A snapshot of lung cancer: where are we now?—a narrative review
Introduction
The global impact of cancer and cancer-related deaths has been a huge challenge and continues to be a setback in the health sector and beyond even in recent times. However, recent advances in screening and surveillance, diagnostic tools, new treatment interventions as well as close monitoring are promising in the effective management and decrease of cancer-related mortality and morbidity. Cancer ranks as a leading cause of death globally and the first or second leading cause of mortality before age 70 in a significant number of countries globally with lung cancer (LC) being the second most prevalent malignancy and the leading cause of mortality amongst cancers in men and women worldwide (1). LC still constitutes a major burden despite recent advances in diagnostic modalities such as genomic sequencing and evolution of tumor markers, early diagnostic screening, identification and prevention of risk factors, modification of environmental hazards as well as treatment with systemic therapy. The life expectancy from the time of diagnosis and or management is somewhere short of 5 years, which clearly differs from other common malignancies like prostate and breast cancer. The rationale behind this study is aimed at identifying current trends in LC, especially non-small cell lung cancer (NSCLC) which happens to be the most common subtype, and the genetic alterations that exist in NSCLC populations. We present the following article in accordance with the Narrative Review reporting checklist (available at https://atm.amegroups.com/article/view/10.21037/atm-22-4479/rc).
Methods
After a structured review of the literature between the years, 2000–2022, a database search was performed using English language as highlighted in Table 1 and Table S1, and a narrative study design was implemented in this research.
Table 1
Items | Specification |
---|---|
Date of search | 10/10/2021–8/25/2022 |
Databases | PubMed, ERIC, SEER, Cochrane |
Search terms used | Table S1 |
Time frame | 2000–2022 |
Inclusion and exclusion criteria | Inclusion: narrative study, English language |
Selection process | The selection was conducted as a consensus by the authors involved |
ERIC, Education Resources Information Center; SEER, Surveillance, Epidemiology, and End Results.
LC subtypes
LCs are broadly classified traditionally into small cell lung cancer (SCLC) and NSCLC based on microscopic appearance with SCLC making up roughly 15% of all LCs and NSCLC which is more predominant, comprising roughly 85% of lung tumors. According to the 2021 World Health Organization’s latest classification of thoracic tumors, LC can be sub-classified into epithelial tumors, lung neuroendocrine neoplasms, tumors of ectopic tissues, and mesenchymal tumors specific to the lung. Epithelial tumors are more common and can be subclassified into common entities such as adenocarcinomas, squamous cell carcinoma (SCC), large-cell carcinoma, and pleomorphic carcinoma amongst others (2). Neuroendocrine tumors on the other hand are subdivided into small cell carcinoma and large cell neuroendocrine carcinoma as well as carcinoid tumors-although rare. The less prevalent LCs are tumors of ectopic tissues and mesenchymal tumors but can occur nonetheless. It is also important to note that pre-invasive lesions do exist but that entity has not been captured in this article.
Epidemiology, LC racial disparity, and risk factors
Epidemiology
Globally, LC is the leading cause of cancer-related mortality and was responsible for 1.8 million deaths, or 18% of all deaths due to cancer in 2020. LC is the second most frequently diagnosed form of cancer with an annual global incidence of 2.2 million or 11.4% of the total number of new cancer diagnoses in 2020. LC incidence is surpassed only by breast cancer at 2.3 million or 11.7% of all new cancer diagnoses in 2020 (3).
LC rates vary globally with a considerably higher rate in men occurring in developed nations than in developing nations. However, the overall incidence is higher in women than men in developed nations and there has been an increasing incidence of LC in women worldwide (4). It is believed that LC-related mortality is relatively more predominant in men than women despite the rising incidence of occurrence in women, but the gap may soon narrow down in the nearest future. This disparity has been attributed to the differential rate of smoking in male versus female populations. Likewise, the enormous effort put into the reduction of smoking rates has seen LC rates between male and female populations in the United states approach parity (5).
In the United States, the overall incidence of LC is higher in non-Hispanic whites than in any other ethnic group (Figure 1). On the other hand, the incidence rate is about 47% more prevalent in African American (AA) men than in white men with even greater mortality, but there seems to be an equal incidence rate in AA women and women of European American (EA) descent. The incidence among American Indians/Alaskan natives, Hispanics, and Asians appears to be significantly lower than the incidence in AA with slightly better survival outcomes for Asians (6). Advanced age has also been strongly implicated due to the shortening of telomeres and DNA damage over time. LC is usually diagnosed around the median age of 70 for both men and women (7).
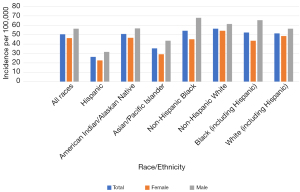
Both the incidence and mortality of LC have seen a steady decline over the past thirty years from a peak incidence of 66 and a mortality rate of 59 per 100,000 in 1992 down to an incidence of 41 and a mortality of 33 per 100,000 in 2019 (8). This constitutes a decrease of 38% in annual incidence and a decrease of 44% in annual mortality. The disproportionate decline in annual mortality yields a 5-year survival rate for LC diagnosed in 2014 that is 75% greater than that of LC diagnosed in 1990 (13.4 vs. 23.4 per 100,000). The National Cancer Institute estimates that LC will account for approximately 236,740 new cases or 12.3% of all new cancer diagnoses in 2022 and 130,180 deaths or 21.4% of all cancer mortality for 2022. If current trends continue, projections for 5-year survival rates for LC diagnosed in 2022 could reach as high as 30–35% (8). While the overall incidence and mortality of LC have fallen, most of the decrease is attributed to the decline in incidence and mortality for males whereas the incidence and mortality of female LC patients have seen only modest decrease (Figure 2A-2D).
