ligands on tumor and immune cells in the tumor microenvironment undermine antitumor immunity and support the rationale for PD-1 blockade in cancer immunotherapy. Several antibodies are currently in advanced clinical trials or have gained regulatory approval, however it is important that such antibodies do not elicit CDC, since the ultimate goal of this treatment is to enhance the function of the T cells and not their elimination (81).
In most cases, the efficiency of antibody-based immunotherapies is compromised by the endogenous inhibitors of the complement system that are uniformly called complement regulatory proteins (CRPs).
CRPs
CRPs are the natural rheostats that protect an organism from undesirable or nonspecific complement activation. They are present in most cells and are categorized in three major classes: those that are (I) in the fluid phase; (II) at the surface of host cell; (III) membrane-integral complement clearance receptors (82). During complement activation, normal cells in the local environment can be susceptible to complement-mediated damage. In order to protect the host cells from unwanted casualties and as a mechanism of regulation of complement cells possess CRPs associated with their cell membranes called mCRPs. The utilization of mAb treatment in cancer is failing to deliver the expected results, since several patients do not respond, while others develop resistance to such treatment. The efficiency of anti-tumor mAb-therapy can be impeded by CRPs (83). Therefore, current studies are looking at the effect of CRP expression by cancer cells in inhibiting CDC (83,84).
mCRPs
Protectin, or CD59, inhibits MAC formation by binding to the C8 and C9 and, while it is universally expressed in normal cells, it is highly expressed in many kinds of cancer cells (85,86). It is very effective in protecting these cells from CDC, thus impairing therapeutic mAb treatment. Several groups have attempted to improve the therapeutic efficiency of mAbs by trying to inhibit the function of CD59. Moreover, a recombinant inhibitor (rILYd4) was utilized against CD59 in a CDC resistant lymphoma cell line and showed a synergistic effect together with the treatment with rituximab both in vitro (85) and in vivo (87). Other groups (88,89) have successfully blocked CD59 using mAbs in a model of NSCLC that led to a similar synergistic effect. Donev et al. (90) used a different approach and identified neural-restrictive silencer factor (REST), as an important regulatory component of the transcriptional machinery of the CD59 gene. They inhibited REST using peptides against the promoter region of CD59 where REST binds. This resulted in a reduced CD59 expression that in turn led to sensitized tumor cells by complement-mediated killing triggered using anti-GD2, a mAb used in neuroblastoma immunotherapy.
Decay-accelerating factor (DAF), or CD55, restricts the action of complement by binding to and dissociating from both the classical (C4b2a) and the alternative (C3bBb) pathway C3/C5 convertases. Several tumor cells express CD55, resulting in increased CDC resistance (91,92), while the expression of CD55 has been demonstrated in hematological malignancies (93), colon, breast, gastric, ovarian, thyroid, prostate, pancreatic, melanoma, glioma, esophageal and cervical cancer (94,95). A decrease in susceptibility to CDC with rituximab was dependent on DAF expression in experiments utilizing an anti-CD55 mAb (96), strengthening the notion that pharmaceutical exploitation of mCRPs can lead to better treatment efficiencies. Moreover, tumor size was positively correlated with DAF expression levels in clinical samples (97,98). In an effort to further explore this mechanism, Zell et al. (99) applied anti-sense phosphorothioate oligonucleotides (S-ODNs) to knock down the expression of CD55 in a number of tumor cell lines and found increased C3 opsonization of the cells together with enhanced CDC. In another study, inhibition of CD55 with siRNA in a human cervical cancer cell line demonstrated increased CDC (100), as was the case in a prostate cancer in vivo model (101). Similarly, overexpression of both CD55 and CD59 was found on NSCLC cells and contributed to the acquisition of resistance to trastuzumab treatment (an anti-HER-2 mAb); in this model, the anticancer efficacy of trastuzumab could be enhanced when these mCRPs were neutralized with blocking mAbs (89).
Membrane cofactor protein (MCP), or CD46, has a cofactor activity for the inactivation of C3b and C4b by factor I. Like most mCRPs, it is expressed on various tumors including head and neck squamous cell carcinoma (HNSCC) (102), hematological malignancies (93,103), breast, prostate (104), ovarian (105,106), glioma (107), liver (108), renal (25) and cervical cancer (109) among others. Together with CD55, downregulation of CD46 with siRNA resulted in increased CDC in a number of cell lines (99,110). Moreover, incubation of lymphoma cells with a recombinant adenovirus-derived protein resulted in transient removal of CD46 from the cell surface, and induced the sensitization of the cells to CDC (111). Buettner et al. have shown that CD46 expression is mediated through signal transducer and activator 3 (STAT3) (104). STAT3 is shown to be overactivated in several tumors (112) and its inhibition led to diminished CD46 expression combined with increased CDC susceptibility in a human prostate cancer cell line (104). Interestingly, Hakulinen et al. have shown that tumor cells produce also a soluble form of CD46 that is fully functional, leading to C3b cleavage by factor I (113). Apart from its mCRP function, CD46 is also a receptor for several pathogens, including measles virus, Neisseria gonorrhoeae and Neisseria meningitides, group A Streptococcus, and human herpes virus 6. This additional property has been exploited by turning CD46 into a CDC-independent tumor target. The oncolytic measles virus can bind to CD46-high expressing multiple myeloma cells, leading to tumor cell death (103). Moreover, human adenoviruses are being utilized for the delivery of gene transfer therapy. Species B adenovirus uses CD46 as their primary cellular-attachment protein, thus making it a good candidate for tumor specific gene targeting (114,115).
