Dexmedetomidine ameliorates ischemia-induced nerve injury by up-regulating Sox11 expression
Highlight box
Key findings
• SRY-box transcription factor 11 (Sox11) mediated the effects of dexmedetomidine (Dex) on neuron protection with the oxygen-glucose deprivation/reoxygenation (OGD/R) condition.
What is known and what is new?
• Dex is vital for cell survival and apoptosis, especially in the neuron system.
• However, whether Dex was associated with neuron injury and stroke development is still unclear. Thus, we aimed to explore whether Dex ameliorated ischemia-induced injury and determine its mechanism.
What is the implication, and what should change now?
• The role of Dex in cell viability and survival was verified. Moreover, Dex protected neurons from middle cerebral artery occlusion (MCAO)-induced injury by up-regulating Sox11 expression. Our research proposes a potential drug to improve the functional recovery of stroke patients in the clinic. However, the mechanism of Dex up-regulation of Sox11 remains unclear, and thus, future studies should focus on this problem. Moreover, the influence of Dex on neuronal function via several other targets should also be explored in the future.
Introduction
Ischemic stroke causes high levels of mortality and disability worldwide (1), with many stroke patients requiring different levels of long-term assistance, which leads to a significant economic burden (2). Therefore, paying attention to this severe public health problem and the outcome of stroke is crucial. However, few studies have focused on novel therapeutic targets for the prevention and recovery from this devastating disease.
Several drugs have been reported to protect the brain from ischemia-induced injury (3-6). Sodium butyrate was reported to prevent middle cerebral artery occlusion (MCAO)-induced cell apoptosis by activating GPR41/Gβγ/PI3K/Akt signaling, which could be transformed in the clinic (7). Vitexin can suppress neuronal autophagy and prevent MCAO-induced cell injury by activating mechanistic target of rapamycin kinase (mTOR) signaling (6). Furthermore, it has been confirmed that berberine also improves brain injury following ischemia/reperfusion treatment by activating BDNF-TrkB-PI3K/Akt signaling (8). microRNA-153 was also associated with brain injury through the activation of sonic hedgehog signaling molecule (Shh) signaling and angiogenesis, as was reported previously (9). However, it is currently difficult to improve the functional recovery of stroke patients. Therefore, more clinical targets and potential drugs should be explored to improve the prognosis of these patients.
Dexmedetomidine (Dex) is associated with several biological processes; it can inhibit the inflammation of the neuronal system by regulating the transformation of M1/M2 polarization via activation of the mitogen-activated protein kinase kinase/mitogen-activated protein kinase (MEK/ERK) signaling pathway, which might protect neurons from injury (10). Dex was also found to improve cognitive function after surgery compared with the placebo group in an animal model, suggesting that it might protect neurons from injury (11). Moreover, it also significantly inhibited reactive oxygen species (ROS) production and suppressed the Cx43/PKC-α/NOX2/ROS pathway to improve lipopolysaccharide (LPS)-induced inflammation and prevent cell apoptosis (12). In a rat model, Dex was also shown to decrease the apoptosis ratio of cardiomyocytes induced by hydrogen peroxide (H2O2) (13). Furthermore, it might also be correlated with the development of cancer by influencing the hypoxia inducible factor (HIF) signaling and the PI3K/Akt pathway (14,15). Also, Dexmedetomidine is a promising agent for off-label use in palliative care (16). Therefore, it seems that Dex plays a vital role in cell survival and apoptosis, especially in the neuronal system. However, whether it is associated with neuronal injury and stroke development remains unclear.
Herein, we confirmed the role of Dex in cell viability and survival. The same results were also observed in the in vivo model. Study has shown that Sox11 increases reactivity in peripheral nerve injury with strong regenerative capacity, and played an important role in regulating neuron survival, proliferation and axon growth in the peripheral nervous system (17). And the expression of Sox11 could be modulated by the cell-type specific machinery in transient forebrain ischemia in the rat (18). Additionally, we also observed that Dex protected neurons from MCAO-induced injury by up-regulating SRY-box transcription factor 11 (Sox11) expression. Our research proposes a potential drug to improve the functional recovery of stroke patients in the clinic. We present the following article in accordance with the MDAR and ARRIVE reporting checklists (available at https://atm.amegroups.com/article/view/10.21037/atm-22-6639/rc).
