The inhibitory effect of isoliquiritigenin on human platelets in vitro
Highlight box
Key findings
• ILTG could inhibit the collagen- and thrombin-induced platelet aggregation and granule release via the glycoprotein VI-mediated signal pathway.
What is known and what is new?
• ILTG has an inhibitory effect on platelet aggregation. ILTG can inhibit various important molecules in platelets, including COX, lipoxygenase, and peroxidase.
• ILTG could inhibit collagen- and thrombin-induced platelet aggregation. ILTG could inhibit the phosphorylation of PLCγ2 and Akt, suggesting ILTG had an inhibiting effect on the collagen–GPVI signaling pathway.
What is the implication, and what should change now?
• ILTG has antiplatelet effect and great potential for use in clinic. More studies are need to clarify the antiplatelet mechanism of ILTG and evaluate its potential in clinical application.
Introduction
Platelets are anucleate blood cells that are mainly produced by megakaryocytes and participate in multiple physiological and pathophysiological processes (1). Platelets have a short lifespan, only existing in blood for 7–10 days, but they are heterogenous and play important roles in homeostasis maintenance and disease progression (2). Activated platelets adhere to the exposed extracellular matrix underlying endothelial cells, resulting in the formation of a platelet plug to stop bleeding (3). In addition to their central roles in hemostasis, platelets have important roles in immune modulation, anti-infection activity, and tissue repair (4). Platelets have been found to participate in the activation of leukocytes and the clearance of pathogens (5-7). As secretory cells, the substances released by platelets, including growth factors, chemokines, and coagulant factors, can alter the microenvironment and influence the activity of adjacent cells (8,9).
Platelets participate in several pathological processes, such as disseminated intravascular coagulation (DIC), atherosclerosis, tumor progression, and inflammation. Multiple antiplatelet drugs have been developed to modulate platelet function. Cyclooxygenase 1 (COX1) inhibitor, adenosine diphosphate (ADP) receptor antagonist, and integrin αIIbβ3 inhibitor are 3 kinds of drugs commonly used to treat cardiovascular disease (2). Aspirin, a COX1 inhibitor, can inhibit the activation of platelets by blocking the formation of thromboxane A2 (TXA2) (10,11). Combining a COX1 inhibitor and an ADP receptor antagonist is the standard therapy to prevent atherothrombotic events (2). Clopidogrel, an ADP receptor inhibitor, can irreversibly bind to the P2Y12 receptor of platelets, exerting an antiplatelet effect (12). Integrin αIIbβ3 inhibitors, including abciximab, eptifibatide, and tirofiban, can suppress the aggregation of platelets (12). However, while antiplatelet agents can prevent the occurrence of thrombosis events, they can also increase the risk of bleeding (2,13). Thus, we need to develop novel antiplatelet drugs to provide more personalized treatment strategies.
Licorice, a Chinese traditional medicine, has been shown to exert antiplatelet activity. Flavonoids are considered to be the main biologically active components of licorice. Studies revealed that flavonoids exert different pharmacological functions, such as antiplatelet, anti-inflammatory, anticancer, antioxidative, antimicrobial, immunomodulatory, and cardioprotective effects (14-16). Ten chemicals have been identified from flavonoids, and one of them, isoliquiritigenin (ILTG), was found to have anti-inflammatory, antioxidative, and anticancer properties (17-19). ILTG was shown to inhibit the degradation of IκBα and further block the activation of nuclear factor kappa beta (NF-κB) in endothelial cells, intestinal epithelial cells, and macrophages (17,20,21). Tawata et al. (22) found ILTG to have an inhibitory effect on platelet aggregation, comparable to that of aspirin, and this effect was associated with the inhibition of arachidonic acid metabolism. This study demonstrated that ILTG can inhibit various important molecules in platelets, including COX, lipoxygenase, and peroxidase (22) but did not clarify the underlying mechanism.