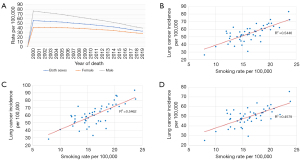
Disparities in LC incidence and mortality
Racial disparities continue to exist in the field of LC and more research is ongoing to bridge the gap in the disparities that exist currently. More research is needed to obtain better understanding of the challenges faced by racial minorities in the overall management of LC. Racial disparities are predominant, as AA males have the highest rates of age-adjusted LC incidence among all U.S. racial/ethnic groups, specifically 73.5 per 100,000 versus 63.5 per 100,000 for white males (9). AA males also have the highest mortality rate compared with other racial/ethnic groups. Black males usually develop LC at an earlier age which is not clearly understood and are also more likely to present with advanced disease; 53% among black individuals vs. 49% in the white population (10). Additionally, these disparities are noted in both smokers as well as non-smokers. Hispanics on the other hand tend to have a lower smoking prevalence and mortality from LC, especially when compared to black males and white males. American Indians and Alaskan natives have the highest overall prevalence of cigarette smoking yet have lower incidence and mortality rates when compared with blacks (9).
Low socioeconomic populations experience more exposure to environmental hazards and risk factors (such as exposure to secondhand smoking and biofuels pollution) that are associated with cancer which is mostly evidenced in people who live in poor resource geographical areas. Blacks who live in poor settings, especially in rural areas are believed to have a greater incidence of LC than those in urban areas and are also thought to have a higher incidence when compared to the whites who live in both rural and urban settings respectively (11). This may be partly explained by low socio-economic income, low literacy levels, minimal access to healthcare services, and to an extent, lack of black representation amongst healthcare providers. This may also be explained by the genetic constitution of individual racial groups but more research is needed to explain these findings.
There is a strong association between socioeconomic status and LC incidence with Poor income significantly associated with cigarette smoking which inadvertently leads to an increase in the risk of LC. A study by Dalton and his colleagues have also associated low socioeconomic, without smoking history with an increased incidence of LC possibly as a result of suboptimal living/housing conditions with high exposure to secondary smoke and occupational exposure (12). Common risk factors associated with LC include tobacco smoking, radon exposure, asbestos, environmental pollution, poor air quality, infections such as human immunodeficiency virus (HIV) and tuberculosis (TB), genetic predisposition as well as potentially delayed consequences of marijuana smoking and e-cigarette vaping which have been on a rapid increase over the last decade both in popularity, ease of access and consumption (13).
Nuclear genetic alterations in LC
The hypothesis of LC occurs in a multistep model and is believed to arise from genome-wide tumorigenesis events, which supports the Darwinian evolution model of the LC genome. The understanding of this model is highly beneficial in deciphering the appropriate treatment of LC and its precursors based on etiopathogenesis (14). The early lung carcinogenesis model is strongly supported by genomic evolution at the single nucleotide level and demarcated evolution at the chromosomal level. Many intermediate pulmonary nodules are now increasingly identified with the advent of CT imaging. They include atypical adenomatous hyperplasia (AAH), preinvasive adenocarcinoma in situ (AIS), minimally invasive adenocarcinoma (MIA), or sometimes early invasive lung adenocarcinoma (ADC).
The molecular basis of these lesions is not well understood but it has been postulated that the preneoplasia AAH may progress to AIS, MIA, and also invasive ADC. In a recent study by Dejima and his colleagues, it was noted that the immune contexture progressed as a continuum from AAH to ADC which, in overall, expresses progressive genomic evolution thus implying that early carcinogenesis of lung ADC is a gradual process strongly influenced by host immune surveillance (15).
Genetic sequencing has led to tremendous improvement in the management of LC over the last decade. It primarily focuses on genome sequencing of lung para-neoplasia, pre-invasive and early invasive LC; however, an article by Hu and his colleagues showed that studies are not comprehensive due to the scarcity of available resected specimens as many indeterminate pulmonary nodules are not resected and biopsied early enough (16). Hopefully, we will see improvement in this field in the nearest future provided nodules are sampled earlier when identified but the timing and the size of the nodule may be a possible limiting factor in the early sampling of the nodule especially as surgical resection of an indeterminate pulmonary nodule is not the standard of care.
Also, Somatic mutation has led to aberrations at the DNA, RNA, protein, and epigenetic levels. Rapid advancements in next-generation sequencing and a better understanding of cancer biology have led to the identification of several biomarkers implicated in the etiopathogenesis of LC which has further led to the evolution of targeted therapies. They include TP53, KRAS, EGFR, BRAF, HER, NF1, MET, and RIT, which are commonly seen in ADC while mutations in TP53, CDKN2A, PIK3CA, NFE2L2, KEAP1NF1 and epidermal growth factor receptor (EGFR), a transmembrane protein that serves as a receptor for members of the epidermal growth factor family are frequently identified in SCCs. Common alterations in ADC are involved in RTK/RAS/RAF, mTOR, JAK-STAT, DNA repair, cell regulation, and epigenetic deregulation with most therapeutic agents targeting EGFR and BRAF mutations as well as rearrangements in genes responsible for making proteins involved in cell growth and cell signaling—anaplastic lymphoma kinase (ALK), and c-ros oncogene 1 (ROS1) (17).
EGFR, which is usually frequently expressed in the etiopathogenesis, development, and progression of NSCLC is known to regulate important tumorigenic processes including proliferation, apoptosis, angiogenesis, and invasion. EGFR is abnormally activated by mutation in over 50% of all NSCLC which leads to increased cell proliferation and survival via RAS/RAF/MEK/MAPK and PI3K/AKT pathways. Similarly, 20% of all NSCLCs arise from an activating mutation in the KRAS gene from the RAS proto-oncogene family (18). Erb/HER2 protein kinase is also one of the biomarkers identified in the etiopathogenesis of LC and ErbB2/HER2 amplified cell lines are known to have high levels of phosphorylation of components of the EGFR pathway as well as other receptor tyrosine kinases like C-MET. Overexpression of EGFR biomarkers as well as other molecular biomarkers such as HER-2 protein kinase, C-MET, and their downward cascade of genetic alterations are summarized in Figure 3. Genomics and proteomic tools have helped in the identification of molecules associated with a specific cancer phenotype. EGFR and KRAS molecules have been extensively researched and found to be associated with many lung tumors. They also serve as therapeutic targets which include monoclonal antibodies (mAb) and EGFR tyrosine kinase inhibitors (TKIs) in LC patients (18). Additionally, the genetic makeup of LCs has been linked with the response to chemotherapy and radiotherapy (RT).