Complement receptor-related gene y (Crry) is a murine mCRP that is a functional analog of human DAF and MCP (116). Although many in vivo studies utilize xenograft cells in immunocompromised animals, the use of murine models with animals that are immune intact provides a better insight into the immune response of the organism against the disease. Thus, several studies have used syngeneic models to examine the role of mCRPs in CDC tumor protection. RNAi mediated knock down of Crry in a metastatic cell model has shown enhanced mAb anti-tumor activity in vitro. However, although Crry knock down in vivo led to a decreased tumor burden and higher survival rates, these results were not affected by mAb treatment. The authors concluded that Crry can suppress T cell response, while enhancing complement activation on a tumor cell surface, thus promoting protective T cell immunity (78). Similar results were produced by other groups (117) where mAb inhibition of Crry led to reduced tumorigenicity of murine cell lines in vivo without the need for anti-tumor mAb treatment. On the other hand, older studies had shown that mAb inhibition of Crry in a syngeneic colorectal cancer metastatic model led to increased complement activation and decreased lung tumor formation when combined with an anti-tumor mAb (118,119).
Fluid phase CRPs
Factor H is an essential regulatory protein that plays a critical role in the homeostasis of the complement system. It binds to C3b, thereby preventing subsequent formation of the lytic components at the cell surface. Resistance to factor H-mediated complement attack was found to be significant in ovarian cancer cells, lung cancer and glioblastoma cells (106,120-122). Moreover, the monoclonal antibody cetuximab had significantly higher activity on A549 cells, in which factor H was genetically downregulated with siRNA (122,123).
Factor I is a plasma serine protease that regulates complement activation by cleaving and thus inactivating C3b and C4b. Its activity depends on the presence of cofactors C4BP, MCP and CR1, which can support both C3b and C4b cleavage. Although the primary site of factor I production is the liver, Okroj et al. (88) have shown that functionally active factor I is produced and secreted by some NSCLCs and that, together with C4BP, leads to decreased C3b deposition thus limiting complement-dependent lysis. Moreover, in a recent report, factor I was detected in cutaneous squamous cell carcinomas and its expression was positively associated with tumor aggressiveness (124).
Despite the inhibitory role of CRPs in treatment with monoclonal antibodies, once again complement’s role is dual. Wang et al. have shown, both in vitro (125) and in vivo (126), that complement components can impede the efficiency of rituximab by inhibiting the ability of rituximab-coated targets to induce NK cell activation. They concluded that C1q and C3 presence in the serum was required for these inhibitory effects, while C5 was not involved.
Therapeutic remarks
The high abundance of CRPs in many types of tumors, together with the promising results of the studies conducted so far, has led many to pursue the use of CRP inhibitors as an adjuvant to tumor therapy. Pharmaceutical blockade of selected CRPs, or a combination of them, could indeed result in improved treatment efficiency, treatment of a greater variety of tumors, as well as higher survival rates and better prognosis. However, complement regulators are widely expressed in normal tissues, and random inhibition of CRP function can induce unwanted effects. The development of appropriate tumor-targeting strategies is vital if one wants to use CRP inhibition as an adjuvant treatment. The number of available CRP inhibitors is currently very limited, since the research interest of many groups is mostly focused in developing methods that harness complement activation rather than inducing it. Although several monoclonal antibodies against CRPs exist and newer are emerging (127), the non-specific nature of the treatment makes them hard to utilize. Several steps are taken towards this area, with research groups choosing different approaches.
One option is the use of bi-specific monoclonal antibodies (bs-mAbs). Bs-mAbs are antibodies that consist of fragments from two different mAbs, thus making a unique antibody that can simultaneously recognize two different epitopes. This approach is aiming at combining the tissue-specificity of an anti-tumor mAb together with the CDC effects of an anti-CRP mAb. Such antibodies against Crry, CD59 and CD55 have shown promising results (118,128-131).
A similar approach was followed by Macor et al. (132), using biotin labeled miniantibodies against CD55 and CD59 together with biotin-rituximab. The three-step biotin-avidin system resulted in a specific binding of the anti-CRP miniantibodies to cells where rituximab had specificity (132) and this was associated with increased animal survival.
In an attempt to maximize CDC efficiency in mAb therapy, Sato et al. (133) developed a unique approach, by generating a CDC-enhancing version of rituximab by converting the Fc portion of the human IgG1 heavy chain into IgG3 to create a novel chimeric constant region of mixed IgG1/IgG3 isotype, which possesses enhanced C1q binding properties and increased CDC (133). In their in vivo model they noticed that the potency of their antibody was much higher than rituximab alone.
Although blocking of the mCRP with mAbs enhances complement-mediated immunoclearance of tumors, their high molecular mass and the ubiquitous expression of their targets can pose serious limitations for their application in humans. An alternative approach, down-modulation of mCRPs, has been successfully achieved in vitro by RNA interference (134); however, there are numerous problems (e.g., in vivo stability, tissue specific targeting and unwanted immune system activation) that are currently preventing the use of RNA interference in vivo.