In our study, we investigated the antiplatelet effect of ILTG in vitro and further identified the underlining mechanism. Our results showed that ILTG could inhibit collagen- and thrombin-induced platelet aggregation, highlighting its potential for use in clinic. We present the following article in accordance with the MDAR reporting checklist (available at https://atm.amegroups.com/article/view/10.21037/atm-22-2839/rc).
Methods
Volunteer recruitment and sample collection
The blood samples were collected from 14 healthy volunteers, including 3 male and 11 female participants. All participants were aged between 20 and 30 years and had no acute or chronic disease, or history of thrombosis, hemorrhagic, or autoimmune diseases. Over the previous 2 weeks, they had not taken any antiplatelet drugs. The female participants were in a nonmenstrual period. Informed consent was obtained from all volunteers at the start of our study. In accordance with the Declaration of Helsinki (as revised in 2013), the study was approved by the review boards of Union Hospital and Tongji Medical College (IORG No. IORG0003571). A total of 30 mL of whole blood was obtained with a sodium citrate anticoagulant tube. The platelet-rich plasma (PRP), washed platelets, and platelet-poor plasma (PPP) were isolated from the whole blood.
Preparation of PRP, PPP, and washed platelets
Whole blood was centrifuged (160 ×g, 15 min) at room temperature. The supernatant (PRP) was carefully transferred to another tube, which was rinsed with 3.8% sodium citrate before transfer. The PPP was obtained from the remaining blood by centrifugation (2000 ×g, 10 min) at room temperature. The platelet was isolated from PRP by centrifugation (550 ×g, 15 min) at room temperature and resuspended with a HEPES-Tyrode buffer (NaCl 137 mM, NaHCO3 12 mM, NaH2PO4 0.4 mM, KCl 2.8 mM, HEPES 10 mM, glucose 5.5 mM, pH 7.4) containing 50 ng/mL of prostaglandin E1 (PGE1) and 1 mM of ethylene diamine tetra-acetic acid (EDTA). The material was centrifuged (550 ×g, 8 min) again at room temperature, and the supernatant was discarded. The washed platelets were resuspended in the HEPES-Tyrode buffer.
Reagents and antibodies
Isoliquiritigenin (961-29-5; the structure is listed in Figure 1A) was purchased from Weikeqi-biotech. Thrombin (T4393), ADP (A5285), PGE1 (P7527), fibrinogen (F3879), TRITC-Phalloidin (P1951), dimethyl sulfoxide (DMSO; D2650), bovine serum albumin (BSA; B2064), and Triton X-100 (X100) were purchased from Sigma-Aldrich. Collagen (LS001652) was purchased from Chrono-Log. Fluo 3-AM (F023-10) was purchased from Dojindo. Fluorescein-conjugated antibody to human CD62P (Clone: AK-4) and PAC-1 (Clone: PAC-1) were purchased from BD Biosciences. The antibodies for the immunoblot in this study were the following: anti-phospho-PLCγ2 (Tyr759) antibody (50535, CST), anti-phospho-ERK1/2 (Thr202/Tyr204) antibody (4370, CST), anti-ERK1/2 antibody (9194, CST), anti-phospho-Jun N-terminal kinase (JNK) (Thr183/Tyr185) antibody (sc-6254, Santa Cruz), anti-JNK antibody (sc-7345, Santa Cruz), anti-phospho-p38 MAPK (Thr180/Tyr182) antibody (4511, CST), anti-p38 MAPK antibody (8690, CST), anti-phospho-Akt (Ser473) antibody (4060, CST), anti-Akt antibody (sc-5298, Santa Cruz), and anti-GAPDH (ANT011, Antegene).