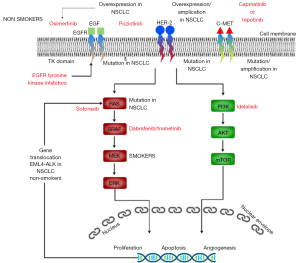
Targeted agents despite their benefits are still somewhat ineffective for most LC subtypes and many targeted therapies are currently undergoing trials however molecular genotyping/tumor profiling is now routine in the diagnosis of ADC. Despite the recent advances in genomic research and targeted therapies, there is still a poor outcome in LC patients as most cases are diagnosed in the advanced stage.
Mitochondrial DNA (mtDNA) alterations in LC
In addition to the nuclear DNA (nDNA) alterations, changes in mtDNA also appear to play a critical role in human tumorigenesis (19-28). Mitochondria are unique cytoplasmic organelles in the cells, uniquely possess their own DNA (16.5 kb), and serve as an integral part of the oxidative phosphorylation system (OXPHOS) in producing cellular adenosine triphosphate (ATP). They are composed of five respiratory complexes (RCs) (I–V), which are escorted and assembled from multiple polypeptides; some encoded by mtDNA and others by nDNA. The human mitochondrial genome (MG) is a double-stranded closed circular molecule, that codes for the 12S and 16S rRNAs, 22 tRNAs, and 13 proteins essential for the mitochondrial RC activity (Figure 4) (29). The majority of the human cells contain multiple copies of mtDNA and nearly all of these mtDNA copies are identical, i.e., homoplasmic at birth as the mtDNA follows a strict maternal mode of inheritance. The mutation rate in mtDNA is approximately 10 times higher than that of nDNA and much easier to trace in cancer cells because of the available abundant copies.
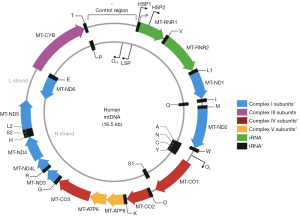
LC is a mitochondria-enriched tumor possibly due to its highly recurrent nature. Earlier studies from our laboratory identified numerous mtDNA mutations in both smoker and non-smoker NSCLC patients (23,24). The majority of the mtDNA mutations were detected in the respiratory complex-1 (RCI) region of the mtDNA, and mtDNA mutations were found to be associated with EGFR gene mutation in never-smoker LC patients (23). Moreover, RCI-targeted mutation appeared to be associated with enhanced tumorigenic potential and reactive oxygen species (ROS) generation in LC cells. Notably, RCI serves as an entry gate for the electron transport chain (ETC) to commence and a major site for ROS generation through electron leaking (23,29). On the other hand, alterations in RCI may attenuate the onset of intrinsic apoptosis, a highly desired pathway of recurrent tumors. In an earlier study, Sidransky’s laboratory detected somatic mtDNA mutation in 43% (6/14) of the LC patients they analyzed (30). A total of 10 mutations were detected and all were from the noncoding D-Loop, 16SrRNA, and tRNA regions of the MG. Another study sequenced MG in peripheral blood, normal, and cancer tissues from 55 LC (31). Sixty percent of the patients (33/55) have exhibited 56 mutations including point mutation, nucleotide insertions, and deletions. Nineteen of these mutations were nonsynonymous coding mtDNA mutations from 9 different RC genes. By employing a next-generation sequencing approach, a recent study comprehensively examined the mtDNA mutation spectrum in 61 stage-I lung adenocarcinomas (LUAD) (32). Frequent germline and somatic mtDNA mutations encompassing the non-coding and coding regions of the MG were detected. The highest frequency of germline mtDNA mutations were detected in the non-coding D-Loop and coding CYTB (RCIII) regions. Notably, somatic mutations in RCIV and RCV genes were found to be associated with shorter relapse-free survival (RFS) of LUAD patients. In another interesting study, Yang and his colleagues examined the extent of D-Loop mtDNA mutation in noninvasively collected exhaled breath condensate from 9 LC patients, 10 smokers/ex-smokers, and 6 non-smokers subjects without a diagnosis of cancer (33). Significantly increased D-Loop mutations were evident in the LC patients compared to the controls with a higher frequency of T16217C nucleotide transition. Among the various non-coding regions of the mtDNA, a mutation in the tRNAs appears to be a hotspot and reported in LC (34). Utilizing a unique pathogenicity scoring system on various tRNA mutations in LC, Wang and his colleagues have found A12172G nucleotide transition (tRNA-His) as a pathogenic mutation (34). In addition to somatic and germline mtDNA mutations, anomalies in mtDNA content have been presented in a glut of human malignancies (23,24). Alteration in mtDNA copy number could be a measure of reprogrammed mitochondrial function, and thus could be useful in biomarker development. Increased mtDNA content has been reported in LC patients and found to be associated with an increased risk of the disease (23,24,35). Thus, a connection between mtDNA alterations and lung carcinogenesis is increasingly becoming evident.