Other approaches to control mCRP expression have involved the use of phosphatidylinositol-phospholipase C (PI-PLC) to down-regulate CD59 in a melanoma cell line that lead to sensitization to mAb treatment (135) or the use of cytokines such as IL1-β and IL-4 in human renal tumor cell lines that lead to down-regulation of mCRPs and increased C3 deposition in the cells (136). However, none of these approaches had a follow-up or were shown to be effective in in vivo models.
Conclusions
For many years the anti-tumor treatment approach focused on targeting the cancer cells themselves. However, current studies reveal that the control of the tumor microenvironment can play a complementary role to this approach. The elucidation of the complex mechanisms involved in the anti-tumor immune response in different types of cancer is required to improve cancer-related treatment and bring fresh weaponry to the bedside. Complement modulation is a promising candidate to supplement existing and design new anti-tumor therapeutics.
Acknowledgements
Figures were produced using Servier Medical Art.
Footnote
Conflicts of Interest: The authors have no conflicts of interest to declare.
References
- de Visser KE, Eichten A, Coussens LM. Paradoxical roles of the immune system during cancer development. Nat Rev Cancer 2006;6:24. [Crossref] [PubMed]
- Hussain SP, Harris CC. Inflammation and cancer: an ancient link with novel potentials. Int J Cancer 2007;121:2373-80. [Crossref] [PubMed]
- Finch CE, Crimmins EM. Inflammatory exposure and historical changes in human life-spans. Science 2004;305:1736-9. [Crossref] [PubMed]
- Markiewski MM, Lambris JD. The role of complement in inflammatory diseases from behind the scenes into the spotlight. Am J Pathol 2007;171:715-27. [Crossref] [PubMed]
- Ricklin D, Hajishengallis G, Yang K, et al. Complement: a key system for immune surveillance and homeostasis. Nat Immunol 2010;11:785-97. [Crossref] [PubMed]
- Merle NS, Noe R, Halbwachs-Mecarelli L, et al. Complement System Part II: Role in Immunity. Front Immunol 2015;6:257. [Crossref] [PubMed]
- Bajic G, Degn SE, Thiel S, et al. Complement activation, regulation, and molecular basis for complement-related diseases. EMBO J 2015;34:2735-57. [Crossref] [PubMed]
- Alawieh A, Elvington A, Tomlinson S. Complement in the Homeostatic and Ischemic Brain. Front Immunol 2015;6:417. [Crossref] [PubMed]
- Warwick A, Khandhadia S, Ennis S, et al. Age-Related Macular Degeneration: A Disease of Systemic or Local Complement Dysregulation? J Clin Med 2014;3:1234-57. [Crossref] [PubMed]
- Ricklin D, Lambris JD. Complement in immune and inflammatory disorders: pathophysiological mechanisms. J. Immunol 2013;190:3831-8. [Crossref] [PubMed]
- Dunkelberger JR, Song WC. Complement and its role in innate and adaptive immune responses. Cell Res 2010;20:34-50. [Crossref] [PubMed]
- Markiewski MM, Lambris JD. Is complement good or bad for cancer patients? A new perspective on an old dilemma. Trends Immunol 2009;30:286-92. [Crossref] [PubMed]
- Rutkowski MJ, Sughrue ME, Kane AJ, et al. Cancer and the complement cascade. Mol Cancer Res 2010;8:1453-65. [Crossref] [PubMed]
- Markiewski MM, DeAngelis RA, Benencia F, et al. Modulation of the antitumor immune response by complement. Nat Immunol 2008;9:1225-35. [Crossref] [PubMed]
- Coussens LM, Werb Z. Inflammation and cancer. Nature 2002;420:860-7. [Crossref] [PubMed]
- El-Zein M, Parent ME, Kâ K, et al. History of asthma or eczema and cancer risk among men: a population-based case-control study in Montreal, Quebec, Canada. Ann Allergy Asthma Immunol 2010;104:378-84. [Crossref] [PubMed]
- Coca S, Perez-Piqueras J, Martinez D, et al. The prognostic significance of intratumoral natural killer cells in patients with colorectal carcinoma. Cancer 1997;79:2320-8. [Crossref] [PubMed]
- Ishigami S, Natsugoe S, Tokuda K, et al. Prognostic value of intratumoral natural killer cells in gastric carcinoma. Cancer 2000;88:577-83. [Crossref] [PubMed]
- Leek RD, Lewis CE, Whitehouse R, et al. Association of macrophage infiltration with angiogenesis and prognosis in invasive breast carcinoma. Cancer Res 1996;56:4625-9. [PubMed]
- Imada A, Shijubo N, Kojima H, et al. Mast cells correlate with angiogenesis and poor outcome in stage I lung adenocarcinoma. Eur Respir J 2000;15:1087-93. [Crossref] [PubMed]
- Ribatti D, Ennas MG, Vacca A, et al. Tumor vascularity and tryptase-positive mast cells correlate with a poor prognosis in melanoma. Eur J Clin Invest 2003;33:420-5. [Crossref] [PubMed]