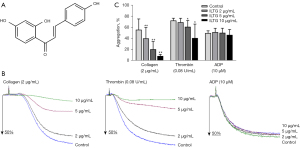
Platelet aggregation assay
Platelets were stimulated with collagen (2 µg/mL), thrombin (0.08 U/mL), or ADP (10 µM) to induce aggregation. The aggregation of platelets was evaluated using the light transmittance aggregometry method according to the manufacturer’s protocol of the Chrono-log Model 700 (CHRONO-LOG). Platelets were added to the reaction tube together with a rotor. CaCl2 was added to platelets to make a final concentration of 1 mM. The speed of the rotor was set at 1,000 rpm. Different concentrations of the ILTG solution were added to the reaction tube at 37 ℃ for 5 min, and the tube with DMSO was set as the control. Then, the tube was placed into the Chrono-log Model 700. The baseline was adjusted with PPP. An agonist was added to the reaction system to induce aggregation, and data were recorded. The light transmittance of PRP with no agonist was set to 0, and the light transmittance of PPP was set to 100%. A platelet aggregation curve was obtained to display the change in light transmittance after the agonist was added (23,24).
Platelet ATP release assay
The amount of ATP released from the activated platelets was detected using a Chrono-Log Lume Kit (CHRONO-LOG) according to the manufacturer’s protocol. Platelets were added to the reaction tube together with 1 mM of CaCl2. Different concentrations of ILTG solution were added to the reaction tube at 37 ℃ for 5 min, and the tube with DMSO was set as the control. Then, the tube was placed into the Chrono-log Model 700, and calibration was completed with Tyrode’s solution. The ATP Chrono-Lumen reagent was added to the reaction system at 37 ℃ for 60 s in the dark. Then, the agonist was added to the reaction tube, and data were recorded in the dark.
Flow cytometry
Washed platelets were separated from whole blood. The CaCl2, DMSO, or ILTG solution was added to the platelet solution and incubated at 37 ℃ for 5 min. The agonist was then added to the reaction system and incubated at 37 ℃ for 5 min. Next, fluorescein-conjugated antibodies or their isotypes were added to the reaction solution and incubated at 37 ℃ for 15 min in the dark. Finally, Tyrode’s solution was used to stop the reaction. All samples were acquired with fluorescence activated cell sorting (FACS) Aria II (Beckton Dickinson), and the data were analyzed using FlowJo v. 10 software (Tree Star).
Platelet extension assay
The coverslip was placed in a 24-well plate and incubated with 25 µg/mL of fibrinogen solution or 1% BSA at 4 ℃ overnight. The coverslip was washed with phosphate-buffered saline (PBS_3 times and blocked with 1% BSA at 37 ℃ for 1 h. Then, the platelets were washed, different solutions were added to the coverslips, and the platelets were incubated at 37 ℃ for 45 min. The supernatant was discarded, and platelets were fixed with 2% paraformaldehyde at 37 ℃ for 15 min. Platelets were permeabilized using 0.1% Tritton at 37 ℃ for 5 min. The supernatant was discarded and then stained with 1 µg/mL of TRITC-Phalloidin for 15 min in the dark. Slices were made, and data were recorded using a fluorescence microscope.
Immunoblot analysis
In the platelet aggregation assay, platelets were collected when they achieved a maximal aggregation ratio. Then, platelets were lysed in radio-immunoprecipitation assay (RIPA) lysis buffer (P0013, Beyotime Biotechnology) with proteases (Calbiochem) and phosphatase inhibitor (Calbiochem) for 25 min on ice. Whole-cell lysates were subjected to the immunoblot assay using standard procedures.
Statistical analysis
The data were analyzed with SPSS 21.0 (IBM Corp.). The paired t test was used for comparisons between two groups. For comparisons between multiple groups, 1-way analysis of variance (ANOVA) was used to generate P values. A P value less than 0.05 was considered statistically significant.