Microbiome and LC
The role of inflammation and microbiome in the etiology of chronic lung diseases such as chronic obstructive pulmonary disease (COPD), chronic pulmonary fibrosis, and asthma has been extensively studied. Chronic lung inflammation plays a significant role in the pathogenesis of LC and this serves as the initial focal point for understanding the role of microbiomes in LC. The healthy lung was previously believed to be sterile, however, a study by Dong and his colleagues suggests that lung microbiome has been cultured in both healthy as well as diseased lungs and usually contains a complex of bacteria such as Prevotella, Streptococcus, Hemophilus, Staphylococcus, Pseudomonas, etc. (36-38). Nevertheless, a distinguishing factor between the lung microbiome and the gut or skin microbiome is its low biomass when compared to the gastrointestinal microbiome (39). With highly improved sequencing techniques, lung microbiota has been strongly associated with LC—the underlying mechanism explained by distinguished local microbiome featured by increased total bacterial abundance, altered bacterial composition, and reduced alpha diversity (36). Evidence suggests a direct relationship between LC and the lung microbiome. Epidemiological data reveals that up to 50–70% of LC cases are complicated by microbial pulmonary infections with its pathogenesis poorly understood. On the other hand, post-obstructive pneumonia can negatively affect the efficacy of LC therapy and the overall survival of cancer patients (36).
Liu and his colleagues have studied the various mechanisms through which the microbiome could play a role in the etiology of LC. These include inflammatory and immune changes which could reflect microbiological dysbiosis that allow pathogens to thrive (37). There is a strong link between chronic bacterial infection with Helicobacter pylori and gastric cancer which arises from pro-inflammatory events. This exact principle is thought to be responsible for the development of LC, especially in cases of repeated lung infections or chronic lung inflammatory diseases such as TB. Studies have shown a possible link between TB and LC primarily because of the potential of TB infections to be foci for inflammation. A recent Danish study compared the cancer risk in TB patients during 1978–2011 with a significantly increased risk noticed in the general population (38). A similar study in Taiwan and South Korea also strongly associated an increased risk of LC with pulmonary TB (38). Chronic fibrosis from TB is believed to decrease the clearance of lung lymph and lymph-associated particles and these have been linked to the suppression of p21. Deregulation of the mechanisms that control p21 expression in the cell cycle is believed to contribute to tumorigenesis. Additionally, increased expression of Bcl-2 will reduce apoptosis leading to genomic instability, and finally, pro-inflammatory activation of cyclooxygenase 2 (COX-2) to produce eicosanoids which encourage angiogenesis that play an essential role in tumorigenesis (37).
LC screening
LC continues to have poor survival rates which emphasize the need for proper screening to help identify individuals at risk as this could prevent cancer progression. The goal is to cure the disease, especially in cases of NSCLC if it is found early and treated. According to the US preventive services task force (USPSTF), annual spiral low-dose computed tomography (CT) chest imaging is the acceptable screening tool for at-risk populations (adults aged 50–80 who have a 20-pack-year smoking history and currently smoke or have quit within the past 15 years). Further, the USPSTF reported that the current evidence was not substantial to either recommend or warn against the use of screening tools to detect LC in asymptomatic patients (40).
Current treatment plans of NSCLC
The treatment of LC is complex and involves the use of systemic therapy which includes chemotherapy, immunotherapy and targeted therapy, RT, and surgery. According to the National Comprehensive Cancer Network (NCCN) guidelines, the current treatment of LC depends on the subclassification of LC, stage of lesion, presence or absence of mediastinal lymph nodes, and tumor operability. Important pretreatment work-up assessment includes pulmonary function test, bronchoscopy, mediastinal lymph node evaluation (such as mediastinoscopy, endobronchial ultrasound), fluorodeoxyglucose positron emission tomography/CT (FDG PET/CT) scan, and brain magnetic resonance imaging (MRI) with contrast, especially for stage II and beyond but can be optional for stage I. For NSCLC with negative mediastinal nodes, surgical resection plus lymph node dissection or systemic node sampling and adjuvant systemic therapy are the current treatment guideline while definitive RT with adjuvant systemic therapy is the guideline for high-risk stages in medically inoperable NSCLC with no nodal involvement.
Systemic therapy
Approximately 20–25% of patients diagnosed with NSCLC have resectable disease however, roughly over 30% of patients who undergo surgical treatment with curative intent have a recurrence and ultimately die of their disease. Stage I NSCLC is usually treated by surgical resection and adjuvant chemotherapy is given post-surgery for high-risk patients, according to the NCCN guidelines. In a neoadjuvant context, Immunotherapy and chemotherapy provide an early intervention in preventing metastatic disease as well as a reduction in the bulk of the tumor. In a recent study by Forde and his colleagues, it was observed amongst patients with resectable NSCLC, that neoadjuvant nivolumab plus chemotherapy resulted in significantly longer event-free survival, pathological complete response, radiological response, and overall survival than chemotherapy alone with greater outcomes seen in patients with stage IIIA disease than in those with stage IB and II disease in most subgroups from their study (41). The results from their study were obtained from a phase 3 clinical trial by Check-Mate 816 (ClinicalTrials.gov number, NCT02998528). Another recent study—a phase III clinical trial (IMPower 010) by Wakelee and his colleagues demonstrates the disease survival benefit of adjuvant immunotherapy with atezolizumab (anti PD-L1) over best supportive care (BSC) with adjuvant platinum-based chemotherapy in patients with early-stage (IB-IIIA) resected NSCLC wand outcomes were more pronounced in PD-L1 tumor concentration ≥1% (42). Similarly, Osimertinib was found to show improvement in disease-free survival in patients with early disease following surgery for resected stages IB-IIIA EGFR mutated NSCLC as observed in the phase III ADAURA study. This study favors the use of adjuvant osimertinib as an effective treatment for patients with stages IB-IIIA EGFR positive NSCLC following complete tumor resection, with or without previous adjuvant chemotherapy (43).