- Albini A, Sporn MB. The tumour microenvironment as a target for chemoprevention. Nat Rev Cancer 2007;7:139-47. [Crossref] [PubMed]
- Hanahan D, Weinberg RA. Hallmarks of cancer: the next generation. Cell 2011;144:646-74. [Crossref] [PubMed]
- Lucas SD, Karlsson-Parra A, Nilsson B, et al. Tumor-specific deposition of immunoglobulin G and complement in papillary thyroid carcinoma. Hum Pathol 1996;27:1329-35. [Crossref] [PubMed]
- Blok VT, Daha MR, Tijsma OM, et al. A possible role of CD46 for the protection in vivo of human renal tumor cells from complement-mediated damage. Lab Invest 2000;80:335-44. [Crossref] [PubMed]
- Niculescu F, Rus HG, Retegan M, et al. Persistent complement activation on tumor cells in breast cancer. Am J Pathol 1992;140:1039-43. [PubMed]
- Zhang L, Conejo-Garcia JR, Katsaros D, et al. Intratumoral T cells, recurrence, and survival in epithelial ovarian cancer. N Engl J Med 2003;348:203-13. [Crossref] [PubMed]
- Vazquez-Cintron EJ, Monu NR, Frey AB. Tumor-induced disruption of proximal TCR-mediated signal transduction in tumor-infiltrating CD8+ lymphocytes inactivates antitumor effector phase. J Immunol 2010;185:7133-40. [Crossref] [PubMed]
- Campbell DJ, Koch MA. Phenotypical and functional specialization of FOXP3+ regulatory T cells. Nat Rev Immunol 2011;11:119-30. [Crossref] [PubMed]
- Ho IC, Glimcher LH. Transcription: tantalizing times for T cells. Cell 2002;109 Suppl:S109-20. [Crossref] [PubMed]
- Ivanov II, McKenzie BS, Zhou L, et al. The orphan nuclear receptor RORgammat directs the differentiation program of proinflammatory IL-17+ T helper cells. Cell 2006;126:1121-33. [Crossref] [PubMed]
- Wei S, Kryczek I, Zou L, et al. Plasmacytoid dendritic cells induce CD8+ regulatory T cells in human ovarian carcinoma. Cancer Res 2005;65:5020-6. [Crossref] [PubMed]
- Jarnicki AG, Lysaght J, Todryk S, et al. Suppression of antitumor immunity by IL-10 and TGF-beta-producing T cells infiltrating the growing tumor: influence of tumor environment on the induction of CD4+ and CD8+ regulatory T cells. J Immunol 2006;177:896-904. [Crossref] [PubMed]
- Heeger PS, Kemper C. Novel roles of complement in T effector cell regulation. Immunobiology 2012;217:216-24. [Crossref] [PubMed]
- Ma Q, Li D, Carreño R, et al. Complement component C3 mediates Th1/Th17 polarization in human T-cell activation and cutaneous GVHD. Bone Marrow Transplant 2014;49:972-6. [Crossref] [PubMed]
- Gapin L. iNKT cell autoreactivity: what is “self” and how is it recognized? Nat Rev Immunol 2010;10:272-7. [Crossref] [PubMed]
- Flavell RA, Sanjabi S, Wrzesinski SH, et al. The polarization of immune cells in the tumour environment by TGFbeta. Nat Rev Immunol 2010;10:554-67. [Crossref] [PubMed]
- Berzofsky JA, Terabe M. The contrasting roles of NKT cells in tumor immunity. Curr Mol Med 2009;9:667-72. [Crossref] [PubMed]
- Ambrosino E, Terabe M, Halder RC, et al. Cross-regulation between type I and type II NKT cells in regulating tumor immunity: a new immunoregulatory axis. J Immunol 2007;179:5126-36. [Crossref] [PubMed]
- Marie JC, Astier AL, Rivailler P, et al. Linking innate and acquired immunity: divergent role of CD46 cytoplasmic domains in T cell induced inflammation. Nat Immunol 2002;3:659-66. [PubMed]
- Kemper C, Chan AC, Green JM, et al. Activation of human CD4+ cells with CD3 and CD46 induces a T-regulatory cell 1 phenotype. Nature 2003;421:388-92. [Crossref] [PubMed]
- Cardone J, Le Friec G, Vantourout P, et al. Complement regulator CD46 temporally regulates cytokine production by conventional and unconventional T cells. Nat Immunol 2010;11:862-71. [Crossref] [PubMed]
- Fang C, Zhang X, Miwa T, et al. Complement promotes the development of inflammatory T-helper 17 cells through synergistic interaction with Toll-like receptor signaling and interleukin-6 production. Blood 2009;114:1005-15. [Crossref] [PubMed]
- Mantovani A, Sozzani S, Locati M, et al. Macrophage polarization: tumor-associated macrophages as a paradigm for polarized M2 mononuclear phagocytes. Trends Immunol 2002;23:549-55. [Crossref] [PubMed]
- Mantovani A, Sica A. Macrophages, innate immunity and cancer: balance, tolerance, and diversity. Curr Opin Immunol 2010;22:231-7. [Crossref] [PubMed]
- Biswas SK, Mantovani A. Macrophage plasticity and interaction with lymphocyte subsets: cancer as a paradigm. Nat Immunol 2010;11:889-96. [Crossref] [PubMed]
- Gullstrand B, Mårtensson U, Sturfelt G, et al. Complement classical pathway components are all important in clearance of apoptotic and secondary necrotic cells. Clin Exp Immunol 2009;156:303-11. [Crossref] [PubMed]