Results
ILTG inhibited platelet aggregation induced by collagen and thrombin
The interaction between adhesion receptors and collagen initiates the activation of platelets, and then the soluble agonist ADP and thrombin further promote the aggregation of platelets (25). We preincubated platelets with different concentrations of ILTG (2, 5, and 10 µg/mL) and observed the aggregation of platelets induced by collagen, thrombin, and ADP separately. The results showed that, compared with the control, ILTG could significantly inhibit collagen- and thrombin-induced platelet aggregation at concentrations of 5 and 10 µg/mL (Figure 1B,1C). However, ADP-induced platelet aggregation was not influenced by the pretreatment of ILTG (Figure 1B). The inhibitory effect of ILTG was more obvious in the collagen group. A relatively low level of ILTG (2 µg/mL) could significantly inhibit collagen-induced platelet aggregation. In contrast, in the thrombin group, only high doses of ILTG (5 and 10 µg/mL) exerted a significant antiplatelet effect (Figure 1C).
ILTG inhibited the release of α granules and dense granules
The activation of platelets led to the release of intracellular granules, including α granules, dense granules, and lysosomal granules (26,27). The dense granules contain ATP, and the secretion of dense granules can increase the level of ATP in the surroundings (2). We treated platelets with ILTG and stimulated them with collagen or thrombin, after which we detected the ATP in the solution. The results showed that ILTG could inhibit the secretion of dense granules induced by collagen and thrombin (Figure 2A,2B). P-selectin is located in α granules in platelets, and the secretion of α granules can increase the level of P-selectin on platelet membranes (28). We detected the P-selectin expression on platelets to evaluate the secretion of α granules. We found that ILTG could downregulate the expression of P-selectin significantly on platelets activated by thrombin (Figure 2C). ILTG also showed a tendency to inhibit P-release expression induced by collagen, but the effect was not significant.
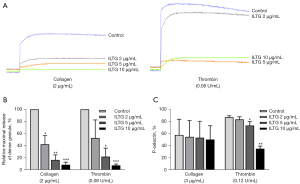
ILTG inhibited the signal transduction during platelet activation
The activation of platelets induces the conformational change of integrin αIIbβ3 and increases its binding affinity to ligands in a process is called inside-out signaling (29,30). Procaspase-activating compound 1 (PAC-1) is a marker of platelet activation, which can specifically bind to the high-affinity integrin αIIbβ3 receptor on activated platelets. We stimulated pretreated platelets with collagen or thrombin and then detected the level of PAC-1 on platelets. The results showed that ILTG could significantly inhibit the binding of PAC-1 on activated platelets (Figure 3A). The binding of integrin αIIbβ3 to its ligand initiates several outside-in signals, resulting in the adherence and extension of platelets (31). We detected the adherence and extension of platelets on the fibrinogen-coated surface by observing fluorescein-conjugated TRITC-Phalloidin-labeled actin in platelets under a fluorescence microscope. Our data indicated that ILTG could inhibit the extension of platelets on a fibrinogen-coated surface (Figure 3B). The number of adhered platelets and the extension area of platelets decreased significantly in the ILTG-treated group (Figure 3C,3D).
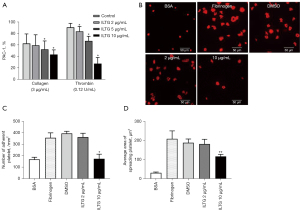
ILTG inhibited the glycoprotein VI-mediated signaling pathway
To investigate the underlying molecular mechanism of ILTG’s antiplatelet effect, platelets were pretreated with ILTG and activated by collagen. Then, we detected the phosphorylation levels of various molecules downstream of the collagen-glycoprotein VI (GPVI) pathway. ILTG could inhibit the phosphorylation of phospholipase C γ2 (PLCγ2) and protein kinase B (PKB/Akt) (Figure 4A,4B). The phosphorylation of extracellular signal–regulated kinase (ERK) was slightly inhibited by ILTG at higher concentrations (Figure 4C,4D). However, the phosphorylation of c-Jun N-terminal kinase (JNK) and p38 was not influenced by the pretreatment of ILTG (Figure 4C). Protein kinase C (PKC) substrates were also detected, and the results showed that their phosphorylation could be suppressed after ILTG treatment (Figure 4E). Overall, our study indicated that ILTG could inhibit the intracellular signals mediated by Akt, PKC, and MAPK pathway, thus decreasing the secretion of granules, suppressing the aggregation and extension of platelet, and exerting its antiplatelet effect (Figure 5).