On the other hand, concurrent chemoradiotherapy (CRT) and consolidative immunotherapy with durvalumab have demonstrated significant improvements with primary endpoints of overall survival and progression-free survival in unresectable stage III NSCLC which is based on the outcome of a phase 3 randomized placebo-controlled clinical study of patients with stage III NSCLC (PACIFIC Trial: NCT02125461). This new regimen is now more favored over platinum-based concurrent CRT which used to be the standard of care for locally advanced unresectable stage III NSCLC and good performance status, especially in patients without disease progression following chemoradiation (44).
For advanced LC (stage IV), broad molecular profiling is strongly recommended by the NCCN for driver mutations as treatments are directed at these molecular biomarkers/driver mutations. Several molecular targets (Tables 2,3) have been identified with therapy targeted against them (45). EGFR, a receptor tyrosine kinase is the most commonly described, and the most common mutations in EGFR are associated with responsiveness to oral TKIs. Others include oral ALK TKIs, ROS1 TKIs, and BRAF mutation-p. V600E-specific mAb is commercially available but should only be used after extensive validation. Checkpoint inhibitor antibodies are also recognized as a beneficial targeted therapy by blocking the PD-1 and PD-L1 interaction, thus improving the antitumor effects of endogenous T cells. Regarding stage IV NSCLC with or without brain metastasis and no actionable driver mutations (highlighted in Tables 2,3), immunotherapy with pembrolizumab plus platinum-based histology-specific chemotherapy improved clinical outcomes in patients with PD-L1 expression including PD-L1 proportion score less than 1% who had good performance status/safety profile as observed in the KEYNOTE studies (46). Similarly, a recent phase 3 randomized study (CheckMate9LA) revealed a significant overall survival benefit, regardless of PD-L1 expression, with dual immune checkpoint inhibitors (nivolumab, a fully human anti-PD-1 antibody, and Ipilimumab, a fully human anti-CTLA4 antibody) combined with two cycles of chemotherapy compared to standard therapy with four cycles of chemotherapy, in the first line treatment of patients with NSCLC (47).
Table 2
Tumor driver mutation | Performance status | Current recommendations |
---|---|---|
HER2 mutation (Clinical Trial NCT03066206) HER2 | 0–1 | Poziotinib |
EGFR sensitizing mutation | 0–2 | Osimertinib monotherapy |
3 | EGFR tyrosine kinase inhibitor | |
ALK gene rearrangement | 0–2 | Alectinib or brigatinib |
RET rearrangement | 0–2 | Selpercatinib or pralsetinib |
ROS1 rearrangement | 0–2 | Crizotinib or entrectinib |
BRAF V600E | 0–2 | Dabrafenib/trametinib |
MET exon 14 skipping mutation | 0–2 | Capmatinib or tepotinib |
NTRK rearrangement | 0–2 | Entrectinib or larotrectinib |
NSCLC, non-small cell lung cancer; HER2, human epidermal growth factor receptor 2; EGFR, epidermal growth factor receptor; ALK, anaplastic lymphoma kinase; RET proto-oncogene, rearranged during transfection; ROS1, c-ros oncogene 1; BRAF, v-raf murine sarcoma viral oncogene homolog B1; MET, mesenchymal-epithelial transition factor; NTRK, neurotrophic tyrosine receptor kinase.
Table 3
Tumor driven mutation | Performance status | Current recommendations |
---|---|---|
HER2 mutation/overexpression (Clinical Trial, NCT03505710) | 0–1 | Trastuzumab deruxtecan if refractory to standard treatment, relapsed disease or where standard treatment is not available |
EGFR sensitizing mutation | 0–2 | For EGFR T790M resistance, osimertinib if not given as first-line therapy |
ALK rearrangement | 0–2 | (I) Alectinib, brigatinib, or ceritinib if crizotinib was given as first-line therapy |
(II) Lorlatinib if previously received alectinib or brigatinib | ||
ROS1 rearrangement | 0–2 | (I) Standard therapy following non-driver mutation guideline if previously treated with ROS1 targeted therapy |
(II) Crizotinib or entrectinib or ceritinib for patients previously treated with nontargeted therapy first line | ||
BRAF mutations | 0–2 | (I) Standard therapy following non-driver mutation guidelines if previously treated with BRAF/MEK targeted therapy |
(II) Dabrafenib/trametinib for patients previously treated with chemotherapy or chemotherapy/immunotherapy | ||
(III) Standard therapy following non-driver mutation guidelines if previously treated with chemotherapy, immunotherapy, and BRAF targeted therapy | ||
(IV) Standard therapy following non-driver mutation guidelines for BRAF mutations other than BRAF V600E mutation | ||
MET Exon 14 skipping mutation | 0–2 | (I) Standard therapy following non-driver mutation guidelines if previously treated with MET targeted therapy or patients with mutations other than exon 14 skipping mutations |
(II) MET targeted therapy with Capmatinib or Tepotinib for patients previously treated with or without immunotherapy or chemotherapy or ineligible for first-line therapy | ||
RET mutations | 0–2 | (I) Standard therapy following non-driver mutation guidelines if previously treated with RET-targeted therapy |
(II) Selpercatinib for patients who have not received RET targeted therapy | ||
NTRK rearrangement | 0–2 | (I) Standard therapy following non-driver mutation guidelines if previously treated with NTRK inhibitor |
(II) Entrectinib or larotrectinib if not previously treated with NTRK inhibitor |
NSCLC, non-small cell lung cancer; HER2, human epidermal growth factor receptor 2; EGFR, epidermal growth factor receptor; ALK, anaplastic lymphoma kinase; ROS1, c-ros oncogene 1; BRAF, v-raf murine sarcoma viral oncogene homolog B1; MET, mesenchymal-epithelial transition factor; RET proto-oncogene, rearranged during transfection; NTRK, neurotrophic tyrosine receptor kinase; MEK, mitogen-activated protein kinase.