- Zhang X, Kimura Y, Fang C, et al. Regulation of Toll-like receptor-mediated inflammatory response by complement in vivo. Blood 2007;110:228-36. [Crossref] [PubMed]
- Powell DR, Huttenlocher A. Neutrophils in the Tumor Microenvironment. Trends Immunol 2016;37:41-52. [Crossref] [PubMed]
- Stoppacciaro A, Melani C, Parenza M, et al. Regression of an established tumor genetically modified to release granulocyte colony-stimulating factor requires granulocyte-T cell cooperation and T cell-produced interferon gamma. J Exp Med 1993;178:151-61. [Crossref] [PubMed]
- Fridlender ZG, Sun J, Kim S, et al. Polarization of tumor-associated neutrophil phenotype by TGF-beta: “N1” versus “N2” TAN. Cancer Cell 2009;16:183-94. [Crossref] [PubMed]
- Pekarek LA, Starr BA, Toledano AY, et al. Inhibition of tumor growth by elimination of granulocytes. J Exp Med 1995;181:435-40. [Crossref] [PubMed]
- Ladányi A, Kiss J, Somlai B, et al. Density of DC-LAMP(+) mature dendritic cells in combination with activated T lymphocytes infiltrating primary cutaneous melanoma is a strong independent prognostic factor. Cancer Immunol Immunother 2007;56:1459-69. [Crossref] [PubMed]
- Lin A, Schildknecht A, Nguyen LT, et al. Dendritic cells integrate signals from the tumor microenvironment to modulate immunity and tumor growth. Immunol Lett 2010;127:77-84. [Crossref] [PubMed]
- Weaver DJ, Reis ES, Pandey MK, et al. C5a receptor-deficient dendritic cells promote induction of Treg and Th17 cells. Eur J Immunol 2010;40:710-21. [Crossref] [PubMed]
- Teh BK, Yeo JG, Chern LM, et al. C1q regulation of dendritic cell development from monocytes with distinct cytokine production and T cell stimulation. Mol Immunol 2011;48:1128-38. [Crossref] [PubMed]
- Hokland M, Kuppen PJ. Natural killer cells: from “disturbing” background to central players of immune responses. Mol Immunol 2005;42:381-3. [Crossref] [PubMed]
- Yang Q, Goding SR, Hokland ME, et al. Antitumor activity of NK cells. Immunol Res 2006;36:13-25. [Crossref] [PubMed]
- Youn JI, Nagaraj S, Collazo M, et al. Subsets of myeloid-derived suppressor cells in tumor-bearing mice. J Immunol 2008;181:5791-802. [Crossref] [PubMed]
- Ostrand-Rosenberg S. Myeloid-derived suppressor cells: more mechanisms for inhibiting antitumor immunity. Cancer Immunol Immunother 2010;59:1593-600. [Crossref] [PubMed]
- Khazaie K, Blatner NR, Khan MW, et al. The significant role of mast cells in cancer. Cancer Metastasis Rev 2011;30:45-60. [Crossref] [PubMed]
- Gounaris E, Blatner NR, Dennis K, et al. T-regulatory cells shift from a protective anti-inflammatory to a cancer-promoting proinflammatory phenotype in polyposis. Cancer Res 2009;69:5490-7. [Crossref] [PubMed]
- Subramanian H, Kashem SW, Collington SJ, et al. PMX-53 as a dual CD88 antagonist and an agonist for Mas-related gene 2 (MrgX2) in human mast cells. Mol Pharmacol 2011;79:1005-13. [Crossref] [PubMed]
- Hartmann K, Henz BM, Krüger-Krasagakes S, et al. C3a and C5a stimulate chemotaxis of human mast cells. Blood 1997;89:2863-70. [PubMed]
- Corrales L, Ajona D, Rafail S, et al. Anaphylatoxin C5a creates a favorable microenvironment for lung cancer progression. J Immunol 2012;189:4674-83. [Crossref] [PubMed]
- Vadrevu SK, Chintala NK, Sharma SK, et al. Complement c5a receptor facilitates cancer metastasis by altering T-cell responses in the metastatic niche. Cancer Res 2014;74:3454-65. [Crossref] [PubMed]
- Sharma SK, Chintala NK, Vadrevu SK, et al. Pulmonary alveolar macrophages contribute to the premetastatic niche by suppressing antitumor T cell responses in the lungs. J Immunol 2015;194:5529-38. [Crossref] [PubMed]
- Bergers G, Benjamin LE. Tumorigenesis and the angiogenic switch. Nat Rev Cancer 2003;3:401-10. [Crossref] [PubMed]
- Motz GT, Coukos G. The parallel lives of angiogenesis and immunosuppression: cancer and other tales. Nat Rev Immunol 2011;11:702-11. [Crossref] [PubMed]
- Nozaki M, Raisler BJ, Sakurai E, et al. Drusen complement components C3a and C5a promote choroidal neovascularization. Proc Natl Acad Sci U S A 2006;103:2328-33. [Crossref] [PubMed]
- Langer HF, Chung KJ, Orlova VV, et al. Complement-mediated inhibition of neovascularization reveals a point of convergence between innate immunity and angiogenesis. Blood 2010;116:4395-403. [Crossref] [PubMed]
- Nunez-Cruz S, Gimotty PA, Guerra MW, et al. Genetic and pharmacologic inhibition of complement impairs endothelial cell function and ablates ovarian cancer neovascularization. Neoplasia 2012;14:994-1004. [Crossref] [PubMed]