Methods
MCAO
The MCAO procedure was conducted as previously described (19,20). Sprague-Dawley rats (female, 8 weeks old, 200–220 g) were obtained from the Model Animal Research Center of Nanjing University, China. The rats were placed in an anesthetic chamber and anesthetized with 3% isoflurane coupled with 30% oxygen and 70% nitrous oxide; 1.5% isoflurane was administered for anesthesia maintenance using a facemask. A 6-0 nylon filament coated with silicone rubber (Doccol, Sharon, MA, USA) was then introduced into the external carotid artery. The filament was pushed approximately 9–10 mm along the internal carotid artery to the bifurcation and origin of the middle cerebral artery (MCA) and was kept in place for 1 h.
Dex (Merck, No. 1179333, 100 µmol/kg) was then administered via the tail vein and blood flow to the MCA was restored for 24 h by removal of the filament (21). Control rats received the same surgical procedure but without MCAO. The animals were housed in a temperature-controlled (22±3 ℃) and humidified (60%±5%) environment with a 12-h light/dark cycle. Each group contained eight rats. This animal experiment was approved by the Institutional Animal Ethics Committee of Guangzhou Women and Children’s Medical Center (No. S2022-107), in compliance with institutional guidelines for the care and use of animals. A protocol was prepared before the study without registration.
Cell culture
SK-N-SH and SH-SY5Y cells were obtained from the Shanghai Institute of Biochemistry and Cell Biology, Chinese Academy of Sciences (Shanghai, China) and grown in Dulbecco’s modified Eagle medium (DMEM, Biosharp, BL308A, Beijing, China) containing 10% fetal bovine serum (FBS), 100 IU/mL penicillin, and 100 µg/mL streptomycin in a 37 ℃/5% CO2 humidified incubator.
Oxygen-glucose deprivation/reoxygenation (OGD/R) model
The cells were rinsed twice with cold phosphate buffer saline (PBS) and grown in a hypoxic environment (1% O2, 95% N2, and 5% CO2) in oxygen-glucose deprivation (OGD) medium (glucose-free and serum-free DMEM) for 8 h at 37 ℃. Thereafter, they were rapidly reoxygenated in 95% air and 5% CO2. The cells were then transferred to a standard medium for 24 h.
NA extraction and quantitation
Total RNA was extracted from tissues or cells using a Total RNA Extraction Kit (#17200; AmyJet Scientific, Wuhan, China) according to the provided protocol. RNA concentrations were measured in a Nanodrop 2000 spectrophotometer (Thermo Fisher Scientific). Complementary DNA (cDNA) was synthesized from approximately 1 µg of the total RNA using an Omniscript RT Kit (#205111; Qiagen) followed by quantitative real-time polymerase chain reaction (qRT-PCR) amplification using a TransStart® Green qPCR SuperMix kit (#AQ101-01; TransGen Biotech, Beijing, China). The standard 2−ΔΔCt method was used to calculate the mRNA expression from a minimum of three biological replicates. The primer sequences are listed in Table 1.
Table 1
Gene name | Primer sequence (5'-3') | Length |
---|---|---|
Human-Sox11 | F: CGGTCAAGTGCGTGTTTCTG | 179 bp |
R: CACTTTGGCGACGTTGTAGC | ||
Human-GAPDH | F: CTCTGCTCCTCCTGTTCGAC | 167 bp |
R: ATGGTGTCTGAGCGATGTGG | ||
Rat-Sox11 | F: TCGTGATTGCAACAAAGGCG | 132 bp |
R: TTGTGCCGTCTAAAGGGTCC | ||
Rat-GAPDH | F: AGAGACAGCCGCATCTTCTT | 101 bp |
R: GGTAACCAGGCGTCCGATAC |
qRT-PCR, quantitative real-time polymerase chain reaction; F, forward primer; R, reverse primer.
Western blotting
Total protein was isolated from tissue samples and SK-N-SH and SH-SY5Y cells using radio immunoprecipitation assay (RIPA) buffer, followed by the measurement of protein concentrations with a bicinchoninic acid assay (BCA) kit (Beyotime, Shanghai, China). The protein sample was first electrophoresed for 2 h before being transferred to polyvinylidene fluoride (PVDF) membranes (Millipore, Billerica, MA, USA). TBST with 5% fat-free milk was used to block the non-specific antigens for 1 hour before incubating with primary antibodies [Sox11 (Abcam, ab234996, 1:2,000), GAPDH (Abcam, ab9485, 1:5,000), BAX (Abcam, ab32503, 1:5,000), BIM (Abcam, ab32158, 1:1,500), MCL-1 (Abcam, ab32087, 1:3,000), and Bcl-2 (Abcam, ab196495, 1:2,000)] and secondary antibodies. The proteins were visualized by enhanced chemiluminescence (ECL) and analyzed using ImageJ (NIH, Bethesda, MD, USA).