Surgery
Surgical resection in resectable tumors remains the most successful option for patients with LC. According to the NCCN guidelines, resection is the preferred local treatment modality [other modalities include stereotactic ablative body radiotherapy (SABR), thermal ablation such as radiofrequency ablation, and cryotherapy] for medically operable diseases. Surgery is the standard treatment modality for stage I NSCLC with curative intent and patients with pathologic stage II or greater, or high-risk factors should be referred to medical oncology for evaluation. Surgery is integral in the diagnosis, staging, and curative treatment of LC. It is also helpful in perioperative mediastinal staging which is essential in the management of NSCLC especially to determine prognosis. The final aim of surgical treatment is complete resection which is defined as the total removal of the primary tumor with no residual macroscopic or microscopic tumor left behind and the highest negative mediastinal lymph node being negative. The standard operative procedures include lobectomy and pneumonectomy with lobectomy and lymphadenectomy or hilar and mediastinal lymph node sampling being the treatment of choice for patients with stage I and II NSCLC (48). Lobectomy was observed to have superior benefits to wedge resection and segmentectomy which had less survival and a greater risk of disease recurrence by about 50% based on a randomized study by Ginsberg and his colleagues on behalf of the Lung Cancer Study Group (49). Anatomical segmentectomy on the other hand is recommended for patients with significant co-morbidities or patients with limited pulmonary function tests. Video-assisted thoracoscopic surgery (VATS) has been strongly favored and is increasingly being used as specific access to the thoracic cavity mostly in early-stage LC. VATS has favorable long-term survival benefits as it has the advantage of minimal blood loss intra and post-operatively, early chest tube removal, reduced complications, and decreased length of stay (48).
RT
RT is the only treatment modality that can be utilized across all stages of NSCLC either for curative or palliative intent irrespective of performance status. For stages I and IIA NSCLC, SABR are utilized for those with inoperable tumors or who decline surgery with improved overall survival as observed in the CHISEL trial (50). Stereotactic RT techniques include fixation, ultraprecise treatment planning, RT directed to gross disease alone, and high doses per fraction. They are used to treat small lung tumors (T1-2, N0, M0). Hadron therapy is another form of RT with potential benefits such as higher relative biologic effectiveness, excellent dose distribution, and higher linear energy transfer but it is limited by its high cost and complexity (40). For stage IV NSCLC which is advanced and mostly considered as incurable, ablative surgery may be helpful in patients with oligometastatic disease and has shown prolonged survival benefits. Per European consensus, synchronous oligometastases is defined as up to five distant metastases in a maximum of three organs based on comprehensive imaging including a PET scan and brain MRI (51).
Future perspective
LC remains the leading cause of cancer-related deaths globally with NSCLC accounting for the most part of the cancer with a low survival rate. The incidence has declined in most developed countries and peaked in some developed as well as underdeveloped nations. It is imperative that more research and clinical trials be undertaken to appreciate an in-depth understanding of LC from the molecular level to facilitate the discovery of more targeted therapy and better overall management of LC. With respect to targeted therapy, some emerging biomarkers are being extensively studied to identify novel target agents. They include high level MET amplification and ERBB2 (HER2) mutations. The future of LC is strongly focused on epigenetics which encompasses DNA methylation, microRNAs, histone modifications, copious reinforcing signals, and chromatin modeling (51). On the other hand, a combination of RT and immunotherapy is an interesting field of study at this time. It is believed that ionizing radiation can lead to danger signals which can increase immunogenicity that can become an efficient individualized in-situ vaccine that enhances the effect of immunotherapy (50). This, hopefully, will help in the treatment of both early and advanced NSCLC with minimal adverse outcomes.
A better and robust understanding of epigenetics will better equip us to understand the etiopathogenesis of LC which will inadvertently lead to favorable outcomes as future therapy will primarily be aimed at targeting identified biomarkers/genetic alterations and mutations. At the same time, unraveling the nature and timing of mitochondrial genetic alterations and delineating their functional upshots along the path of LC progression and racial disparity will enable us to develop novel biomarkers and therapeutic targets. Considering the accumulating pieces of evidence on the causative role of various pathogenic microbes in human tumorigenesis, identification and functional characterization of the microbial niche in LC may open up novel avenues for cancer detection and therapeutics. Further efforts are also needed to identify the major cause or causes of LCs arising in never smokers before successful strategies for prevention, early diagnosis and novel therapies can be implemented.
Conclusions
LC remains the leading cause of cancer-related deaths globally with poor prognosis despite available treatment options and even recent advances in both diagnostic tools and management guidelines. Lung microbiome dysbiosis, Human nuclear and mitochondrial alterations clearly play a role in tumorigenesis and progressive genomic evolution is crucial in the early carcinogenesis of LC which is strongly influenced by host immune surveillance. Several molecular targets have been identified in the etiopathogenesis of LC with therapeutic targets mostly centered around EGFR and BRAF as well as other genetic mutations.
It is imperative that more research and clinical trials be undertaken to appreciate an in-depth understanding of LC from the molecular level to facilitate the discovery of more targeted therapy and overall better management of LC.
Acknowledgments
Funding: This work is supported by funding from Mitchell Cancer Institute and the University of South Alabama (to SD).
Footnote
Reporting Checklist: The authors have completed the Narrative Review reporting checklist. Available at https://atm.amegroups.com/article/view/10.21037/atm-22-4479/rc
Conflicts of Interest: All authors have completed the ICMJE uniform disclosure form (available at https://atm.amegroups.com/article/view/10.21037/atm-22-4479/coif). SD serves as an unpaid editorial board member of Annals of Translational Medicine from September 2018 to August 2024. The other authors have no conflicts of interest to declare.
Ethical Statement: The authors are accountable for all aspects of the work in ensuring that questions related to the accuracy or integrity of any part of the work are appropriately investigated and resolved.