- Bulla R, Tripodo C, Rami D, et al. C1q acts in the tumour microenvironment as a cancer-promoting factor independently of complement activation. Nat Commun 2016;7:10346. [Crossref] [PubMed]
- Bertout JA, Patel SA, Simon MC. The impact of O2 availability on human cancer. Nat Rev Cancer 2008;8:967-75. [Crossref] [PubMed]
- Vaupel P, Mayer A. Hypoxia in cancer: significance and impact on clinical outcome. Cancer Metastasis Rev 2007;26:225-39. [Crossref] [PubMed]
- Okroj M, Corrales L, Stokowska A, et al. Hypoxia increases susceptibility of non-small cell lung cancer cells to complement attack. Cancer Immunol Immunother 2009;58:1771-80. [Crossref] [PubMed]
- Collard CD, Väkevä A, Büküsoglu C, et al. Reoxygenation of hypoxic human umbilical vein endothelial cells activates the classic complement pathway. Circulation 1997;96:326-33. [Crossref] [PubMed]
- Varela JC, Imai M, Atkinson C, et al. Modulation of protective T cell immunity by complement inhibitor expression on tumor cells. Cancer Res 2008;68:6734-42. [Crossref] [PubMed]
- Taylor RP, Lindorfer MA. The role of complement in mAb-based therapies of cancer. Methods 2014;65:18-27. [Crossref] [PubMed]
- Gelderman KA, Tomlinson S, Ross GD, et al. Complement function in mAb-mediated cancer immunotherapy. Trends Immunol 2004;25:158-64. [Crossref] [PubMed]
- Homet Moreno B, Ribas A. Anti-programmed cell death protein-1/ligand-1 therapy in different cancers. Br J Cancer 2015;112:1421-7. [Crossref] [PubMed]
- Zipfel PF, Skerka C. Complement regulators and inhibitory proteins. Nat Rev Immunol 2009;9:729-40. [PubMed]
- Yan J, Allendorf DJ, Li B, et al. The role of membrane complement regulatory proteins in cancer immunotherapy. Adv Exp Med Biol 2008;632:159-74. [Crossref] [PubMed]
- Meyer S, Leusen JH, Boross P. Regulation of complement and modulation of its activity in monoclonal antibody therapy of cancer. MAbs 2014;6:1133-44. [Crossref] [PubMed]
- You T, Hu W, Ge X, et al. Application of a novel inhibitor of human CD59 for the enhancement of complement-dependent cytolysis on cancer cells. Cell Mol Immunol 2011;8:157-63. [Crossref] [PubMed]
- Geis N, Zell S, Rutz R, et al. Inhibition of membrane complement inhibitor expression (CD46, CD55, CD59) by siRNA sensitizes tumor cells to complement attack in vitro. Curr Cancer Drug Targets 2010;10:922-31. [Crossref] [PubMed]
- Hu W, Ge X, You T, et al. Human CD59 inhibitor sensitizes rituximab-resistant lymphoma cells to complement-mediated cytolysis. Cancer Res 2011;71:2298-307. [Crossref] [PubMed]
- Okroj M, Hsu YF, Ajona D, et al. Non-small cell lung cancer cells produce a functional set of complement factor I and its soluble cofactors. Mol Immunol 2008;45:169-79. [Crossref] [PubMed]
- Zhao WP, Zhu B, Duan YZ, et al. Neutralization of complement regulatory proteins CD55 and CD59 augments therapeutic effect of herceptin against lung carcinoma cells. Oncol Rep 2009;21:1405-11. [PubMed]
- Donev RM, Gray LC, Sivasankar B, et al. Modulation of CD59 expression by restrictive silencer factor-derived peptides in cancer immunotherapy for neuroblastoma. Cancer Res 2008;68:5979-87. [Crossref] [PubMed]
- Liu J, Miwa T, Hilliard B, et al. The complement inhibitory protein DAF (CD55) suppresses T cell immunity in vivo. J Exp Med 2005;201:567-77. [Crossref] [PubMed]
- Morgan J, Spendlove I, Durrant LG. The role of CD55 in protecting the tumour environment from complement attack. Tissue Antigens 2002;60:213-23. [Crossref] [PubMed]
- Hara T, Kojima A, Fukuda H, et al. Levels of complement regulatory proteins, CD35 (CR1), CD46 (MCP) and CD55 (DAF) in human haematological malignancies. Br J Haematol 1992;82:368-73. [Crossref] [PubMed]
- Spendlove I, Ramage JM, Bradley R, et al. Complement decay accelerating factor (DAF)/CD55 in cancer. Cancer Immunol Immunother 2006;55:987-95. [Crossref] [PubMed]
- Mikesch JH, Schier K, Roetger A, et al. The expression and action of decay-accelerating factor (CD55) in human malignancies and cancer therapy. Cell Oncol 2006;28:223-32. [PubMed]
- Ziller F, Macor P, Bulla R, et al. Controlling complement resistance in cancer by using human monoclonal antibodies that neutralize complement-regulatory proteins CD55 and CD59. Eur J Immunol 2005;35:2175-83. [Crossref] [PubMed]
- Terui Y, Sakurai T, Mishima Y, et al. Blockade of bulky lymphoma-associated CD55 expression by RNA interference overcomes resistance to complement-dependent cytotoxicity with rituximab. Cancer Sci 2006;97:72-9. [Crossref] [PubMed]
- Mikesch JH, Buerger H, Simon R, et al. Decay-accelerating factor (CD55): a versatile acting molecule in human malignancies. Biochim Biophys Acta 2006;1766:42-52.