Cell viability
Cell viability was assessed by Cell Counting Kit-8 (CCK-8) assays. About 1,500 cells were inoculated in 96-well plates. Dex (1, 10, and 50 µM) was added into wells after 1 day (22). The absorbance was assessed after 2 days using CCK-8 (Dojindo, Japan).
Apoptosis assay
For the apoptosis assay, SK-N-SH and SH-SY5Y cells in six-well plates were incubated with Dex (1, 10, and 50 µM) in the indicated groups for 48 hours. This was followed by incubation with Annexin V-fluorescein isothiocyanate (FITC) and Prodium Iodide (PI) (KeyGEN BioTECH, China) for about 10 mins (as indicated by the instructions) and detection using CytExpert.
5-ethynyl-2'- deoxyuridine (EdU) assay
Cellular proliferation was measured with an EdU assay kit (Ribobio, Guangzhou, China). The cells were digested and inoculated into six-well plates at 10×105 per well. Dex (1, 10, and 50 µM) was added into the wells 1 day later. Then, 50 µM of EdU buffer was added into the wells and incubated at 37 ℃ for 2 h as indicated by the instructions. After fixation with 4% formaldehyde for 30 min and permeabilization with 0.1% Triton X-100 for 10 min, the cells were incubated with an EdU solution, and the nuclei were then stained with Hoechst. The cells were examined and imaged by fluorescence microscopy.
Plasmid transfection
Short hairpin RNA (shRNA) for Sox11 knockdown and Sox11 overexpression plasmids pcDNA4.0-Sox11 were obtained from Synbio Technologies (Suzhou, China). The cells were transfected with plasmids expressing shSox11 and cDNA for Sox11 using Lipofectamine 2000 (Life Technologies) for 72 h, as indicated by the instructions. The transfected cells were then selected using 2 mg/mL of puromycin (Gibco; California, USA) for several days.
Assessment of neurological deficits
Neurological deficits were examined using the Longa and Bederson scores. The rats were evaluated 24 h after awakening from anesthesia and were scored as follows: 0 points, no symptoms of damage; 1 point, unable to extend contralateral forepaw completely; 2 points, circling to the opposite side; 3 points, collapsing to the opposite side; 4 points, unable to walk spontaneously; and 5 points, unconscious.
Statistical analysis
All experiments were performed three times and all of the data were expressed as means ± standard deviation (SD). Analysis was performed using SPSS 25.0 (IBM Corp., NY, USA) and graphics were constructed using GraphPad Prism 8.0 (La Jolla, CA, USA). T-tests were used to evaluate differences between groups, and multiple group comparisons were analyzed with one-way ANOVA, and P values <0.05 were considered significant.
Results
Dex positively regulates the expression of Sox11 under OGD/R conditions
Given that Sox11 is indispensable for neuronal survival, neurite outgrowth, axon regeneration, and neuron fate diversity (23-25), we first verified whether DEX treatment influenced Sox11 expression. As expected, the OGD/R conditions markedly reduced both the mRNA and protein levels of Sox11 (Figure 1A-1C). The cells were then incubated with varying concentrations of Dex (1, 10, and 50 µM). As shown in Figure 1A-1C, Dex treatment significantly elevated the levels of SOX in cells under OGD/R conditions (Figure 1A-1C). Thus, we demonstrated Dex positively regulates Sox11 expression under OGD/R conditions.
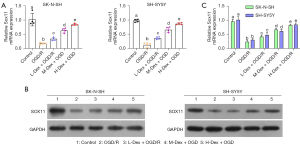
Dex protects cells from OGD/R-induced damage
Next, we examined the influence of Dex on cell survival. As expected, the OGD/R conditions notably inhibited cell viability, as indicated by the CCK-8 assay. However, Dex dose-dependently ameliorated the OGD/R-induced effects on cell survival (Figure 2A). As depicted by the EdU assay, the proliferation of SK-N-SH and SH-SY5Y cells was markedly reduced after OGD/R (Figure 2B,2C), while Dex treatment counteracted these effects and enhanced cell proliferation. Similarly, OGD/R significantly induced apoptosis, which was dose-dependently reversed by Dex treatment (Figure 3A,3B).