Open Access Statement: This is an Open Access article distributed in accordance with the Creative Commons Attribution-NonCommercial-NoDerivs 4.0 International License (CC BY-NC-ND 4.0), which permits the non-commercial replication and distribution of the article with the strict proviso that no changes or edits are made and the original work is properly cited (including links to both the formal publication through the relevant DOI and the license). See: https://creativecommons.org/licenses/by-nc-nd/4.0/.
References
- Bray F, Laversanne M, Weiderpass E, et al. The ever-increasing importance of cancer as a leading cause of premature death worldwide. Cancer 2021;127:3029-30. [Crossref] [PubMed]
- Nicholson AG, Tsao MS, Beasley MB, et al. The 2021 WHO Classification of Lung Tumors: Impact of Advances Since 2015. J Thorac Oncol 2022;17:362-87. [Crossref] [PubMed]
- Sung H, Ferlay J, Siegel RL, et al. Global Cancer Statistics 2020: GLOBOCAN Estimates of Incidence and Mortality Worldwide for 36 Cancers in 185 Countries. CA Cancer J Clin 2021;71:209-49. [Crossref] [PubMed]
- Youlden DR, Cramb SM, Baade PD. The International Epidemiology of Lung Cancer: geographical distribution and secular trends. J Thorac Oncol 2008;3:819-31. [Crossref] [PubMed]
- Siegel RL, Miller KD, Jemal A. Cancer statistics, 2018. CA Cancer J Clin 2018;68:7-30. [Crossref] [PubMed]
- Ries LA, Melbert D, Krapcho M, et al. SEER cancer statistics review, 1975–2005. Bethesda, MD: National Cancer Institute. 2008 Jul; 2999.
- Torre LA, Siegel RL, Jemal A. Lung Cancer Statistics. Adv Exp Med Biol 2016;893:1-19. [Crossref] [PubMed]
- Available online: https://seer.cancer.gov/statfacts/html/lungb.html
- Howlader NN, Krapcho M, Miller D, et al. SEER Cancer Statistics Review, 1975–2016 National Cancer Institute. Bethesda, MD2019 [based on November 2018 SEER data submission, posted to the SEER web site, April 9].
- DeSantis CE, Miller KD, Goding Sauer A, et al. Cancer statistics for African Americans, 2019. CA Cancer J Clin 2019;69:211-33. [Crossref] [PubMed]
- Houston KA, Mitchell KA, King J, et al. Histologic Lung Cancer Incidence Rates and Trends Vary by Race/Ethnicity and Residential County. J Thorac Oncol 2018;13:497-509. [Crossref] [PubMed]
- Dalton SO, Frederiksen BL, Jacobsen E, et al. Socioeconomic position, stage of lung cancer and time between referral and diagnosis in Denmark, 2001-2008. Br J Cancer 2011;105:1042-8. [Crossref] [PubMed]
- de Groot PM, Wu CC, Carter BW, et al. The epidemiology of lung cancer. Transl Lung Cancer Res 2018;7:220-33. [Crossref] [PubMed]
- Ahn S, Lim J, Park SY, et al. Genetic Alterations in Preinvasive Lung Synchronous Lesions. Cancer Res Treat 2020;52:1120-34. [Crossref] [PubMed]
- Dejima H, Hu X, Chen R, et al. Immune evolution from preneoplasia to invasive lung adenocarcinomas and underlying molecular features. Nat Commun 2021;12:2722. [Crossref] [PubMed]
- Hu X, Fujimoto J, Ying L, et al. Multi-region exome sequencing reveals genomic evolution from preneoplasia to lung adenocarcinoma. Nat Commun 2019;10:2978. [Crossref] [PubMed]
- Chang YS, Tu SJ, Chen YC, et al. Mutation profile of non-small cell lung cancer revealed by next generation sequencing. Respir Res 2021;22:3. [Crossref] [PubMed]
- Pastor MD, Nogal A, Molina-Pinelo S, et al. Oncoproteomic approaches in lung cancer research. Edited by César López-Camarillo and Elena Aréchaga-Ocampo. 2013 Mar 13:169.
- Ishikawa K, Takenaga K, Akimoto M, et al. ROS-generating mitochondrial DNA mutations can regulate tumor cell metastasis. Science 2008;320:661-4. [Crossref] [PubMed]
- Zhou S, Kachhap S, Sun W, et al. Frequency and phenotypic implications of mitochondrial DNA mutations in human squamous cell cancers of the head and neck. Proc Natl Acad Sci U S A 2007;104:7540-5. [Crossref] [PubMed]
- Dasgupta S, Hoque MO, Upadhyay S, et al. Mitochondrial cytochrome B gene mutation promotes tumor growth in bladder cancer. Cancer Res 2008;68:700-6. [Crossref] [PubMed]
- Dasgupta S, Hoque MO, Upadhyay S, et al. Forced cytochrome B gene mutation expression induces mitochondrial proliferation and prevents apoptosis in human uroepithelial SV-HUC-1 cells. Int J Cancer 2009;125:2829-35. [Crossref] [PubMed]
- Dasgupta S, Soudry E, Mukhopadhyay N, et al. Mitochondrial DNA mutations in respiratory complex-I in never-smoker lung cancer patients contribute to lung cancer progression and associated with EGFR gene mutation. J Cell Physiol 2012;227:2451-60. [Crossref] [PubMed]
- Dasgupta S, Yung RC, Westra WH, et al. Following mitochondrial footprints through a long mucosal path to lung cancer. PLoS One 2009;4:e6533. [Crossref] [PubMed]
- Kopinski PK, Singh LN, Zhang S, et al. Mitochondrial DNA variation and cancer. Nat Rev Cancer 2021;21:431-45. [Crossref] [PubMed]