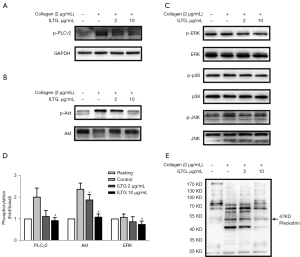
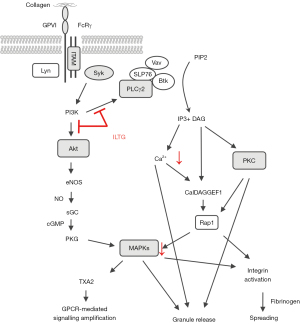
Discussion
In our study, we confirmed the antiplatelet activity of ILTG, including the inhibitory effect on platelet aggregation, granule secretion, and intracellular signal transduction. We used 3 agonists, namely, collagen, thrombin, and ADP, to activate platelets and found that ILTG could inhibit the aggregation of platelets stimulated with collagen and thrombin. ADP is stored in dense granules and can be released after platelet activation (32). ILTG may exert its suppressive effect on platelets when stimulated by collagen or thrombin through the blockage of ADP secretion, but this may have little influence on the ADP-mediated platelet aggregation. Activated platelets can release ATP to their surroundings, and P-selectin stored in α granules will be transported to the platelet membrane (33,34). Thus, the level of ATP in the platelet suspension and the expression of P-selectin on the platelet surface are good indicators of the secretory ability of activated platelets. We confirmed that ILTG could inhibit the secretion of granules by detecting the concentration of ATP and expression of P-selectin after adding an agonist to the platelet suspension.
When collagen activates the GPVI signal, Src family kinases such as Fyn and Lyn will bind to the ITAM motif of FcRγ. Then, Fyn and Lyn activate PLCγ2, phosphoinositide 3-kinase (PI3K) and MAPK via tyrosine kinase Syk (35). These intracellular signals can elevate the amount of Ca2+ in platelets, resulting in the secretion of granules, activation of integrin, and platelet aggregation (2). We extracted the protein from activated platelets and detected the expression of these signal mediators. We found that ILTG could inhibit the phosphorylation of PLCγ2 and Akt, suggesting ILTG had an inhibiting effect on the collagen-GPVI signaling pathway.
Thrombin and ADP can bind to protease-activated receptor and P2Y1 receptor and then activate PLCβ, PI3K/Akt, and MAPK via G protein Gq (36,37). Furthermore, thrombin can activate small G protein RhoA via guanine nucleotide exchanging factors, leading to platelet deformation and a secretory reaction (38). ADP can inhibit the activity of adenylyl cyclase (AC), reduce the synthesis of cyclic AMP (cAMP), and relieve the suppression effects on platelet (39). ILTG inhibited the activation of platelets when in the presence of collagen or thrombin, indicating some common molecules such as PLCβ and Akt might be targets of ILTG.
The inside-out signaling increases the binding affinity of the integrin αIIbβ3 receptor. Furthermore, the binding of activated integrin αIIbβ3 receptor and collagen initiates outside-in signaling (40-42). Src family kinases (SFK)-mediated signaling activates several downstream pathways, including the phosphorylation of the myosin heavy chain, the activation of RhoA GTPase activating protein, and the phosphorylation of PLCγ2 (43). We found that both inside-out signaling and outside-in signaling could be suppressed by ILTG, leading to dysfunction of the platelets.