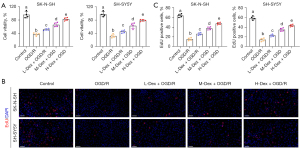
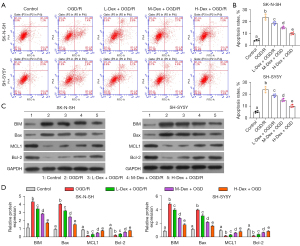
We then evaluated the apoptosis-associated proteins in each group. BIM and Bax were notably increased by OGD/R treatment. Furthermore, the different concentrations of Dex decreased BIM and Bax levels compared to OGD/R-only treatment (Figure 3C,3D). Moreover, MCL1 and Bcl-2 levels were notably reduced by OGD/R treatment, and were increased by the different concentrations of Dex, as compared to the OGD/R group (Figure 3C,3D). Taken together, these results confirmed that Dex prevented OGD/R-induced injury.
Sox11 rescues OGD/R-induced cell damage
To determine the function of Sox11 in cell protection, we overexpressed Sox11 in OGD/R-treated cells, and the efficacy of Sox11 overexpression was confirmed (Figure 4A-4C). We then evaluated cell viability and proliferation. OGD/R treatment notably inhibited cell viability. However, Sox11 overexpression counteracted the effects of OGD/R on cell survival (Figure 4D). The EdU assay indicated that cell proliferation was significantly inhibited with OGD/R. Meanwhile, Sox11 overexpression promoted cell proliferation under OGD/R conditions (Figure 4E and Figure S1).
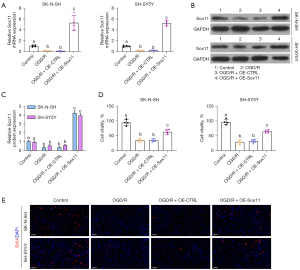
Cell apoptosis was also detected by the apoptosis assay. Similarly, OGD/R significantly induced cell apoptosis, while Sox11 overexpression notably rescued the apoptosis ratio under OGD/R conditions (Figure 5A,5B). We then evaluated apoptosis-associated proteins in each group. As expected, BIM and Bax were notably increased by OGD/R treatment, and were decreased by Sox11 overexpression, as compared to the OGD/R group (Figure 5C,5D). Additionally, the expressions of MCL1 and Bcl-2 were notably decreased by OGD/R treatment, and were increased by Sox11 overexpression, as compared to the OGD/R group (Figure 5C,5D). Therefore, Sox11 rescues cells from OGD/R-induced damage.
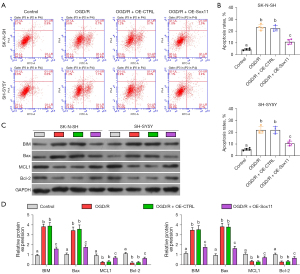
Dex prevents OGD/R-mediated damage by up-regulating Sox11
To verify whether Dex reduced OGD/R-mediated damage via the promotion of Sox11 expression, Sox11 was knocked down in the OGD/R-pretreated cells, which were also treated with Dex. The efficacy of Sox11 knockdown was also confirmed (Figure 6A). We then evaluated cell viability, proliferation, and apoptosis. Dex treatment notably promoted cell viability under OGD/R conditions. However, Sox11 knockdown rescued the effects of Dex on cell survival (Figure 6B). The EdU assay indicated that cell proliferation was notably promoted with Dex treatment. Meanwhile, Sox11 knockdown inhibited cell proliferation with Dex and OGD/R treatment (Figure 6C). Similarly, Dex also significantly decreased cell apoptosis. Moreover, Sox11 knockdown notably rescued the apoptosis ratio under Dex treatment (Figure 6D).