- Koshikawa N, Akimoto M, Hayashi JI, et al. Association of predicted pathogenic mutations in mitochondrial ND genes with distant metastasis in NSCLC and colon cancer. Sci Rep 2017;7:15535. [Crossref] [PubMed]
- Amer W, Toth C, Vassella E, et al. Evolution analysis of heterogeneous non-small cell lung carcinoma by ultra-deep sequencing of the mitochondrial genome. Sci Rep 2017;7:11069. [Crossref] [PubMed]
- Gammage PA, Frezza C. Mitochondrial DNA: the overlooked oncogenome? BMC Biol 2019;17:53. [Crossref] [PubMed]
- Hertweck KL, Dasgupta S. The Landscape of mtDNA Modifications in Cancer: A Tale of Two Cities. Front Oncol 2017;7:262. [Crossref] [PubMed]
- Fliss MS, Usadel H, Caballero OL, et al. Facile detection of mitochondrial DNA mutations in tumors and bodily fluids. Science 2000;287:2017-9. [Crossref] [PubMed]
- Jin X, Zhang J, Gao Y, et al. Relationship between mitochondrial DNA mutations and clinical characteristics in human lung cancer. Mitochondrion 2007;7:347-53. [Crossref] [PubMed]
- Raghav L, Chang YH, Hsu YC, et al. Landscape of Mitochondria Genome and Clinical Outcomes in Stage 1 Lung Adenocarcinoma. Cancers (Basel) 2020;12:755. [Crossref] [PubMed]
- Yang Ai SS, Hsu K, Herbert C, et al. Mitochondrial DNA mutations in exhaled breath condensate of patients with lung cancer. Respir Med 2013;107:911-8. [Crossref] [PubMed]
- Wang L, Chen ZJ, Zhang YK, et al. The role of mitochondrial tRNA mutations in lung cancer. Int J Clin Exp Med 2015;8:13341-6. [PubMed]
- Hosgood HD 3rd, Liu CS, Rothman N, et al. Mitochondrial DNA copy number and lung cancer risk in a prospective cohort study. Carcinogenesis 2010;31:847-9. [Crossref] [PubMed]
- Dong Q, Chen ES, Zhao C, et al. Host-Microbiome Interaction in Lung Cancer. Front Immunol 2021;12:679829. [Crossref] [PubMed]
- Liu NN, Ma Q, Ge Y, et al. Microbiome dysbiosis in lung cancer: from composition to therapy. NPJ Precis Oncol 2020;4:33. [Crossref] [PubMed]
- Mur LA, Huws SA, Cameron SJ, et al. Lung cancer: a new frontier for microbiome research and clinical translation. Ecancermedicalscience 2018;12:866. [Crossref] [PubMed]
- Suau A, Bonnet R, Sutren M, et al. Direct analysis of genes encoding 16S rRNA from complex communities reveals many novel molecular species within the human gut. Appl Environ Microbiol 1999;65:4799-807. [Crossref] [PubMed]
- Molina JR, Yang P, Cassivi SD, et al. Non-small cell lung cancer: epidemiology, risk factors, treatment, and survivorship. Mayo Clin Proc 2008;83:584-94. [Crossref] [PubMed]
- Forde PM, Spicer J, Lu S, et al. Neoadjuvant Nivolumab plus Chemotherapy in Resectable Lung Cancer. N Engl J Med 2022;386:1973-85. [Crossref] [PubMed]
- Wakelee HA, Altorki NK, Zhou C, et al. IMpower010: Primary results of a phase III global study of atezolizumab versus best supportive care after adjuvant chemotherapy in resected stage IB-IIIA non-small cell lung cancer (NSCLC). J Clin Oncol 2021;39:8500. [Crossref]
- Wu YL, John T, Grohe C, et al. Postoperative Chemotherapy Use and Outcomes From ADAURA: Osimertinib as Adjuvant Therapy for Resected EGFR-Mutated NSCLC. J Thorac Oncol 2022;17:423-33. [Crossref] [PubMed]
- Faivre-Finn C, Vicente D, Kurata T, et al. Four-Year Survival With Durvalumab After Chemoradiotherapy in Stage III NSCLC-an Update From the PACIFIC Trial. J Thorac Oncol 2021;16:860-7. [Crossref] [PubMed]
- Singh N, Temin S, Baker S Jr, et al. Therapy for Stage IV Non-Small-Cell Lung Cancer With Driver Alterations: ASCO Living Guideline. J Clin Oncol 2022;40:3310-22. [Crossref] [PubMed]
- Powell SF, Rodríguez-Abreu D, Langer CJ, et al. Outcomes With Pembrolizumab Plus Platinum-Based Chemotherapy for Patients With NSCLC and Stable Brain Metastases: Pooled Analysis of KEYNOTE-021, -189, and -407. J Thorac Oncol 2021;16:1883-92. [Crossref] [PubMed]
- Paz-Ares L, Ciuleanu TE, Cobo M, et al. First-line nivolumab plus ipilimumab combined with two cycles of chemotherapy in patients with non-small-cell lung cancer (CheckMate 9LA): an international, randomised, open-label, phase 3 trial. Lancet Oncol 2021;22:198-211. [Crossref] [PubMed]
- Dziedzic R, Marjański T, Rzyman W. A narrative review of invasive diagnostics and treatment of early lung cancer. Transl Lung Cancer Res 2021;10:1110-23. [Crossref] [PubMed]
- Ginsberg RJ, Rubinstein LV. Randomized trial of lobectomy versus limited resection for T1 N0 non-small cell lung cancer. Lung Cancer Study Group. Ann Thorac Surg 1995;60:615-22; discussion 622-3. [Crossref] [PubMed]
- Vinod SK, Hau E. Radiotherapy treatment for lung cancer: Current status and future directions. Respirology 2020;25:61-71. [Crossref] [PubMed]
- Jha G, Azhar S, Rashid U, et al. Epigenetics: The Key to Future Diagnostics and Therapeutics of Lung Cancer. Cureus 2021;13:e19770. [Crossref] [PubMed]