Multiple in vivo studies have investigated the function of ILTG, but the dose of ILTG applied varied (44,45). Our in vitro study indicates that ILTG has great potential to be an antiplatelet drug because it can inhibit the formation of platelet plug and the extension of thrombus. However, further research is needed to evaluate the safety and efficacy of ILTG in vivo, including the use of animal models to investigate the pharmacodynamics, metabolic processes, and side effects of ILTG. Whether ILTG can be used in patients still needs to be verified by clinical trials. However, ILTG might have applications in cancer treatment. Cancer cells can induce the activation of platelets by direct connection or by releasing an agonist. Activated platelets participate in cancer progression, angiogenesis, and tumor invasion (44). The antiplatelet function of ILTG might contribute to its anticancer effect. In conclusion, more studies are need to clarify the antiplatelet mechanism of ILTG and evaluate its potential in clinical application.
Acknowledgments
Funding: This research was supported by funds from the Key Special Project of Ministry of Science and Technology of China (No. 2020YFC0845700), Scientific Research Projects of the Chinese Academy of Engineering of China (No. 2020-XY-70 [2020-KYGG-01-07]), and Fundamental Research Funds for the Central Universities of China (No. 2020kfyXGYJ029).
Footnote
Reporting Checklist: The authors have completed the MDAR reporting checklist. Available at https://atm.amegroups.com/article/view/10.21037/atm-22-2839/rc
Data Sharing Statement: Available at https://atm.amegroups.com/article/view/10.21037/atm-22-2839/dss
Conflicts of Interest: All authors have completed the ICMJE uniform disclosure form (available at https://atm.amegroups.com/article/view/10.21037/atm-22-2839/coif). The authors have no conflicts of interest to declare.
Ethical Statement:
Open Access Statement: This is an Open Access article distributed in accordance with the Creative Commons Attribution-NonCommercial-NoDerivs 4.0 International License (CC BY-NC-ND 4.0), which permits the non-commercial replication and distribution of the article with the strict proviso that no changes or edits are made and the original work is properly cited (including links to both the formal publication through the relevant DOI and the license). See: https://creativecommons.org/licenses/by-nc-nd/4.0/.
References
- Machlus KR, Thon JN, Italiano JE Jr. Interpreting the developmental dance of the megakaryocyte: a review of the cellular and molecular processes mediating platelet formation. Br J Haematol 2014;165:227-36. [Crossref] [PubMed]
- van der Meijden PEJ, Heemskerk JWM. Platelet biology and functions: new concepts and clinical perspectives. Nat Rev Cardiol 2019;16:166-79. [Crossref] [PubMed]
- Holinstat M. Normal platelet function. Cancer Metastasis Rev 2017;36:195-8. [Crossref] [PubMed]
- Jenne CN, Kubes P. Platelets in inflammation and infection. Platelets 2015;26:286-92. [Crossref] [PubMed]
- Langer HF, Choi EY, Zhou H, et al. Platelets contribute to the pathogenesis of experimental autoimmune encephalomyelitis. Circ Res 2012;110:1202-10. [Crossref] [PubMed]
- Yeaman MR, Bayer AS, Koo SP, et al. Platelet microbicidal proteins and neutrophil defensin disrupt the Staphylococcus aureus cytoplasmic membrane by distinct mechanisms of action. J Clin Invest 1998;101:178-87. [Crossref] [PubMed]