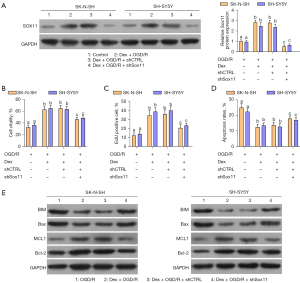
We then evaluated apoptosis-associated proteins in each group. As expected, BIM and Bax were notably decreased by Dex treatment. Furthermore, Sox11 knockdown increased BIM and Bax expression comparing with Dex treatment in OGD/R. In addition, MCL1 and Bcl-2 expression were notably up-regulated by Dex treatment. Sox11 knockdown reduced the levels of MCL1 and Bcl-2 compared to those in the Dex group (Figure 6E). These results indicate that Dex improved OGD/R-induced cell damage by up-regulating Sox11.
Dex protected rats from ischemia-induced injury in the MCAO model
We also explored the effects of Dex in vivo. As expected, MCAO significantly induced ischemia and injury to the rat brains, as shown by a larger infarct area and higher Bederson and Longa behavior scores (Figure 7A-7D). However, Dex treatment notably protected rat from ischemia-induced injury compared with the MCAO group (Figure 7A-7D). The apoptosis-associated proteins were also detected. The expressions of BIM and Bax were markedly increased in the MCAO group. Furthermore, Dex decreased the BIM and Bax levels compared to those in the MCAO group (Figure 7E). Additionally, MCL1 and Bcl-2 levels were notably reduced in the MCAO group, and were increased by Dex treatment, as compared to the MCAO group (Figure 7E). Together, these results highlight that Dex prevented ischemia-induced injury in the MCAO model.
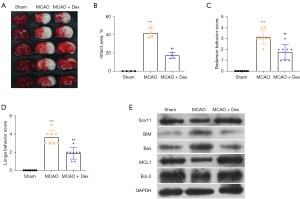
Discussion
Ischemic stroke leads to high levels of both mortality and disability worldwide (1). When acute ischemic reperfusion occurs, neurons are damaged by inflammation. However, anti-inflammation therapies were not transformed in the clinic (26). In this study, we confirmed that Dex treatment enhanced cell viability and proliferation and reduced apoptosis. The same results were also observed in the in vivo model. Additionally, we also observed that Dex protected neurons from MCAO-induced injury by up-regulating Sox11 expression.
Ischemic stroke can cause neuronal apoptosis (27-30). Therefore, anti-apoptosis treatment could be a promising method of improving stroke-induced damage. Using a rat MCAO model, a previous study proved that delayed recanalization efficiently protected neurons from apoptosis mediated by HGF/c-Met/STAT3/Bcl-2 signaling (31). Long OPA1 mitochondrial dynamin like GTPase (L-OPA1) restoration also suppresses neuronal apoptosis induced by ischemic stroke by regulating mitochondrial function (32). It was reported that γ-glutamylcysteine suppressed neuronal apoptosis by decreasing ROS production as well as glutathione balance (33). Herein, we found that Dex significantly inhibited neuronal apoptosis and promoted cell survival and proliferation. These results are meaningful for patients suffering from stroke and neuronal damage.
As previously reported, Dex has several targets (34-36). Studies have confirmed that Dex regulates c-Fos/NLRP3/caspase-1 cascades, ERK signaling, AKT signaling, and the miR-330-3p/ULK1 axis (34-37). It can also regulate several miRNAs to modulate cell apoptosis, drug resistance, and cancer metastasis (38). In adipogenesis, Dex directly up-regulates miR-155 and promotes the differentiation of 3T3-L1 preadipocytes (39). FOXO1 is another target of Dex in MC3T3-E1 cells, which was identified by bioinformatics analysis (40). In our study, we identified that Sox11 is a new target of Dex; Dex positively regulated Sox11 in neuronal cells to maintain cell survival and inhibit apoptosis.
Sox11 is vital for cell survival. It has been reported to regulate neuronal survival, is a key regulator of sensory neuron development (41), and is also a regulator of cancer stem cells (42). Sox11 overexpression also improves angiotensin II receptor type 2 (AT2) mechanical injury and could represent a target for treating ventilatorinduced lung injury (43). A previous study showed that Sox11 overexpression augmented the excitation of dentate gyrus (DG) granule cells and reduced the levels of various potassium channel subunits, suggesting that Sox11 activity is vital for modulating the plasticity of DG neurons (44). Our results indicated that Sox11 was important for neuronal survival under OGD/R conditions and protected against stroke-induced injury. Furthermore, Sox11 mediated the neuronal protective effects of Dex under OGD/R conditions.