- Zell S, Geis N, Rutz R, et al. Down-regulation of CD55 and CD46 expression by anti-sense phosphorothioate oligonucleotides (S-ODNs) sensitizes tumour cells to complement attack. Clin Exp Immunol 2007;150:576-84. [Crossref] [PubMed]
- Gao LJ, Ding L, Guo SY, et al. Role of decay-accelerating factor in regulating survival of human cervical cancer cells. J Cancer Res Clin Oncol 2011;137:81-7. [Crossref] [PubMed]
- Loberg RD, Day LL, Dunn R, et al. Inhibition of decay-accelerating factor (CD55) attenuates prostate cancer growth and survival in vivo. Neoplasia 2006;8:69-78. [Crossref] [PubMed]
- Ravindranath NM, Shuler C. Expression of complement restriction factors (CD46, CD55 & CD59) in head and neck squamous cell carcinomas. J Oral Pathol Med 2006;35:560-7. [Crossref] [PubMed]
- Ong HT, Timm MM, Greipp PR, et al. Oncolytic measles virus targets high CD46 expression on multiple myeloma cells. Exp Hematol 2006;34:713-20. [Crossref] [PubMed]
- Buettner R, Huang M, Gritsko T, et al. Activated signal transducers and activators of transcription 3 signaling induces CD46 expression and protects human cancer cells from complement-dependent cytotoxicity. Mol Cancer Res 2007;5:823-32. [Crossref] [PubMed]
- Surowiak P, Materna V, Maciejczyk A, et al. CD46 expression is indicative of shorter revival-free survival for ovarian cancer patients. Anticancer Res 2006;26:4943-8. [PubMed]
- Junnikkala S, Hakulinen J, Jarva H, et al. Secretion of soluble complement inhibitors factor H and factor H-like protein (FHL-1) by ovarian tumour cells. Br J Cancer 2002;87:1119-27. [Crossref] [PubMed]
- Ulasov IV, Tyler MA, Zheng S, et al. CD46 represents a target for adenoviral gene therapy of malignant glioma. Hum Gene Ther 2006;17:556-64. [Crossref] [PubMed]
- Kinugasa N, Higashi T, Nouso K, et al. Expression of membrane cofactor protein (MCP, CD46) in human liver diseases. Br J Cancer 1999;80:1820-5. [Crossref] [PubMed]
- Gao LJ, Guo SY, Cai YQ, et al. Cooperation of decay-accelerating factor and membrane cofactor protein in regulating survival of human cervical cancer cells. BMC Cancer 2009;9:384. [Crossref] [PubMed]
- Mamidi S, Höne S, Teufel C, et al. Neutralization of membrane complement regulators improves complement-dependent effector functions of therapeutic anticancer antibodies targeting leukemic cells. Oncoimmunology 2015;4:e979688. [Crossref] [PubMed]
- Wang H, Liu Y, Li ZY, et al. A recombinant adenovirus type 35 fiber knob protein sensitizes lymphoma cells to rituximab therapy. Blood 2010;115:592-600. [Crossref] [PubMed]
- Buettner R, Mora LB, Jove R. Activated STAT signaling in human tumors provides novel molecular targets for therapeutic intervention. Clin Cancer Res 2002;8:945-54. [PubMed]
- Hakulinen J, Junnikkala S, Sorsa T, et al. Complement inhibitor membrane cofactor protein (MCP; CD46) is constitutively shed from cancer cell membranes in vesicles and converted by a metalloproteinase to a functionally active soluble form. Eur J Immunol 2004;34:2620-9. [Crossref] [PubMed]
- Wang Y, Ma L, Wang S, et al. Assessment of CAR- or CD46-dependent adenoviral vector-mediated TRAIL gene therapy in clinical adenocarcinoma lung cancer cells. Oncology 2009;77:366-77. [Crossref] [PubMed]
- Hall K, Blair Zajdel ME, Blair GE. Unity and diversity in the human adenoviruses: exploiting alternative entry pathways for gene therapy. Biochem J 2010;431:321-36. [Crossref] [PubMed]
- Foley S, Li B, Dehoff M, et al. Mouse Crry/p65 is a regulator of the alternative pathway of complement activation. Eur J Immunol 1993;23:1381-4. [Crossref] [PubMed]
- Ohta R, Kondor N, Dohi N, et al. Mouse complement receptor-related gene y/p65-neutralized tumor vaccine induces antitumor activity in vivo. J Immunol 2004;173:205-13. [Crossref] [PubMed]
- Gelderman KA, Kuppen PJ, Okada N, et al. Tumor-specific inhibition of membrane-bound complement regulatory protein Crry with bispecific monoclonal antibodies prevents tumor outgrowth in a rat colorectal cancer lung metastases model. Cancer Res 2004;64:4366-72. [Crossref] [PubMed]