- Wong CH, Jenne CN, Petri B, et al. Nucleation of platelets with blood-borne pathogens on Kupffer cells precedes other innate immunity and contributes to bacterial clearance. Nat Immunol 2013;14:785-92. [Crossref] [PubMed]
- Denis MM, Tolley ND, Bunting M, et al. Escaping the nuclear confines: signal-dependent pre-mRNA splicing in anucleate platelets. Cell 2005;122:379-91. [Crossref] [PubMed]
- Henn V, Slupsky JR, Gräfe M, et al. CD40 ligand on activated platelets triggers an inflammatory reaction of endothelial cells. Nature 1998;391:591-4. [Crossref] [PubMed]
- Patrono C, Morais J, Baigent C, et al. Antiplatelet Agents for the Treatment and Prevention of Coronary Atherothrombosis. J Am Coll Cardiol 2017;70:1760-76. [Crossref] [PubMed]
- Halvorsen S, Andreotti F, ten Berg JM, et al. Aspirin therapy in primary cardiovascular disease prevention: a position paper of the European Society of Cardiology working group on thrombosis. J Am Coll Cardiol 2014;64:319-27. [Crossref] [PubMed]
- McFadyen JD, Schaff M, Peter K. Current and future antiplatelet therapies: emphasis on preserving haemostasis. Nat Rev Cardiol 2018;15:181-91. [Crossref] [PubMed]
- Laine L. Review article: gastrointestinal bleeding with low-dose aspirin - what's the risk? Aliment Pharmacol Ther 2006;24:897-908. [Crossref] [PubMed]
- Zhang Z, Yung KK, Ko JK. Therapeutic Intervention in Cancer by Isoliquiritigenin from Licorice: A Natural Antioxidant and Redox Regulator. Antioxidants (Basel) 2022;11:1349. [Crossref] [PubMed]
- Wang Z, Xu G, Li Z, et al. NLRP3 Inflammasome Pharmacological Inhibitors in Glycyrrhiza for NLRP3-Driven Diseases Treatment: Extinguishing the Fire of Inflammation. J Inflamm Res 2022;15:409-22. [Crossref] [PubMed]
- Rui-Zhi T, Ke-Huan X, Yuan L, et al. Renoprotective effect of isoliquiritigenin on cisplatin-induced acute kidney injury through inhibition of FPR2 in macrophage. J Pharmacol Sci 2022;148:56-64. [Crossref] [PubMed]
- Funakoshi-Tago M, Ohsawa K, Ishikawa T, et al. Inhibitory effects of flavonoids extracted from Nepalese propolis on the LPS signaling pathway. Int Immunopharmacol 2016;40:550-60. [Crossref] [PubMed]
- Ay C, Dunkler D, Marosi C, et al. Prediction of venous thromboembolism in cancer patients. Blood 2010;116:5377-82. [Crossref] [PubMed]
- Mackman N. Triggers, targets and treatments for thrombosis. Nature 2008;451:914-8. [Crossref] [PubMed]
- Sun J, Zhang Q, Yang G, et al. The licorice flavonoid isoliquiritigenin attenuates Mycobacterium tuberculosis-induced inflammation through Notch1/NF-κB and MAPK signaling pathways. J Ethnopharmacol 2022;294:115368. [Crossref] [PubMed]
- Yang J, Hu F, Guo C, et al. Discovery of isoliquiritigenin analogues that reverse acute hepatitis by inhibiting macrophage polarization. Bioorg Chem 2021;114:105043. [Crossref] [PubMed]
- Tawata M, Aida K, Noguchi T, et al. Anti-platelet action of isoliquiritigenin, an aldose reductase inhibitor in licorice. Eur J Pharmacol 1992;212:87-92. [Crossref] [PubMed]
- Clark SR, Ma AC, Tavener SA, et al. Platelet TLR4 activates neutrophil extracellular traps to ensnare bacteria in septic blood. Nat Med 2007;13:463-9. [Crossref] [PubMed]
- Maugeri N, Campana L, Gavina M, et al. Activated platelets present high mobility group box 1 to neutrophils, inducing autophagy and promoting the extrusion of neutrophil extracellular traps. J Thromb Haemost 2014;12:2074-88. [Crossref] [PubMed]
- Mattheij NJ, Swieringa F, Mastenbroek TG, et al. Coated platelets function in platelet-dependent fibrin formation via integrin αIIbβ3 and transglutaminase factor XIII. Haematologica 2016;101:427-36. [Crossref] [PubMed]