Our study had several limitations that should be noted. Firstly, the mechanism of Dex up-regulation of Sox11 is still unclear, and thus, future studies should focus on elucidating this mechanism. Secondly, Dex might influence neuronal function via several other targets, and we will explore this in the future. Then, the mechanism we clarified in the study was should be confirmed in the rat model. Finally, ischemic reperfusion also could induce inflammation, whether Dex could inhibit the inflammation of the neuronal system, and the effect of Dex on the cytokines expression need to be further confirmed.
Conclusions
In this study, we verified the role of Dex in cell viability and survival. Moreover, Dex protected neurons from MCAO-induced injury by up-regulating Sox11 expression. Our research proposed a potential drug to improve the functional recovery of stroke patients in the clinic.
Acknowledgments
Funding: None.
Footnote
Reporting Checklist: The authors have completed the MDAR and ARRIVE reporting checklists. Available at https://atm.amegroups.com/article/view/10.21037/atm-22-6639/rc
Data Sharing Statement: Available at https://atm.amegroups.com/article/view/10.21037/atm-22-6639/dss
Conflicts of Interest: All authors have completed the ICMJE uniform disclosure form (available at https://atm.amegroups.com/article/view/10.21037/atm-22-6639/coif). The authors have no conflicts of interest to declare.
Ethical Statement: The authors are accountable for all aspects of the work, including ensuring that any questions related to the accuracy or integrity of any part of the work have been appropriately investigated and resolved. This animal experiment was approved by the Institutional Animal Ethics Committee of Guangzhou Women and Children’s Medical Center (No. S2022-107), in compliance with institutional guidelines for the care and use of animals.
Open Access Statement: This is an Open Access article distributed in accordance with the Creative Commons Attribution-NonCommercial-NoDerivs 4.0 International License (CC BY-NC-ND 4.0), which permits the non-commercial replication and distribution of the article with the strict proviso that no changes or edits are made and the original work is properly cited (including links to both the formal publication through the relevant DOI and the license). See: https://creativecommons.org/licenses/by-nc-nd/4.0/.
References
- Volný O, Kašičková L, Coufalová D, et al. microRNAs in Cerebrovascular Disease. Adv Exp Med Biol 2015;888:155-95. [Crossref] [PubMed]
- Feigin VL, Nguyen G, Cercy K, et al. Global, Regional, and Country-Specific Lifetime Risks of Stroke, 1990 and 2016. N Engl J Med 2018;379:2429-37. [Crossref] [PubMed]
- Gu Z, Li Y, Zhang L, et al. Foxp3 attenuates cerebral ischemia/reperfusion injury through microRNA-150-5p-modified NCS1. Exp Cell Res 2021; Epub ahead of print. [Crossref] [PubMed]
- Mostafavi H, Amoli N, Ghasemloo E, et al. Up regulation of miR-149-5p reduces the consequences of cerebral ischemia in MCAO rats by modulating cation-chloride co-transporters expressions. Iran Biomed J 2022;26:357-65. [Crossref] [PubMed]
- Yu J, Li X, Matei N, et al. Ezetimibe, a NPC1L1 inhibitor, attenuates neuronal apoptosis through AMPK dependent autophagy activation after MCAO in rats. Exp Neurol 2018;307:12-23. [Crossref] [PubMed]
- Jiang J, Dai J, Cui H. Vitexin reverses the autophagy dysfunction to attenuate MCAO-induced cerebral ischemic stroke via mTOR/Ulk1 pathway. Biomed Pharmacother 2018;99:583-90. [Crossref] [PubMed]
- Zhou Z, Xu N, Matei N, et al. Sodium butyrate attenuated neuronal apoptosis via GPR41/Gβγ/PI3K/Akt pathway after MCAO in rats. J Cereb Blood Flow Metab 2021;41:267-81. [Crossref] [PubMed]