- Gelderman KA, Hakulinen J, Hagenaars M, et al. Membrane-bound complement regulatory proteins inhibit complement activation by an immunotherapeutic mAb in a syngeneic rat colorectal cancer model. Mol Immunol 2003;40:13-23. [Crossref] [PubMed]
- Wilczek E, Rzepko R, Nowis D, et al. The possible role of factor H in colon cancer resistance to complement attack. Int J Cancer 2008;122:2030-7. [Crossref] [PubMed]
- Junnikkala S, Jokiranta TS, Friese MA, et al. Exceptional resistance of human H2 glioblastoma cells to complement-mediated killing by expression and utilization of factor H and factor H-like protein 1. J Immunol 2000;164:6075-81. [Crossref] [PubMed]
- Ajona D, Castaño Z, Garayoa M, et al. Expression of complement factor H by lung cancer cells: effects on the activation of the alternative pathway of complement. Cancer Res 2004;64:6310-8. [Crossref] [PubMed]
- Hsu YF, Ajona D, Corrales L, et al. Complement activation mediates cetuximab inhibition of non-small cell lung cancer tumor growth in vivo. Mol Cancer 2010;9:139. [Crossref] [PubMed]
- Riihilä P, Nissinen L, Farshchian M, et al. Complement factor I promotes progression of cutaneous squamous cell carcinoma. J Invest Dermatol 2015;135:579-88. [Crossref] [PubMed]
- Wang SY, Racila E, Taylor RP, et al. NK-cell activation and antibody-dependent cellular cytotoxicity induced by rituximab-coated target cells is inhibited by the C3b component of complement. Blood 2008;111:1456-63. [Crossref] [PubMed]
- Wang SY, Veeramani S, Racila E, et al. Depletion of the C3 component of complement enhances the ability of rituximab-coated target cells to activate human NK cells and improves the efficacy of monoclonal antibody therapy in an in vivo model. Blood 2009;114:5322-30. [Crossref] [PubMed]
- Guo B, Ma Z, Li H, et al. Mapping of binding epitopes of a human decay-accelerating factor monoclonal antibody capable of enhancing rituximab-mediated complement-dependent cytotoxicity. Clin Immunol 2008;128:155-63. [Crossref] [PubMed]
- Gelderman KA, Lam S, Gorter A. Inhibiting complement regulators in cancer immunotherapy with bispecific mAbs. Expert Opin Biol Ther 2005;5:1593-601. [Crossref] [PubMed]
- Gelderman KA, Kuppen PJ, Bruin W, et al. Enhancement of the complement activating capacity of 17-1A mAb to overcome the effect of membrane-bound complement regulatory proteins on colorectal carcinoma. Eur J Immunol 2002;32:128-35. [Crossref] [PubMed]
- Gelderman KA, Blok VT, Fleuren GJ, et al. The inhibitory effect of CD46, CD55, and CD59 on complement activation after immunotherapeutic treatment of cervical carcinoma cells with monoclonal antibodies or bispecific monoclonal antibodies. Lab Invest 2002;82:483-93. [Crossref] [PubMed]
- Macor P, Secco E, Mezzaroba N, et al. Bispecific antibodies targeting tumor-associated antigens and neutralizing complement regulators increase the efficacy of antibody-based immunotherapy in mice. Leukemia 2015;29:406-14. [Crossref] [PubMed]
- Macor P, Tripodo C, Zorzet S, et al. In vivo targeting of human neutralizing antibodies against CD55 and CD59 to lymphoma cells increases the antitumor activity of rituximab. Cancer Res 2007;67:10556-63. [Crossref] [PubMed]
- Sato F, Ito A, Ishida T, et al. A complement-dependent cytotoxicity-enhancing anti-CD20 antibody mediating potent antitumor activity in the humanized NOD/Shi-scid, IL-2Rγ(null) mouse lymphoma model. Cancer Immunol Immunother 2010;59:1791-800. [Crossref] [PubMed]
- Cinci M, Mamidi S, Li W, et al. Targeted delivery of siRNA using transferrin-coupled lipoplexes specifically sensitizes CD71 high expressing malignant cells to antibody-mediated complement attack. Target Oncol 2015;10:405-13. [Crossref] [PubMed]
- Brasoveanu LI, Altomonte M, et al. Levels of cell membrane CD59 regulate the extent of complement-mediated lysis of human melanoma cells. Lab Invest 1996;74:33-42. [PubMed]
- Blok VT, Gelderman KA, Tijsma OHM, et al. Cytokines affect resistance of human renal tumour cells to complement-mediated injury. Scand J Immunol 2003;57:591-9. [Crossref] [PubMed]