- Joshi S, Whiteheart SW. The nuts and bolts of the platelet release reaction. Platelets 2017;28:129-37. [Crossref] [PubMed]
- Golebiewska EM, Poole AW. Platelet secretion: From haemostasis to wound healing and beyond. Blood Rev 2015;29:153-62. [Crossref] [PubMed]
- Rendu F, Brohard-Bohn B. The platelet release reaction: granules' constituents, secretion and functions. Platelets 2001;12:261-73. [Crossref] [PubMed]
- Shattil SJ, Kim C, Ginsberg MH. The final steps of integrin activation: the end game. Nat Rev Mol Cell Biol 2010;11:288-300. [Crossref] [PubMed]
- Payrastre B, Missy K, Trumel C, et al. The integrin alpha IIb/beta 3 in human platelet signal transduction. Biochem Pharmacol 2000;60:1069-74. [Crossref] [PubMed]
- Shattil SJ, Newman PJ. Integrins: dynamic scaffolds for adhesion and signaling in platelets. Blood 2004;104:1606-15. [Crossref] [PubMed]
- Golebiewska EM, Harper MT, Williams CM, et al. Syntaxin 8 regulates platelet dense granule secretion, aggregation, and thrombus stability. J Biol Chem 2015;290:1536-45. [Crossref] [PubMed]
- Han P, Hanlon D, Arshad N, et al. Platelet P-selectin initiates cross-presentation and dendritic cell differentiation in blood monocytes. Sci Adv 2020;6:eaaz1580.
- Panicker SR, Mehta-D'souza P, Zhang N, et al. Circulating soluble P-selectin must dimerize to promote inflammation and coagulation in mice. Blood 2017;130:181-91. [Crossref] [PubMed]
- Semeniak D, Kulawig R, Stegner D, et al. Proplatelet formation is selectively inhibited by collagen type I through Syk-independent GPVI signaling. J Cell Sci 2016;129:3473-84. [PubMed]
- Canobbio I, Cipolla L, Consonni A, et al. Impaired thrombin-induced platelet activation and thrombus formation in mice lacking the Ca(2+)-dependent tyrosine kinase Pyk2. Blood 2013;121:648-57. [Crossref] [PubMed]
- Sebastiano M, Momi S, Falcinelli E, et al. A novel mechanism regulating human platelet activation by MMP-2-mediated PAR1 biased signaling. Blood 2017;129:883-95. [Crossref] [PubMed]
- Qian F, Le Breton GC, Chen J, et al. Role for the guanine nucleotide exchange factor phosphatidylinositol-3,4,5-trisphosphate-dependent rac exchanger 1 in platelet secretion and aggregation. Arterioscler Thromb Vasc Biol 2012;32:768-77. [Crossref] [PubMed]
- Clark JC, Kavanagh DM, Watson S, et al. Adenosine and Forskolin Inhibit Platelet Aggregation by Collagen but not the Proximal Signalling Events. Thromb Haemost 2019;119:1124-37. [Crossref] [PubMed]
- Huang J, Li X, Shi X, et al. Platelet integrin αIIbβ3: signal transduction, regulation, and its therapeutic targeting. J Hematol Oncol 2019;12:26. [Crossref] [PubMed]
- Durrant TN, van den Bosch MT, Hers I. Integrin αIIbβ3 outside-in signaling. Blood 2017;130:1607-19. [Crossref] [PubMed]
- Stefanini L, Bergmeier W. RAP GTPases and platelet integrin signaling. Platelets 2019;30:41-7. [Crossref] [PubMed]
- Zhu J. Csk/CD148 and platelet SFK activation: a balancing act! Blood 2018;131:1042-3. [Crossref] [PubMed]
- Wang KL, Yu YC, Hsia SM. Perspectives on the Role of Isoliquiritigenin in Cancer. Cancers (Basel) 2021;13:115. [Crossref] [PubMed]
- Liu JQ, Zhao XT, Qin FY, et al. Isoliquiritigenin mitigates oxidative damage after subarachnoid hemorrhage in vivo and in vitro by regulating Nrf2-dependent Signaling Pathway via Targeting of SIRT1. Phytomedicine 2022;105:154262. [Crossref] [PubMed]
(English Language Editors: C. Mullens and J. Gray)