- Yang J, Yan H, Li S, et al. Berberine Ameliorates MCAO Induced Cerebral Ischemia/Reperfusion Injury via Activation of the BDNF-TrkB-PI3K/Akt Signaling Pathway. Neurochem Res 2018;43:702-10. [Crossref] [PubMed]
- Wang BX, Xu JJ, Hu J, et al. Effects of miR-153 on angiogenesis in MCAO rats through Shh signaling pathway. Eur Rev Med Pharmacol Sci 2019;23:732-9. [PubMed]
- Qiu Z, Lu P, Wang K, et al. Dexmedetomidine Inhibits Neuroinflammation by Altering Microglial M1/M2 Polarization Through MAPK/ERK Pathway. Neurochem Res 2020;45:345-53. [Crossref] [PubMed]
- Carr ZJ, Cios TJ, Potter KF, et al. Does Dexmedetomidine Ameliorate Postoperative Cognitive Dysfunction? A Brief Review of the Recent Literature. Curr Neurol Neurosci Rep 2018;18:64. [Crossref] [PubMed]
- Chai Y, Cao Z, Yu R, et al. Dexmedetomidine Attenuates LPS-Induced Monocyte-Endothelial Adherence via Inhibiting Cx43/PKC-α/NOX2/ROS Signaling Pathway in Monocytes. Oxid Med Cell Longev 2020;2020:2930463. [Crossref] [PubMed]
- Weng X, Shi W, Zhang X, et al. Dexmedetomidine attenuates H(2)O(2)-induced apoptosis of rat cardiomyocytes independently of antioxidant enzyme expression. Rev Port Cardiol 2021;40:273-81. (Engl Ed). [Crossref] [PubMed]
- Li J, Chen Q, He X, et al. Dexmedetomidine attenuates lung apoptosis induced by renal ischemia-reperfusion injury through α(2)AR/PI3K/Akt pathway. J Transl Med 2018;16:78. [Crossref] [PubMed]
- Zheng L, Jia R, Zhao J. Retracted: Dexmedetomidine Regulates Proliferation, Apoptosis, Migration, and Invasion in Ovarian Cancer Cells via MiR-155-HIF-1α Axis. Med Sci Monit 2021;27:e931024. [Crossref] [PubMed]
- Gaertner J, Fusi-Schmidhauser T. Dexmedetomidine: a magic bullet on its way into palliative care-a narrative review and practice recommendations. Ann Palliat Med 2022;11:1491-504. [Crossref] [PubMed]
- Jankowski MP, Miller L, Koerber HR. Increased Expression of Transcription Factor SRY-box-Containing Gene 11 (Sox11) Enhances Neurite Growth by Regulating Neurotrophic Factor Responsiveness. Neuroscience 2018;382:93-104. [Crossref] [PubMed]
- Kim DK, Han SB, Hong ST, et al. Expression of Sox11 and Brn transcription factors during development and following transient forebrain ischemia in the rat. Neurosci Lett 2008;433:259-64. [Crossref] [PubMed]
- Wang M, Liang X, Cheng M, et al. Homocysteine enhances neural stem cell autophagy in in vivo and in vitro model of ischemic stroke. Cell Death Dis 2019;10:561. [Crossref] [PubMed]
- Dojo Soeandy C, Salmasi F, Latif M, et al. Endothelin-1-mediated cerebral ischemia in mice: early cellular events and the role of caspase-3. Apoptosis 2019;24:578-95. [Crossref] [PubMed]
- Chen Y, Fan Z, Wu Q. Dexmedetomidine improves oxygen-glucose deprivation/reoxygenation (OGD/R) -induced neurological injury through regulating SNHG11/miR-324-3p/VEGFA axis. Bioengineered 2021;12:4794-804. [Crossref] [PubMed]
- Wang Q, Tan Y, Zhang N, et al. Dexmedetomidine inhibits activation of the MAPK pathway and protects PC12 and NG108-15 cells from lidocaine-induced cytotoxicity at its maximum safe dose. Biomed Pharmacother 2017;91:162-6. [Crossref] [PubMed]
- Klingler E, Tomasello U, Prados J, et al. Temporal controls over inter-areal cortical projection neuron fate diversity. Nature 2021;599:453-7. [Crossref] [PubMed]
- Chang KC, Bian M, Xia X, et al. Posttranslational Modification of Sox11 Regulates RGC Survival and Axon Regeneration. eNeuro 2021; Epub ahead of print. [Crossref] [PubMed]
- Jankowski MP, Cornuet PK, McIlwrath S, et al. SRY-box containing gene 11 (Sox11) transcription factor is required for neuron survival and neurite growth. Neuroscience 2006;143:501-14. [Crossref] [PubMed]
- Zhou Z, Lu J, Liu WW, et al. Advances in stroke pharmacology. Pharmacol Ther 2018;191:23-42. [Crossref] [PubMed]
- Rabinstein AA. Update on Treatment of Acute Ischemic Stroke. Continuum (Minneap Minn) 2020;26:268-86. [Crossref] [PubMed]
- Zhang Y, Chen Q, Chen D, et al. SerpinA3N attenuates ischemic stroke injury by reducing apoptosis and neuroinflammation. CNS Neurosci Ther 2022;28:566-79. [Crossref] [PubMed]
- Chen B, Lin W, Qi W, et al. Cofilin Inhibition by Limk1 Reduces Rod Formation and Cell Apoptosis after Ischemic Stroke. Neuroscience 2020;444:64-75. [Crossref] [PubMed]
- Yan L, Zhu T. Effects of rosuvastatin on neuronal apoptosis in cerebral ischemic stroke rats via Sirt1/NF-kappa B signaling pathway. Eur Rev Med Pharmacol Sci 2019;23:5449-55. [PubMed]
- Tang H, Gamdzyk M, Huang L, et al. Delayed recanalization after MCAO ameliorates ischemic stroke by inhibiting apoptosis via HGF/c-Met/STAT3/Bcl-2 pathway in rats. Exp Neurol 2020;330:113359. [Crossref] [PubMed]
- Lai Y, Lin P, Chen M, et al. Restoration of L-OPA1 alleviates acute ischemic stroke injury in rats via inhibiting neuronal apoptosis and preserving mitochondrial function. Redox Biol 2020;34:101503. [Crossref] [PubMed]
- Li HQ, Xia SN, Xu SY, et al. γ-Glutamylcysteine Alleviates Ischemic Stroke-Induced Neuronal Apoptosis by Inhibiting ROS-Mediated Endoplasmic Reticulum Stress. Oxid Med Cell Longev 2021;2021:2961079. [Crossref] [PubMed]
- Li H, Zhang X, Chen M, et al. Dexmedetomidine inhibits inflammation in microglia cells under stimulation of LPS and ATP by c-Fos/NLRP3/caspase-1 cascades. EXCLI J 2018;17:302-11. [PubMed]
- Deng S, Dai G, Chen S, et al. Dexamethasone induces osteoblast apoptosis through ROS-PI3K/AKT/GSK3β signaling pathway. Biomed Pharmacother 2019;110:602-8. [Crossref] [PubMed]
- Xia M, Ji NN, Duan ML, et al. Dexmedetomidine regulate the malignancy of breast cancer cells by activating α2-adrenoceptor/ERK signaling pathway. Eur Rev Med Pharmacol Sci 2016;20:3500-6. [PubMed]
- Xu S, Gao R, Chen L. Dexmedetomidine regulates sevoflurane-induced neurotoxicity through the miR-330-3p/ULK1 axis. J Biochem Mol Toxicol 2021;35:e22919. [Crossref] [PubMed]
- Mohammadi L, Mosayyebi B, Imani M, et al. Correlation Between Dexamethasone and miRNAs in the Regulation of Apoptosis, Drug-resistance, and Metastasis of Cancer Cell. Curr Mol Med 2021;21:392-401. [Crossref] [PubMed]
- Peshdary V, Atlas E. Dexamethasone induced miR-155 up-regulation in differentiating 3T3-L1 preadipocytes does not affect adipogenesis. Sci Rep 2018;8:1264. [Crossref] [PubMed]
- Xing L, Zhang X, Feng H, et al. Silencing FOXO1 attenuates dexamethasone-induced apoptosis in osteoblastic MC3T3-E1 cells. Biochem Biophys Res Commun 2019;513:1019-26. [Crossref] [PubMed]
- Lin L, Lee VM, Wang Y, et al. Sox11 regulates survival and axonal growth of embryonic sensory neurons. Dev Dyn 2011;240:52-64. [Crossref] [PubMed]
- Tsang SM, Oliemuller E, Howard BA. Regulatory roles for SOX11 in development, stem cells and cancer. Semin Cancer Biol 2020;67:3-11. [Crossref] [PubMed]
- Fang M, Xu T, Fan S, et al. SOX11 and FAK participate in the stretch-induced mechanical injury to alveolar type 2 epithelial cells. Int J Mol Med 2021;47:361-73. [Crossref] [PubMed]
- von Wittgenstein J, Zheng F, Wittmann MT, et al. Sox11 is an Activity-Regulated Gene with Dentate-Gyrus-Specific Expression Upon General Neural Activation. Cereb Cortex 2020;30:3731-43. [Crossref] [PubMed]
(English Language Editor: A. Kassem)